Veterinary Anatomic and Clinical Pathology I Study Guide 2022 PDF
Document Details
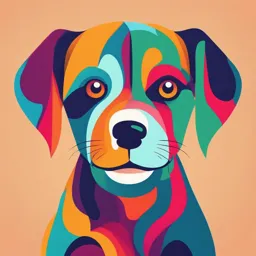
Uploaded by GlowingDada4940
Massey University
2022
null
Tags
Summary
This study guide for Veterinary Anatomic and Clinical Pathology I at Massey University covers the 2022 course content for weeks 1-6. It details postmortem examination techniques, sample collection procedures, and lesion description. The guide also includes sample preservation methods and submission protocols.
Full Transcript
227.313 Veterinary Anatomic and Clinical Pathology I Study Guide Weeks 1-6 2022 Pathobiology Department School of Veterinary Science Massey University SESSION 1 POSTMORTEM EXAMINATION References...
227.313 Veterinary Anatomic and Clinical Pathology I Study Guide Weeks 1-6 2022 Pathobiology Department School of Veterinary Science Massey University SESSION 1 POSTMORTEM EXAMINATION References King, John M; Roth-Johnson, Lois; Dodd, David C.; Newsom, Marion E.THE NECROPSY BOOK: A Guide for Veterinary Students, Residents, Clinicians, Pathologists, and Biological Researchers. Available online: http://hdl.handle.net/1813/37948 McDonough S, Southard T. The necropsy guide for dogs, cats, and small mammals. 1st ed. Wiley, Blackwell, 2017 Zachary J. Pathologic Basis of Veterinary Disease. 6th ed, 2017 Disclaimer: Content and images from these notes come from different sources (see references). These notes are intended for teaching purposes only. Please do not distribute. Section learning objectives Understand the purpose and the importance of conducting a postmortem examination in veterinary practice Learn the basic principles and techniques to perform a postmortem examination adequately and safely in domestic animal species Learn how to collect appropriate samples for subsequent diagnostic evaluation (histology, clinical pathology, microbiology, parasitology, toxicology) Learn the basics of lesion description and interpretation. This notes are intended to be used in conjunction with the “Postmortem examination” Kuracloud tutorial. “POSTMORTEM” = “PM” = “NECROPSY” = “AUTOPSY” What is it? A careful dissection, gross examination, and tissue collection from a body aimed at determining the cause of death or illness. Why do it? In clinical practice, a postmortem (PM) examination may be performed for various reasons. Examples include: To determine the cause of death and/or establish the cause of disease To confirm or clarify a clinical diagnosis, and to rule out other disease processes To assess the effectiveness of medical or surgical therapy To diagnose herd health management problems or diseases in order to establish causes of production loss For disease surveillance. Who does it? In some cases, the autopsy will be performed by the veterinarian involved, while in other cases either the carcass or individual tissue samples will be referred to a diagnostic laboratory for gross and/or histological examination. As always, a thorough and accurate history is essential for a meaningful diagnosis to be made. Which Samples? In some cases, the gross lesions are sufficiently distinct in their pattern to be presumptively diagnostic based on autopsy alone. However, when a definitive diagnosis is not obvious from the gross examination, samples can be collected and submitted to a diagnostic laboratory for histopathology or/and additional testing. In this case, decisions need to be made about which tissues to collect and to send. Some recommendations can be followed: If there are observable gross lesions present, (e.g., a mass in the liver, or ulcers in the stomach wall), take samples of organs with obvious lesions In cases where gross abnormalities are not observed, a range of tissues can be collected including representative samples of lung, liver, kidney, and heart muscle Other tissues may be included depending on the clinical signs and the medical history (e.g. obviously it would be appropriate to include samples of the gastrointestinal tract if diarrhoea was a feature of the disease). In some diseases, specific tissues are required to make a diagnosis (for example samples of the gastrointestinal tract in cases of enteric disease; brain tissue in cases of suspected neurological disease) It is worth noting that most diagnostic laboratories charge a set amount for 3 or more tissues, so when in doubt, send as many tissues as possible. Sample Preservation Autopsies should be performed as soon as possible after death. If a delay is inevitable, it is better to chill a carcass than to freeze it. Freezing and thawing cause tissue damage that can make histological interpretation difficult or impossible. In contrast, chilling will slow the rate of autolysis, without causing significant damage to tissues. Fresh tissues must be preserved before they can be processed. Tissue decomposition (autolysis) starts almost immediately after death, so sample preservation should not be delayed. Phosphate-buffered 10% formalin solution is the standard fixative in diagnostic laboratories and is suitable for most tissues. Formalin is a hazardous substance that gives off an irritant gas, affecting the eyes and nose. Safety regulations should be strictly adhered to when preparing solutions (protective masks or fume hoods), as well as when handling and processing samples in order to both protect yourself and to prevent exposure of couriers and laboratory staff to irritant fumes (e.g. use of leak proof containers, protective packaging). Some practical information: Proper tissue fixation is essential for obtaining diagnostically useful sections for microscopic evaluation. Adequate tissue preservation requires formalin to penetrate throughout the entire sample Sections must be 0.5 cm thick to fix properly. Big pieces may autolyze before they fix! Fixative to tissue ratio must be at least 10:1 Because tissues harden once they are fixed, don’t squeeze tissues into jars with narrow necks – it may be impossible to get them out again once they are fixed! Samples for procedures other than histology can also be collected during autopsies. Examples include faeces for parasite egg counts, tissues and tissue contents for toxicological analysis, or collection of fine needle aspirates or smears for cytology. Sample Submission Sample containers should be labelled with the practice name, owner’s name, animal identification, and date. Submission forms should be filled out fully and clearly. Details required usually include: Practice name, address and veterinarian’s name Owner’s name, animal identification and signalment (species, breed, age and sex) Date Clinical history (may include duration of illness, response to treatment, previous lab results) Nature and site of sample (e.g. liver biopsy, fine needle aspirate of a mass - diagrams may be useful) Tests required. Packaging The aim of packaging is to ensure that samples reach the laboratory intact. Measures should be taken to ensure that lids do not leak, containers do not break, paperwork remains dry and the correct forms are with each sample. Most commercial laboratories supply sample containers and plastic bags. If you use your own containers, these should be sturdy, have screw tops, be of adequate size, and be well labelled. General Rules When taking and submitting samples for histopathology, follow the following general principles: Remember the 1:10 rule! ONE PART TISSUE TO TEN PARTS FORMALIN SOLUTION Be gentle, minimise handling DO NOT scrape the luminal surface (mucosa) of luminal organs Put fresh tissue into formalin as quickly as possible Small pieces fix more rapidly than large pieces; make sure samples are representative of the disease process If large samples need to be submitted (e.g. whole brain, spleen) consider that they will need more formalin (and a larger container) and will take longer to fix Multiple samples of different organs from the same animal can generally be put into the same container (with some exceptions like skin biopsies) Label everything fully, complete submission forms (including a detailed clinical history), and package securely (screw tops are more leak proof than other types of lids, plastic is safer than glass). Documenting gross lesions seen at postmortem examination The final diagnosis obtained from a postmortem examination requires the integration of data from a variety of sources. The postmortem examination is just part of the picture; history, clinical findings, laboratory data, histology are all equally important. When doing a field autopsy, followed by submission of tissues to the laboratory for a pathologist to examine, the quality and accuracy of the diagnosis is dependent in a large part on the information that you send. First and foremost, a complete clinical history is essential; include full signalment (species, age, sex, breed, colour, ID), and a concise medical history, clinical examination findings, and clinical pathology data if available. In production animal postmortem examinations, include information on herd management practices (number of animals affected, vaccination, drenching, production records, nutrition, water supply, use of pesticides/herbicides/fertilisers, presence/absence of poisonous plants) as this information may be relevant in the investigation. In field autopsies, the pathologist will rely in your descriptions! So adequate description of gross lesions is essential for lesion interpretation and disease diagnosis. It is also very helpful to photograph lesions and include them with your case submission. Morphological descriptions and diagnoses will be covered in the general pathology and inflammation sections. Photographing lesions Images of lesions, together with lesion descriptions, will assist the pathologist in interpreting changes seen histologically, and enable them to integrate findings and make a diagnosis. With the widespread availability of digital cameras/phone cameras it is relatively easy to snap an image of lesions and include it with the case submission. To make the most out of your photographs, make sure you follow these recommendations: 1. Make sure you have good light in the area you are taking the picture to ensure the image is clear and in focus 2. After taking the photograph, make sure that there is no glare over the lesion 3. Make sure the lesion is in the centre of the photo. Don’t make the viewer guess where the lesion is on the image! 4. Include a scale in photo if you can, a ruler is ideal but not always available in which case use an item that you know the size of, for example, a needle, knife, coin, etc. Autopsy Technique There are many ways to perform an autopsy, but any technique should be: Thorough: a quick autopsy may give the diagnosis in 50% of cases, but will consistently miss important lesions. “We miss more by not looking than by not knowing!” Systematic: develop the habit of performing each autopsy in the same way, so the technique becomes “automatic” Efficient: an efficient technique allows you to do the procedure quickly, while being thorough and systematic For more detailed information on the autopsy technique please refer to Chapter 3 “The Necropsy Procedure” (Pages 21- 37) from McDonough S, Southard T. The necropsy guide for dogs, cats, and small mammals. 1st ed. Wiley, Blackwell, 2017 (available through Massey Library). 227.313/W Roe/General Path/2022 Topic 1: General Pathology & Pathogenesis SPECIFIC LEARNING OBJECTIVES By the end of this topic you should be able to: - define the terms that are written in bold - describe how the various branches of pathology contribute to making a diagnosis - use your existing knowledge to construct a pathogenesis diagram for a common disease - list the changes that occur in a body after death - understand how post mortem changes develop - recognise common post mortem changes and understand how to distinguish them from lesions that developed during life INTRODUCTION Pathology is the study of disease, and acts as a bridge between fundamental science topics (anatomy, physiology) and medicine. If you understand the principles of pathology you will be well equipped to recognise, diagnose and treat diseases. There is a range of tools available to the clinician or pathologist when diagnosing diseases. Most of these tools aim at recognising changes in morphology (structure or appearance) or function. Gross lesions are defined as visible abnormalities in a tissue or organ that can be recognised with the naked eye. These lesions can be detected during a clinical exam in the live patient, or at post mortem examination in dead patients. In some cases the lesions are so distinctive that they can only have one possible cause – these are known as pathognomonic lesions. More often however, the appearance of a lesion gives us a clue as to the cause of a disease by allowing us to make a list of possible diagnoses (a differential diagnosis list). Many diseases also cause changes in the cellular structure and architecture of the affected tissue, which can often be seen under the microscope. These changes are known as histological lesions. In some cases tissues that appear normal grossly will have histological lesions, but in most cases these changes can be seen both grossly and histologically. Histopathology is a branch of pathology where tissues are collected surgically (biopsies) or at post mortem, fixed in a preservative, and processed into thin slices, stained and mounted on glass slides for examination under a microscope. As with gross lesions, histological lesions can be interpreted to give either a list of possible diagnoses, or a specific single diagnosis. The histological changes may give extra clues as to the cause of the disease (for example, finding gram negative bacteria in an area of pulmonary abscessation). 1 227.313/W Roe/General Path/2022 Cytology is another technique that uses microscopy to examine samples, but in this case the samples are not fixed sections of whole tissues or organs, but instead are collections of cells that are smeared or blotted onto a microscope slide before examination. In cytology we use cellular detail and cell type to make a diagnosis. Cytological techniques such as fine needle aspirates and skin scrapings are quick, cheap and fairly non-invasive compared with biopsies, and can often be examined in the clinic, rather than requiring submission to a diagnostic lab as for histopathology. Often the two methods are complementary, each giving different but useful information about the nature or cause of a disease. The ultimate purpose of the techniques described above is to make a diagnosis and to determine the cause of a disease. The term aetiology is used to describe the cause of a disease, and is not always the same as the diagnosis. For example, a doctor might tell you that your biopsy report came back with a diagnosis of dermatitis, but there are many different causes of dermatitis – the aetiology could be allergic, bacterial, fungal etc. Determining the aetiology of a disease can often involve additional tests such as microbiological culture, immunohistochemistry or molecular tests such as PCR (polymerase chain reaction). Not all diseases result in observable morphological changes in tissues or organs. Often there are changes in function of tissues/organs that may not be visible but can cause detectable changes in body fluids such as blood or urine. Two of the branches of pathology that investigate these changes are haematology (examination of whole blood) and clinical biochemistry (assays of enzyme levels in body fluids including plasma, serum and urine). DEVELOPMENT OF DISEASE (PATHOGENESIS) At its most basic level, disease results from damage to individual cells or to the membranes, enzyme systems and organelles that make up individual cells. This damage can be caused by physical trauma (e.g. rupture of cell membranes by crushing injury), membrane damage by oxidation, lack of oxygen supply, genetic defects, toxins, or infectious agents. When a group of cells are damaged, a gross or histological lesion can result. In some tissues, damage to cells causes them to leak out substances that accumulate and can be measured in serum or plasma (clinical biochemistry). General pathology is the study of the mechanisms by which disease occurs. One of the most important concepts of pathology is that of pathogenesis, which is the sequence of events that occurs to result in a lesion or a disease. You can think of this as a series of steps leading from the aetiology to the disease itself. If you understand the pathogenesis of a disease, you can often work out the most appropriate diagnostic tests, the likely gross and histological lesions, and sometimes gain an insight into how the disease could be treated. DEATH AND DECAY Somatic death is defined as “the disappearance of life from an entire individual”. The specific time of death can be difficult to determine, but generally we assume this happens when cardiac and respiratory function no longer occur spontaneously. Obviously not all cells will be dead at this point in time, but 2 227.313/W Roe/General Path/2022 eventually they will all die due to lack of oxygen (anoxia). Some tissues are more resistant to anoxia than others, and may survive for slightly longer. In human organ donors, death is defined as the time at which there is no spontaneous heartbeat or breathing, and there is no detectable brain activity. Body organs are still viable at this stage. Post mortem changes As soon as somatic death occurs, a number of predictable changes start to develop in the dead body. A thorough knowledge of these changes is extremely useful for forensic scientists, for example to determine time of death. Some post mortem changes result in visible abnormalities in tissues and organs that mimic real lesions: a good understanding of these changes is essential to prevent misinterpretation of these ‘pseudo-lesions’ at post mortem. 1. Cooling Dead bodies cool until they reach ambient temperature. The rate at which a body cools depends on: (i) the initial temperature of the body (ii) insulation (fat, wool, housing) (iii) external temperature and wind The core temperature of a dead body can be used to calculate a likely range for the time of death, as long as this is interpreted along with the factors above. 2. Rigor mortis Rigor mortis is the stiffening of muscles that occurs after death, and is due to the gradual contraction of muscle fibres that occurs as their energy supply (ATP) runs out. Uncoupling of actin-myosin complexes in muscle is an energy-requiring process, so once ATP runs out, muscles can no longer relax and sustained contraction of fibres begins to occur. For a short time (1-9 hours) after death ATP can be produced from glycogen, so rigor mortis does not begin immediately. The onset of rigor varies with glycogen stores (develops later in well-nourished individuals) and temperature (ATP is used up more quickly at higher temperatures). Rigor occurs in a defined order (head, heart, diaphragm then limbs) and disappears in the same order 12-30 hours later due to autolysis of muscle fibres. The degree of rigor can be used to estimate time of death within fairly narrow limits, taking into account environmental factors. 3. Blood changes a) Hypostatic congestion (lividity; livor mortis) After death, blood tends to accumulate in the more dependent parts of the body under the effect of gravity. For example, the lung that was downwards at the time of death will be more congested. After a while this pooled blood clots, and will remain in place even when the body is moved. b) Post mortem clots When the heart stops beating, blood stops moving and starts to clot. In the heart and large blood vessels, red cells have time to sediment out as the blood clots, so that a pale clot of serum forms over a 3 227.313/W Roe/General Path/2022 layer of red clot containing most of the red blood cells. The pale part of this type of clot is sometimes called a “chicken fat clot”. It is important to be able to distinguish between ante-mortem clots (known as thrombi) and post mortem clots. In general a post-mortem clot fills a vessel, is smooth and glistening, and conforms to the vessel shape. Thrombi form in a living body, are usually rough and granular, don’t separate into red and white layers, and are often attached to the wall of the blood vessel (see thrombosis lecture later). 4. Post-mortem degeneration Post-mortem degeneration (decay) of a body and its organs involves two processes that differ in origin but occur simultaneously: (i) Autolysis – breakdown of cells and tissues by the body’s own cellular enzymes. (ii) Putrefaction - breakdown of cells and tissues by bacteria that invade from the environment or from within the body (e.g. gut flora). The rate of post-mortem degeneration is influenced by: (i) Temperature Enzymes are more active in warm conditions (hence decay of a carcase or tissue can be delayed by refrigeration or freezing). (ii) The presence of bacterial species capable of invading and destroying tissue. (Note that neonatal animals are slower to putrefy because they have not acquired an intestinal flora.) Autolysis and putrefaction after death result in discolouration of tissues, production of gas and breakdown of tissue structure. The ‘pseudo-lesions’ that result from these changes include: a) Paleness and friability Autolysis results in breakdown of tissue fibres and cells, causing loss of colour and tissue fragility (friability). In some organs this can mimic disease processes (e.g. fatty liver – see later). b) Pseudonecrosis This term describes areas of discolouration and softening within organs that mimics the appearance of groups of dead (necrotic) cells. Necrosis is discussed in more detail in a later lecture. c) Pseudomelanosis This is dark staining of tissues that occurs due to production of iron sulphide. Iron sulphide forms from interactions between hydrogen sulphide (produced by invading anaerobic bacteria) and iron (from red blood cells) d) Gas production Gas is produced by anaerobic putrefactive bacteria, and can accumulate in body cavities (mimicking bloat) or as small bubbles in tissues (post mortem emphysema). Accumulation of large amounts of putrefactive gases in the abdominal viscera can cause stomach or intestinal rupture. (Think about how you might distinguish between ante mortem and post mortem gut rupture.) Putrefactive gases are often foul-smelling (hydrogen sulphide). e) Haemoglobin imbibition This term describes staining of tissues with blood pigments, and can be misinterpreted as bruising or haemorrhage. 4 227.313/W Roe/General Path/2022 f) Bile imbibition Tissues adjacent to the gall bladder or bile ducts can be stained dark orange to greenish after death. Tutorial 1 – Understanding pathogenesis LEARNING OBJECTIVES - To integrate knowledge of anatomy, physiology, medicine and pathology in order to work out the pathogenesis of selected diseases - To apply your knowledge of pathogenesis in order to determine how to make a diagnosis for selected diseases The pathogenesis of a disease is essentially the steps that occur between a cause (aetiology) and the outcomes of the condition, including clinical signs, gross lesions and histological lesions. Understanding pathogenesis is essential to be able to make rational, informed decisions about diagnosis and treatment. In class we will use an example disease to illustrate how you can use your understanding of pathogenesis to work out the consequences of the disease and potentially how to treat it. 5 227.313/W Roe/General Path/2022 Topic 2: Cellular Degeneration SPECIFIC LEARNING OBJECTIVES: By the end of this topic you should be able to: - define the terms that are written in bold - explain the differences between reversible and irreversible cell injury - recognise and distinguish between cellular changes that indicate cell degeneration and cell death - explain the pathogenesis of reversible and irreversible cell injury CELLULAR DAMAGE /DEATH WITHIN THE LIVING BODY All forms of cell damage act by damaging cell membranes or enzyme systems. If the initial injury is mild or is corrected quickly then the cell may be able to recover (reversible cell injury). On the other hand, if the damage is severe or prolonged then irreversible injury (cell death) will occur. Both reversible and irreversible cellular damage cause changes in the cell that can be recognised under a light microscope. In addition, damaged cell membranes can allow intracellular substances to leak out into surrounding tissue fluids. Some of these substances can build up in blood or urine and be measured as part of diagnostic clinical biochemistry. (What would you need to know to be able to interpret these levels?) Reversible cell injury (cellular degeneration) In almost all cases, cell degeneration begins with damage to the sodium-potassium pump in the cell membrane. The function of this pump is to maintain cellular homeostasis by removing excess intracellular sodium, in exchange for potassium. The pump requires energy in the form of ATP, and if anything causes loss of ATP (e.g. lack of oxygen) or damage to the pump itself, then intracellular sodium levels will slowly increase. This is followed by movement of water from the extracellular fluid into the cell, across an osmotic gradient (cell swelling). Cell swelling may become severe enough to detach ribosomes from the endoplasmic reticulum, impairing protein production and thus compromising cell function even further. These changes are potentially reversible, but if the initiating cause of damage is severe or repeated, irreversible damage (cell death) may occur. Irreversible injury (cell death) Irreversible injury occurs when cell swelling becomes so severe that mitochondria are damaged (preventing energy production) and membranes rupture, including both the cell membrane and membranes of cell organelles (lysosomes, nuclei etc.). Membrane damage can become self- perpetuating, through: (i) influx of calcium into the cell across damaged membranes, resulting in activation of endogenous phospholipases, which in turn lyse membrane phospholipids 6 227.313/W Roe/General Path/2022 (ii) cytoskeletal abnormalities caused by cell swelling, including detachment of the cell membrane from the cytoskeleton (iii) production of free radicals (particularly following reperfusion of blood to damaged tissues) (iv) production of lipid breakdown products due to phospholipase activity, which have a detergent effect on cell membranes Fig 1. Mechanisms of membrane damage in cell injury. Decreased O2 and increased cytosolic Ca2+ are typically seen in ischemia (lack of blood supply) but may accompany other forms of cell injury. Reactive oxygen species, which are often produced on reperfusion of ischemic tissues, also cause membrane damage (not shown). Causes of cell damage 1. Hypoxia Hypoxia means lack of oxygen supply. It mediates many types of cell damage, by causing decreased ATP production and therefore decreased function of the Na/K pump. The following diagram summarises the mechanisms involved in hypoxic cell damage. 7 227.313/W Roe/General Path/2022 Fig 2. Functional and morphologic consequences of decreased intracellular ATP during hypoxic cell injury. (List the mechanisms that could result in decreased oxygen delivery to a tissue.) 8 227.313/W Roe/General Path/2022 2. Other causes of cell damage There are numerous other causes of cell damage, including direct trauma, heat, infectious agents and chemical agents. Many of these are mediated by hypoxia or by ‘free radical damage’. Free radicals are unstable chemicals that can damage cell proteins, DNA, mitochondria and cell membranes. Note: One of the online modules explores a particular consequence of free radical damage known as lipid peroxidation of cell membranes. This occurs because the double bonds present between carbon atoms in unsaturated fatty acids (e.g. in cell membranes) are vulnerable to attack by free radicals. Oxidation of these fatty acids results in production of lipid peroxides, which are themselves free radicals, propagating a chain reaction and causing more and more membrane damage. Some fats, such as fish oils, are highly unsaturated and are therefore particularly prone to free radical damage and lipid peroxidation. (iii) breaks in DNA strands (this can cause both cell death, and malignant transformation of cells) (iv) mitochondrial damage (causing decreased ATP production) Free radicals are formed during normal cellular metabolism in healthy individuals, and the body has inbuilt mechanisms to decrease damage from these free radicals, including: (i) natural decay (e.g. superoxide radicals decay to form O2 and H2 O.) (ii) anti-oxidants, which can either block production of free radicals or inactivate them as soon as they are formed. Examples include vitamin E (“scavenges” free radicals) and transferrin (binds free iron, thus blocking catalysis of hydroxyl ion production) (iii) enzymes - e.g. superoxide dismutase; glutathione peroxidase (a selenium-containing enzyme). Morphological appearance of cellular degeneration Cell swelling and eosinophilia Cell membrane damage leads to cell swelling as extracellular fluid moves into the affected cell. Increased intracellular fluid results in disruption of the cytoskeleton as well as swelling of individual organelles, compromising normal cell function. The most common routine stain for histological sections is haematoxylin and eosin (H&E). The haematoxylin component stains basic structures such as nucleic acids blue, while the eosin stains proteins pink. In H&E stained sections from tissues with cell swelling, the affected cells appear enlarged and cloudy with slightly granular cytoplasm. This change is sometimes known as hydropic degeneration. Cell swelling is most commonly seen in hepatocytes, renal tubular cells, and cardiac muscle. Although cell swelling is reversible, the increase in cell size can have other consequences - for example swelling of hepatocytes may cause compression of sinusoids and decreased blood, thus exacerbating the initial liver injury. 9 227.313/W Roe/General Path/2022 In H&E sections, degenerate cells also appear pinker than normal. As a cell and its organelles swell, the ribosomes become detached from the endoplasmic reticulum and disperse throughout the cytoplasm. Since ribosomes contain RNA, which stains blue with haematoxylin, the loss of blue staining causes a relative increase in pinkness (eosinophilia). 10 227.313/W Roe/General Path/2022 Topic 3: Necrosis (cell death) SPECIFIC LEARNING OBJECTIVES By the end of this topic you should be able to: - define the terms that are written in bold - recognise and give examples of the main types of necrosis - describe how necrotic material is removed or otherwise ‘dealt with’ by the body, and why this is important - describe the pathogenesis and types of gangrene - discuss the potential sequelae of gangrene, including how they develop and how they can be mitigated Definition Irreversible cell damage leads to cellular necrosis, which is defined as the local death of tissue cells within a living individual. Cell death may occur suddenly or slowly, with a cell passing through various stages of "degeneration" through a point of no return until finally it dies. Significance of necrosis The clinical effect of necrosis on an animal (or person) depends on the site, size, rate of progression, and sequelae. A small focus of necrosis in the brainstem or within the myocardial conduction system is likely to quickly lead to death. Conversely, even a large focus of necrosis in an organ with abundant reserves may have no clinical effect. Rapid loss of tissue is always more significant than gradual loss. There are also many examples where the inflammatory or healing sequelae (consequences) of necrosis are more significant than the original necrosis. Necrosis of the oesophagus heals by scarring and stricture of the lumen results. Local reaction of the body to necrosis Necrotic tissue is irritant, and initiates a mild local inflammatory reaction. Microscopically, this appears as an accumulation of leucocytes (inflammatory cells) surrounding and sometimes infiltrating the area of necrosis, as well as a rim of dilated blood vessels (hyperaemia). Sometimes this peripheral inflammatory zone can be seen grossly as a red line of demarcation between living and necrotic tissue. Systemic reaction to necrosis Enzymes released from damaged and dying cells can leak out into the serum. This can be useful diagnostically, but some of the enzymes that are released are mediators of inflammation, causing an acute phase response. This response can cause quite severe systemic illness, including fever, lethargy, 11 227.313/W Roe/General Path/2022 depression and anorexia (for example, a cat with a cat bite abscess will often be quite ill until the abscess is treated (or bursts), removing the source of enzymes that cause the acute phase response). Morphologic appearance of necrosis Grossly, necrotic tissue tends to be pale and friable, often with a rim of hyperaemia. Necrosis can resemble other changes such as autolysis and fatty infiltration. Under the microscope there are several diagnostically useful changes that can be used to distinguish between necrosis and cellular degeneration: 1. Cytoplasmic changes Necrotic cells show increased eosinophilia, as for cellular degeneration, but tend to be smaller than normal (as opposed to swollen). A distinctive feature of necrosis is loss of the cell membrane, which often appears histologically as a lack of distinct borders between adjacent cells. 2. Nuclear changes Nuclear changes are more reliable histologic indicators of necrosis than the changes that occur in the cytoplasm. These are: (i) Pyknosis: a shrunken dark-staining (ii) Karyorrhexis: fragmentation of the nucleus (iii) Karyolysis: ‘fading’ of the nucleus as it loses affinity for haematoxylin Classification of necrosis Certain types of necrosis are distinctive grossly or histologically, and are suggestive of specific aetiologies. It is important to remember that pathological lesions are dynamic, and the appearance of necrotic tissue can change over time. For example an area of coagulative necrosis can be invaded by bacteria and turn into suppurative necrosis. It is also important to note that morphologic changes in tissues often lag behind the biochemical events that have already determined the fate of the cell. Myocardial cells irreversibly injured by an ischaemic event, for example, may look grossly and histologically normal for several hours after they are functionally dead. 1. Coagulative necrosis is local death of tissue within the living individual in which the architectural detail of the tissue persists, though cellular detail is lost, i.e. we can recognise the tissue as, for example, liver even though the individual cells are dead. The most common causes of coagulative necrosis are ischaemia, burns and caustic chemicals. 2. Caseous necrosis is the local death of tissue within a living individual, in which both cellular and architectural detail of the tissue is lost. Affected areas look like a homogenous pale, granular mass. Causes include certain specific bacterial infections such as Mycobacterium tuberculosis and Corynebacterium pseudotuberculosis. 3. Liquefactive necrosis is characterised by the disintegration of the necrotic material into a liquid mass, in which all cellular and architectural detail is lost. This type of necrosis is most often seen in the central nervous system, where there are large numbers of enzymes and abundant lipid-containing material that 12 227.313/W Roe/General Path/2022 tends to break down into a clear, soft-to liquid material. Liquefactive necrosis of the CNS is known as malacia (eg. polioencephalomalacia). 4. Suppurative necrosis Suppuration is a combination of liquefaction and pus formation. Pus, or purulent exudate, is composed of semi-liquid necrotic debris and neutrophils suspended in tissue fluid. Suppuration involves particular types of bacteria known as pyogenic (pus-forming) bacteria, which are able to attract large numbers of neutrophils from the blood. Liquefaction of the infected tissue occurs due to lytic enzymes released from the bacteria themselves as well as from dead tissue cells and dying neutrophils. 5. Fat necrosis is the death of fat cells. The process involves the splitting of stored fat into glycerol and fatty acids. The fatty acids combine with calcium, potassium or sodium salts to form soaps which precipitate and appear microscopically as a dull opaque, slightly basophilic material, contrasting with the clear spaces in normal fat cells. This process is known as saponification. Grossly, necrotic fat appears opaque, firm and white, and can cause a significant inflammatory response. In some cases peroxidation of lipids results in deposition of a yellow pigment known as ceroid (e.g. ‘yellow fat disease’ in cats, mink and ferrets. Note: this forms the basis of online tutorial Case 3.) Sequelae of necrosis Ideally, the body needs to either dispose of or wall off areas of necrosis, in order to minimise ongoing inflammation and to decrease or stop the acute phase response. The fate of necrotic tissue depends on: - the type of necrosis - the tissue involved - the amount of tissue involved Sometimes necrotic tissue may remain in situ for a considerable time. Ultimately its disposal is by one of the following processes: 1. Liquefaction and removal This mechanism of dead tissue removal tends to occur when the amount of affected tissue is small, and the tissue is rich in enzymes and tissue fluid. Once liquefied, the resulting fluid and small molecules can be absorbed into the blood or lymphatics. 2. Encapsulation without liquefaction (sequestration). This occurs with ‘dry’ types of necrosis such as coagulative necrosis. The dead tissue acts as an irritant and incites a low-grade inflammatory reaction, which leads to the development of new fibrous tissue as part of the repair process. This ultimately results in a fibrous capsule surrounding a necrotic centre, known as a sequestrum. With time the necrotic centre gets more and more inspissated (dry) and may gradually be replaced by fibrous tissue as its contents are very slowly reabsorbed. The most common examples of sequestrum formation in clinical practice are corneal sequestra (an idiopathic condition seen mostly in long-haired cat breeds) and bony sequestra. 13 227.313/W Roe/General Path/2022 3. Abscessation The formation of pus within a tissue results in an abscess. As the amount of pus increases, so does the internal pressure. This leads to pain, redness and swelling. Abscesses can resolve either by formation of a thick fibrous capsule around the pus (similar to sequestration), or by bursting, where the necrotic purulent material dissects its way out through the surrounding tissue onto the surface or lumen of the tissue involved. 4. Erosion and ulceration. On the body surface (e.g. skin, bladder, ureter, bronchus, intestine) dead cells may lose their attachment to the underlying living tissue and desquamate (or slough) into the surrounding space or lumen. This usually occurs in the skin and luminal organs, causing either ulceration or erosion. Erosion implies loss of epithelial cells only, while ulceration occurs when the basement membrane is involved and underlying connective tissue is exposed. 5. Repair and regeneration These processes are dealt with in your inflammation lectures. GANGRENE Definition. Gangrene is a term borrowed from human medicine, where it means ischaemic necrosis of extremities. Classification 1. Dry gangrene This is uncomplicated ischaemic necrosis. A good example of dry gangrene would be frostbite, where extreme vasoconstriction causes obliteration of the blood supply. 2. Moist gangrene Where ischaemic necrosis becomes complicated by bacterial infection, moist gangrene can occur. In these cases, the affected tissue is dark and foul smelling. Necrotic tissues accessible to organisms, e.g. leg, udder, tail or gut, are more often affected with gangrene than less accessible organs such as bone, muscle and heart. Bacteria are able to invade because the normal body defence mechanisms are inactive in dead tissue. 3. Gas gangrene This is a special form of moist gangrene caused by gas producing bacteria of the genus Clostridia. These organisms are anaerobic spore-forming saprophytes, usually found in the intestinal tract or ground. They can’t grow in healthy tissue, but can establish themselves in small areas of necrosis or bruising where there is low oxygen content. Once established they proliferate and produce necrotising 14 227.313/W Roe/General Path/2022 exotoxins that diffuse into the nearby healthy tissue and induce necrosis. The organisms are than able to colonise this new area of necrosis. Thus invasion of the tissues by Clostridia occurs behind a widening front of exotoxin-induced necrosis. Bubbles of gas formed by the organisms are found in the gangrenous tissue and give it a characteristic foul odour. Morphological appearance of gangrene. Because gangrenous tissue is dead, it is cool to the touch, unresponsive to stimuli, and does not bleed on incision. The dark colour is due to: (i) anoxic blood (dark bluish/red) (ii) haemosiderin (golden brown) and other pigments associated with breakdown of red blood cells (iii) iron sulphide (dark green) derived from breakdown of –SH containing amino acids, producing hydrogen sulphide which then reacts with iron from red cells to form iron sulphide. Hydrogen sulphide is partly responsible for the foul odour of gangrenous tissue in moist gangrene. Areas of necrosis and gangrene become separated from the adjoining living tissue by an inflammatory reaction, which forms a visible line of demarcation and is readily observed microscopically by the invasion of the zone by leucocytes. Sequelae of gangrene Gangrene is a serious event. Toxic substances produced during the decomposition of body proteins within the gangrenous tissue can be absorbed into the lymphatics and bloodstream of adjacent living areas causing toxaemia, i.e. toxins in the blood. This is one of the reasons why surgical debridement (removal of dead tissue) or amputation are often used in gangrene treatment. If gangrene is untreated, the following sequelae are possible: - sloughing of the dead tissue, followed by scarring (if the animal survives) - death from toxaemia. 15 227.313/W Roe/General Path/2022 Topic 4: Cellular Adaptations (Disturbances of Growth) SPECIFIC LEARNING OBJECTIVES At the end of this lecture you should be able to: - provide definitions for the terms written in bold - understand the similarities and differences between the different types of cellular adaptation - give examples of disease processes and physiological processes that involve each cellular adaptation - demonstrate your understanding of how cellular adaptations can cause disease or abnormal function - determine how to make a diagnosis in cases of dysfunction or disease associated with cellular adaptations INTRODUCTION All cells require the ability to adapt to environmental conditions. Even in a healthy animal cells may need to adjust their activities, for example in response to different kinds of hormonal stimulation. When such changes occur in a healthy animal under normal conditions they are known as physiological adaptations. In injury or disease, cellular changes may take place in order to enable a cell or tissue to survive; these are known as pathological adaptations. TYPES OF GROWTH DISTURBANCE/CELLULAR ADAPTATION Atrophy refers to an organ or tissue that initially developed to normal size but then shrank due to a decrease in size of the individual cells making up the tissue (Compare atrophy with hypoplasia.) Examples include: - disuse atrophy (e.g. muscles) - denervation atrophy (e.g. following spinal injury) - loss of hormonal stimulation (e.g. atrophy of the prostate following castration) Hypertrophy is the increase in the size of a tissue or organ due to an increase in the size of individual cells. This term can apply to a whole organ or to certain tissues within an organ. For example, a thickened intestinal wall can be due to hypertrophy of the smooth muscle layers, with a normal mucosa. Hypertrophy may be pathological (a response to disease) or physiological (a response to increased metabolic or physiological demand). Note that hypertrophy is the only way organs made up of ‘permanent’ or post-mitotic cells can increase in size, as these cells can’t divide (e.g. cardiac muscle). Examples of hypertrophy include: - increased skeletal muscle bulk due to prolonged exercise (physiological hypertrophy) 16 227.313/W Roe/General Path/2022 - increased ventricle wall thickness due to a stenotic valve (pathological hypertrophy) - physiological hypertrophy of the uterus in pregnancy due to stretching and enlargement of smooth muscle cells Hypoplasia is the incomplete growth of an organ or tissue. This can vary from severe (only tiny remnants of tissue) to mild (a very slight decrease in size with relatively normal function). Aplasia or agenesis is the extreme form of hypoplasia and means the complete absence of a tissue. Atresia is a term used for the absence of a lumen. - testicular hypoplasia - cerebellar and cerebral hypoplasia - aplasia of the palate leading to cleft palate - intestinal atresia - aplasia of the epididymis Note that hypoplasia, aplasia/agenesis, and atresia are congenital rather than being true cellular adaptations. An acquired decrease in luminal size of an organ that was previously normal is known as a stricture or stenosis (e.g. blockage of the oesophagus due to scarring; narrowing of an artery due to atherosclerosis). Hyperplasia is an increase in the number of cells in a tissue or organ, which may increase the size of the organ grossly. As with hypertrophy, a specific population of cells within an organ may undergo hyperplasia. The stimuli for hyperplasia can be the same as for hypertrophy but is obviously limited to cell types that can divide. Not surprisingly, enlargement of an organ can be due to both hyperplasia and hypertrophy – the distinction between the two can be impossible to make grossly, and may need to be confirmed histologically. Examples include: - thyroid and parathyroid hyperplasia - adrenocortical hyperplasia - prostatic hyperplasia Hyperplastic lesions are often further described by an adjective e.g cystic hyperplasia of the gall bladder; nodular hyperplasia of the spleen. Variations between species and between individuals mean that it can sometimes be difficult to determine when an organ or tissue is abnormal in size, and you may need to know: - the normal range of organ size/weight in the species - normal ratios (e.g.cortex to medulla thickness ratio for adrenal glands; ventricle wall thickness ratios in suspected heart disease; the ratio of thyroid weight to bodyweight for diagnosing goitre in neonates) - for paired organs, the size of the other organ (eg unilateral testicular hypoplasia) Dysplasia is a term that describes a lack of normal histologic architecture in a tissue or organ. Dysplasia can be developmental e.g. hip dysplasia (an abnormally shaped hip joint). The term can also be used in 17 227.313/W Roe/General Path/2022 the description of a neoplasm (see later) or in any situation where there is jumbled differentiation of a tissue e.g. healing epithelium will often be disorganised due to the presence of damaged remnants of underlying connective tissue. Note: the term dystrophy is very similar in meaning to dysplasia, but in human medicine this term has come to be associated with a few specific conditions involving abnormal tissue structure, namely, fibrous osteodystrophy (abnormal bone architecture occurring due to a nutritional imbalance) and muscular dystrophy, a group of inherited diseases of muscle function. Metaplasia is where one fully differentiated cell type changes to a different but related cell type. The classic example is squamous metaplasia in which cuboidal or columnar epithelium changes to stratified squamous epithelium. A metaplastic change usually involves a change to a cell type that is more durable in response to chronic irritation, for example squamous metaplasia of the bronchiolar epithelium in response to chronic irritation caused by smoking. Hamartoma is an improper mixture of tissues within an organ, usually with an excess of one tissue type. “Strawberry birthmarks” in humans are a good example and are due to an abnormal amount of vascular tissue in the skin. Hamartomas may grossly and microscopically resemble neoplasms. 18 227.313/W Roe/General Path/2022 Topic 5: Pigments from red cell breakdown SPECIFIC LEARNING OBJECTIVES At the end of this topic you should be able to: - describe the normal process of breakdown of red blood cells, including the pigments that result from this breakdown - discuss the consequences, gross lesions and histological lesions that result from excessive red blood cell breakdown - compare and contrast intravascular and extravascular haemolysis, including identifying lesions that are specific to one or other of these processes - formulate a diagnostic plan for cases of jaundice - list the three main mechanisms of jaundice and give examples for each one - interpret basic laboratory results to determine the most likely mechanism of jaundice HAEMOGLOBIN (HB) AND HB-DERIVED PIGMENTS 1. Haemoglobin (globin) Globin chain 19 Fig 4. Structure of the haemoglobin molecule 227.313/W Roe/General Path/2022 Haemoglobin is a complex molecule made up of four subunits of the protein "globin", each of which is folded about a molecule of "heme", which is a tetrapyrollic ring with a central atom of iron. As blood passes through the spleen, old and damaged red blood cells are removed and broken down (haemolysed) by fixed macrophages. Haemoglobin is released from the lysed red blood cells, and broken down into heme, globin and iron. The heme is further processed and eventually excreted as bilirubin (see later), while the amino acids that make up globin are recycled, and the iron is incorporated into haemosiderin or ferritin. This is a normal process, and is occurring constantly at a low level as aged red blood cells are removed from the circulation. In certain haemolytic diseases, however, large numbers of red blood cells are damaged, resulting in greatly increased removal and haemolysis of erythrocytes by splenic macrophages. As a consequence there is increased formation of all the associated breakdown products. These diseases are said to cause increased extravascular haemolysis. An example is Mycoplasma haemofelis (previously known as Haemobartonella felis), a parasite of feline erythrocytes. In a second group of haemolytic diseases red blood cells rupture free in the lumen of blood vessels (intravascular haemolysis), so that haemoglobin is released directly into the plasma rather than being contained within macrophages. The presence of haemoglobin in plasma is known as haemoglobinaemia, and is recognised as a pink discolouration of plasma after blood has been centrifuged. At a certain threshold this haemoglobin may pass through the glomerular tuft and stains the urine a dark pinkish colour - this is known as haemoglobinuria. As the urinary filtrate is concentrated, some haemoglobin may be taken up by the tubular epithelial cells, where it can be seen in histological sections initially as small bright pink globules. Over time this haemoglobin is converted into orangey brown haemosiderin granules (see next section). The kidney may grossly have a red-brown discolouration. 2. Haemosiderin As described earlier, old or damaged red blood cells are taken up by fixed macrophages in the spleen, where they are broken down. Iron is released from the haemoglobin molecule and accumulates as haemosiderin, an orange-brown insoluble pigment made of iron complexed loosely with protein. Haemosiderin may later be broken down and the iron re-used. Some iron will also bind to the protein apoferritin to form a soluble storage pigment known as ferritin. A small amount of haemosiderin will always be present in the macrophages of the spleen. It can also be found, usually within macrophages, at sites of haemorrhage due to localised breakdown of red cells and haemoglobin. (Old bruises appear yellowish due to the formation of haemosiderin). Haemosiderin is also seen within macrophages of the lung (‘heart failure cells”) and/or liver in congestive heart failure, again reflecting local breakdown of red blood cells. The accumulation of large amounts of haemosiderin is known as haemosiderosis, and may be seen when there is a systemic overload of iron, e.g. in severe haemolytic disease, in diseases involving impaired iron utilisation, and following blood transfusions. In these conditions organs in which haemosiderin is deposited will appear grossly discoloured (yellow/brown). Because haemosiderin contains iron, it stains blue-black with Prussian blue-based stains (e.g. Perl’s stain). 3. Bilirubin 20 227.313/W Roe/General Path/2022 (see figure 5 below) In normal degradation of haemoglobin in the spleen, the globin molecule is split off and the heme is broken to release iron, which will form ferritin and/or haemosiderin. The remaining substance is a linear tetrapyrrolic orange pigment known as bilirubin, which is released into the blood, where it becomes loosely coupled to albumin. It is now referred to as free bilirubin and is normally found in small amounts in the bloodstream. Because of this loose protein coupling it is comparatively insoluble and is not excreted through the kidney but gets transported to the liver where it is taken up by hepatocytes. Here it is detached from albumin and forms an ester with two molecules of the sugar glucuronic acid to become a more soluble molecule known as conjugated bilirubin. Conjugated bilirubin is excreted in the bile via the bile duct to the small intestine where a further series of changes occur. In the intestine, conjugated bilirubin is changed to a group of colourless substances referred to as urobilinogens. Some urobilinogen is reabsorbed and excreted in the urine where it is oxidised, giving the yellow/brown coloration of urine. Similarly the brown coloration of faeces is due to stercobilin, also an oxidised product of the urobilinogens. Jaundice (also known as icterus) describes a state where the tissues of the body are abnormally yellow, and is due to a high concentration of bilirubin in the blood and tissues. Jaundice is most apparent in pale tissues such as fat and sclera of the eye. (a) Causes of jaundice There are three mechanistic causes of jaundice: (i) Haemolytic (pre-hepatic) jaundice This occurs when there is a greatly increased breakdown of red blood cells. Note that as described earlier, haemolysis can be intravascular or extravascular. Both can result in jaundice, but it is only with intravascular haemolysis that you will also see free haemoglobin in the bloodstream and in the urine (pink plasma/serum and urine). Examples of haemolytic jaundice: - some types of leptospirosis. Leptospirosis is caused by a group of related bacteria in the genus Leptospira. Some serotypes of this bacteria (for example Lepto pomona) produce an exotoxin that causes haemolysis. - Mycoplasma haemofelis (previously Haemobartonella felis) is an organism that parasitises red cells of cats and can cause extravascular haemolysis. - Incompatible blood transfusions occur when a patient receives blood that contains antibodies against their own red blood cells. These antibodies then bind to the patient’s red cells, causing either aggregation and removal of clumps of red cells (i.e. extravascular haemolysis) or lysis of red cells within the bloodstream (i.e. intravascular haemolysis) - Neonatal icterus is a similar condition that occurs in humans and horses when a neonate has circulating antibody in their blood stream that is directed against their own red cells. Foals ingest these antibodies when they first drink colostrum from a dam that has been sensitised against the foal’s blood group. In primates this antibody can cross the placenta, so affected human and non- human primate babies are born with anaemia and jaundice. Icterus in neonates is especially important since free bilirubin is toxic to neurons and can result in permanent loss of nerve cells. 21 227.313/W Roe/General Path/2022 (ii) Toxic (hepatic) jaundice This occurs when there is damage to the liver so that hepatocytes are unable to adequately conjugate and excrete bilirubin, resulting in a build-up of unconjugated bilirubin. The term ‘toxic’ here is a bit misleading as the hepatic damage can be due to a variety of causes other than toxins, for example: - Some serotypes of leptospirosis (e.g. L copenhageni in dogs) cause hepatocellular necrosis - Some viruses can cause hepatic jaundice via damage to hepatocytes. Examples include hepatitis A and B in humans, and infectious canine hepatitis virus in dogs - Copper poisoning. When there is excessive dietary intake of copper, much of the excess copper is stored in the liver. With chronic exposure to high dietary copper levels the amount of copper stored within the liver reaches toxic levels, and hepatocellular necrosis occurs. This results in further release of copper from dead and dying liver cells, and potentiates the liver damage, resulting in impaired conjugating ability and hence in jaundice. (Note also that this released copper spills over into the bloodstream, and once it exceeds the carrying capacity of serum proteins such as albumin and ceruloplasmin, it causes damage to red blood cell membranes which results in intravascular haemolysis; thus chronic copper poisoning causes jaundice by two mechanisms.) (iii) Obstructive (post hepatic) jaundice This occurs when there is obstruction to the excretion of conjugated bile due to blockage of the bile duct system. This obstruction, which is also known as cholestasis, can be classified as: - Extra-hepatic. This is where the obstruction is in the large bile ducts that drain from the liver. Examples include gallstones (choleliths), biliary tract tumours, bile duct fibrosis or inflammation, parasites within the bile ducts) - Intra-hepatic. This occurs when there is obstruction of the tiny bile canaliculi that travel between hepatocytes, and is usually due to hepatocellular swelling (ie it is secondary to hepatic jaundice). As a consequence of obstruction, conjugated bilirubin is not excreted via the bile duct but is released directly into the blood. If there is complete obstruction of the bile duct, urobilinogens are unable to reach the intestinal tract, resulting in pale faeces. This is very uncommon in practice, since only a tiny amount of pigment is required to impart a brown colour to faeces. 22 227.313/W Roe/General Path/2022 Fig 5. Bilirubin production from red blood cells. 23 227.313/W Roe/General Path/2022 Session 6: Tutorial (online cases) This time has been set aside as self-directed time. You don’t need to come to class for this session. Note that although there is class time set aside to do this, you can access the cases at any time you like. The learning modules for this tutorial are on Stream. Go to the General Pathology topic page and scroll down to the heading : “Online cases for General Pathology (Wendi Roe)”. By the time you get to this session you should be able to work through General Pathology Cases 1-4, but you may need to have your lecture notes nearby so that you can refer to them if you get stuck. A note about these online case study tutorials The aim of the modules is to give you a chance to apply the knowledge you gain in lectures to case scenarios, and to give you a chance to check your progress and understanding of the concepts you have learned. Each case study will generate a mark at the end – please note that this mark is not recorded, and is for your own information only. You will however receive marks for completing each module, and this will contribute to the tutorial assessment component of your final mark for this course – if you complete all the modules you will get the full mark allocation for tutorial contributions for this section of the course. Cases 1 – 4 cover material from the first three topics (Principles of General Pathology, Cell Degeneration and Necrosis) Cases 5 – 8 cover material from topics 2-4 (Cell Degeneration and Necrosis, Cellular Adaptations), along with some of the concepts covered in topics 5-10. You don’t need to wait until we’ve covered topics 5-10 to do these cases, but if you do want to do them early it will be helpful to have your lecture notes on hand. 24 227.313/W Roe/General Path/2022 Topic 6: Cellular accumulations/pigments – melanin and fat SPECIFIC LEARNING OBJECTIVES: By the end of this topic you should be able to: - give definitions for the terms written in bold - recognise lesions associated with increased and decreased melanin pigmentation - give examples of diseases and syndromes associated with abnormal melanin production - explain the pathogenesis of fatty liver and diagnose some of the causes of this condition INTRODUCTION In this group of conditions there is either an abnormal substance or large amounts of a normal substance in cells or tissues. The substance can accumulate inside cells or between them. In many intracellular infiltrations the substance is stored in lysosomes, which for one reason or another do not have the enzymes that are needed to break the material down. In other cases, the substance stored cannot be degraded. There are numerous examples of pigment accumulation in veterinary medicine, but we will focus here on melanin and pigments associated with red blood cell breakdown. MELANIN Melanin occurs naturally in the skin, hair, iris and choroid as yellow brown granules and is responsible for the brown or black pigmentation of these organs. It is formed by melanocytes, cells of neuroectodermal origin that have the ability to oxidise and polymerise tyrosine (via the copper dependent enzyme tyrosinase) which then complexes with sulphur-containing proteins to form melanin granules within the cytoplasm of the melanocytes. Variations in colour are due to variations in the effectiveness of these cells in the production of melanin rather than to relative deficiency or excess of the melanocytes themselves. Melanin can be shed from melanocytes and transferred to the epithelium of skin and hair or taken up by macrophages. Local hyperpigmentation by melanocytes occurs in freckles. Abnormal conditions of melanin pigmentation include: 1. Decreased formation of melanin: 25 227.313/W Roe/General Path/2022 (a) Albinism is a genetically induced condition where no melanin is produced by melanocytes within the whole body. It is most commonly due to an inherited deficiency of the tyrosinase. (b) Acquired hypopigmentation associated with: - hormonal imbalances - local loss of melanocytes due to physical trauma or chemical injury e.g. scars; freeze branding - copper deficiency producing generalised hypopigmentation (tyrosinase is a copper dependant enzyme) 2. Increased formation of melanin: (a) Acquired melanosis Ultraviolet light stimulates an increase in melanin production in exposed skin (seen as a suntan). (b) Congenital melanosis If melanocytes don’t disperse evenly during embryonic development, this can lead to discrete focal areas of hyperpigmentation, most often in the lung or liver. These hyperpigmented lesions have no clinical significance. (c) Moles (nevi) are dermal accumulations of melanoblasts, particularly in humans. They can transform into neoplasms (melanoma), particularly following chronic injury due to sun exposure. (d) Environmental (acquired) melanosis Some plants contain melanin precursors that can accumulate over time in various organs. These lesions are not clinically significant. FATTY CHANGE (Also known as lipidosis, steatosis, fatty degeneration, or fatty infiltration.) Fatty change is the abnormal accumulation of triglycerides in parenchymal cells. Some tissues normally contain significant amounts of fat (e.g. adipose tissue, adrenal glands, renal cortical tubular epithelium of cats, Sertoli cells of the testicle) but the accumulation of fat in cells of the liver, heart, muscle and kidney is abnormal. The liver is the most common organ affected, as it is the main organ involved in fat metabolism. Under normal conditions, lipids are transported to the liver from the diet (as chylomicrons or as free fatty acids) and from adipose tissue (as free fatty acids). They are taken up by hepatocytes and most are then stored as triglycerides (synthesised in hepatocytes by esterification of fatty acids and glycerol), while the remainder may be converted to cholesterol, incorporated into phospholipids, or oxidised within mitochondria to ketones. Triglycerides can be secreted from the liver bound to specific carrier proteins known as apoproteins. The apoprotein-triglyceride complexes are known as ‘very low density lipoproteins’. Excessive fat accumulation in the liver (fatty liver) occurs when these pathways are disrupted, either by: 26 227.313/W Roe/General Path/2022 (i) increased fat mobilisation - starvation, particularly in obese individuals - pregnancy toxaemia/ketosis in last gestation ewes carrying twins (“twin lamb disease; sleepy sickness”) - ketosis in high producing dairy cows in early lactation - diabetes mellitus(due to decreased carbohydrate metabolism associated with lack of insulin) (ii) decreased metabolism of fat within hepatocytes, e.g. due to abnormal mitochondrial function - pregnancy toxaemia of ewes - alcoholism in humans - toxic damage to hepatocytes (iii) reduced transport of fat out of hepatocytes - often due to decreased synthesis of apoprotein, e.g. in starvation or protein malnutrition Over time, fat progressively accumulates in cells. In acute (recent) fatty change there will be numerous small globules of fat around a central nucleus. In more chronic (long-standing) disease the globules coalesce into one or more larger globules, which may displace the nucleus towards the margin of the cell. When tissues are routinely processed into paraffin wax for histology, the solvents used dissolve out the fat, leaving clear spaces. To specifically stain fat, frozen sections and special stains such as Sudan III or Oil Red O can be used. Grossly, fatty livers appear pale and greasy, are crumbly (friable) and bulge out from the cut surface. In extreme cases, affected liver tissue may float in water (or formalin). 27 227.313/W Roe/General Path/2022 Topic 7: Disturbances in circulation I – Increases and decreases in blood supply SPECIFIC LEARNING OBJECTIVES: By the end of this topic you should be able to: - provide definitions for the terms written in bold - give examples of disease conditions or syndromes associated with hyperaemia, congestion, ischaemia and infarction - apply your understanding of alterations in blood supply to clinical situations in order to determine the consequences and lesions that could result from such alterations - recognise and diagnose common diseases associated with altered blood supply INCREASED BLOOD IN THE TERMINAL VASCULAR BED 1. Hyperaemia Under normal circumstances only part of a capillary bed will have blood flowing through it as a large functional reserve is maintained. If more blood is needed in an area then more arterial blood may be pumped to it and the reserve capillaries will become filled with flowing blood. The increased number of red cells in the area will give it a general redness and small arterioles and venules may stand out visually. This acute, active process is known as hyperaemia. Hyperaemia can be either physiological (associated with changed physiological need such as the hyperaemia that occurs in the stomach and intestine during digestion) or pathological, for example in association with inflammation. 2. Congestion Congestion also results in an increased amount of blood in a tissue, but in congestion this is due to obstructed flow of blood out of the tissues rather than increased inflow as in hyperaemia. Congestion may be caused by either physical obstructions within or surrounding veins, or failure of forward flow of blood because of a failing heart. The blood accumulates in dilated capillaries and venules giving the tissue a red appearance. The redness will be somewhat darker than in hyperaemia though, and will have a bluish tinge, as the haemoglobin of red cells will be less oxygenated. (a) Sub-types of congestion: 28 227.313/W Roe/General Path/2022 (i) Localised congestion is commonly seen where there is a local restriction of venous blood flow from a particular part of the body. This may be due to thrombi within veins or collapse of a vein due to external pressure e.g. tumour, tight plaster cast or bandage, tourniquet. (N.B. external pressure collapses thin walled veins much more easily than thicker walled muscular arteries). (ii) Systemic venous congestion (generalised congestion) involves a larger part of the body and is most commonly seen in heart failure. Due to a deficient forward flow of blood through the failing heart, blood dams back in the great veins affecting quite wide areas of tissue as the pressure is transmitted back through the venous system. (iii) Hypostatic congestion occurs due to the force of gravity. Gravitation of blood to dependent parts of an organ or body occurs when there is peripheral circulatory failure and the blood vessels are relaxed. It therefore occurs in weak sick animals just before death. At post mortem examination, the presence of hypostatic congestion in organs such as the lung allows us to determine the posture of the animal at death. The lung nearest to the ground will often be heavy and a very dark red due to the terminal gravitation of blood into this organ. (b) Effects of congestion If local venous congestion is gradual in onset e.g. a slow growing tumour pressing on a vein, there may be time for the development of an adequate collateral circulation through other veins and the effects will thus be minimised. When passive congestion local or systemic continues for a long time i.e. it is chronic, there are a number of serious effects: - oedema (accumulation of fluid from blood vessels in surrounding tissues) - ischaemia (lack of blood supply to affected tissues) and infarction (death of cells deprived of oxygen) - diapedesis (loss of red cells through intact capillary walls) or haemorrhage due to rupture of capillaries These last two effects are particularly seen in chronic venous congestion of the lung and liver, for example during congestive heart failure. In left sided heart failure there is an increase in pulmonary vascular pressure due to congestion of the pulmonary circulation. Capillaries in alveolar walls become distended with blood. They become more permeable and fluid (oedema) and erythrocytes escape into the alveolar spaces. Macrophages move out into the alveolar space to remove this material. They engulf red cells and the haemosiderin that results from their breakdown can be seen microscopically as brownish granules in the macrophages. These pigment-loaded macrophages are often called "heart failure cells" because of their association with pulmonary congestion resulting from heart failure. They are, however, seen in other conditions besides heart failure. In right sided heart failure there is congestion of the systemic vasculature, particularly the liver. A congested liver appears larger and darker in colour than normal. Often there is a mottled pattern on the capsular and cut surface, caused by dark red congested centrilobular parenchymal areas about the centrilobular veins contrasted with pale peripheral (portal) parts of the lobule. Such a liver may be 29 227.313/W Roe/General Path/2022 referred to as a "nutmeg" liver due to a similarity in appearance to a nutmeg. The build-up in pressure in the central veins eventually causes the adjacent liver cells to atrophy or even die. Later there may be fibrosis particularly about the central veins. This is known as cardiac cirrhosis. Haemosiderin deposits can also be seen in local macrophages due to phagocytosis of the pooled red blood cells. DECREASED BLOOD IN THE TERMINAL VASCULAR BED Ischaemia 1. Definition Ischaemia is defined as a condition of inadequate blood supply to an area of tissue relative to its needs. Literally it means "without blood". 2. Consequences of ischaemia An inadequate blood supply results in: (a) Oxygen deprivation (anoxia or hypoxia). This is the most important factor (b) Local nutrient deprivation. In practice this is probably not a limiting factor because the blood contains more glucose and amino acids than could possibly be metabolised by the oxygen present. (c) Failure to remove waste products. The accumulation of metabolites is probably the cause of pain in muscle ischaemia 3. Causes of ischaemia Ischaemia may result from a wide variety of causes as illustrated in some examples given below: (a) Cardiac arrest During cardiac arrest the blood supply to the whole body is stopped but the brain is affected first by the ischaemia because it is particularly sensitive to anoxia. If the arrest lasts more than four minutes the brain is severely damaged. (b) Arterial obstruction This may be caused by: (i) clotting of blood within the artery, i.e. thrombosis (see later) (ii) a small circulating body (an embolus) such as a clot lodging in an artery and thereby obstructing it, i.e. embolism (see later) (iii) spasm of the arterial wall as in ergot poisoning (iv) arteritis leading to thrombosis and obstruction, e.g. bovine malignant catarrhal fever or invasion of the arterial wall by parasites such as Strongylus vulgaris in the horse (v) occlusive external pressure such as might come from an adjacent space occupying lesion or from a tight bandage (vi) arterial occlusion e.g. gastric volvulus 30 227.313/W Roe/General Path/2022 (c) Venous obstruction may impede the arterial blood flow to an area sufficiently to cause significant ischaemia. (d) Capillary damage. Damaged capillaries or occlusion of capillaries may also cause ischaemia. This is the mechanism whereby pressure sores and bed sores are produced. The nursing procedures employed in the treatment of recumbent animals are largely aimed at maintaining capillary circulation to the skin and subcutaneous tissues at vulnerable points. (e) Hypovolaemia. (f) Severe vasodilation. 4. Factors modifying the effects of ischaemia: The effects of ischaemia are predominantly those mediated by tissue anoxia and hypoxia. The effects depend on a number of modifying factors: - some tissues (e.g. the brain) are more sensitive to hypoxia than others (e.g connective tissue) Sensitivity of the tissue to hypoxia. - if ischaemia develops quickly, there is not time for the body to adapt. Conversely, if it develops slowly then factors such as developing collateral circulation can decrease the impact of ischaemia. - prolonged ischaemia is more likely to produce tissue damage than ischaemia of short duration. - an animal with well oxygenated blood will have fewer effects of ischaemia compared with an anaemic animal. - lowering the temperature of tissue lowers the metabolic rate and therefore the oxygen demand. This principle is applied in hypothermic surgery as a means of avoiding tissue damage resulting from ischaemia. INFARCTION Definition An infarct is a localised area of necrotic tissue that occurs as a result of ischaemia. The process by which an infarct develops is known as infarction. General principles Most infarcts are caused by obstruction of arterial supply, although occasionally venous infarcts can occur. The organs most commonly infarcted are those with a terminal vascular bed type arterial supply, such as the kidney, spleen, limb extremities, brain and intestines. In contrast, organs with a dual blood supply (e.g. lungs (pulmonary and bronchial vessels) and liver (hepatic artery and portal vein)) seldom develop infarcts. Development of an infarct Following obstruction to an end artery the hydrostatic pressure within the capillary bed will decrease, and venous blood will tend to flow back into the tissue. This means that acute infarct tend to be red. Hypoxia of the venous and capillary walls will result in loss of fluid and sometimes frank haemorrhage 31 227.313/W Roe/General Path/2022 into surrounding tissues. The increased amount of fluid in early infarcts causes them to appear swollen. Continuing hypoxia results in the affected tissue become degenerate and eventually necrotic. As degenerate cells swell, some tissue fluid is forced out of the infarcted area, This factor, in combination with lysis of leaked red cells, means that the infarct tends to become paler with time. The degree of swelling will also decrease. At the edges of the infarct the necrotic tissue will induce inflammation (a rim of hyperaemia or line of demarcation). Presuming the individual lives, macrophages and neutrophils will invade the infarct to remove necrotic tissue. Eventually healing is by fibrosis leaving a depressed scar on the surface of an organ such as the kidney. In human medicine, cardiac infarction due to thrombus formation in the coronary arteries is particularly common. With severe myocardial infarction and peracute death the changes descried above do not have time to develop, and the heart may appear completely normal at post mortem. Microscopic examination may reveal subtle or absent signs of tissue death and diagnosis may depend on demonstrating a blocked coronary artery. Tutorial 2 – Case studies In this section of class we will use some conditions that will be familiar to you in order to start thinking in a diagnostic way – integrating the new information presented in class with your existing knowledge (physiology, anatomy, general medical knowledge) in order to understand disease processes and outcomes. 32 227.313/W Roe/General Path/2022 Topic 8: Disturbances of circulation II – haemorrhage and shock SPECIFIC LEARNING OBJECTIVES: By the end of this topic you should be able to: - give definitions for the terms written in bold - describe the local and systemic effects of haemorrhage - describe the mechanisms that compensate for blood loss and their implications for diagnosis - give examples of disease syndromes that are commonly associated with blood loss - describe the different types of shock and give examples of each - demonstrate your understanding of the pathogenesis of shock HAEMORRHAGE Definitions The term haemorrhage is used to describe bleeding from a transected or ruptured blood vessel or from the heart. Haemorrhage may be caused by: trauma (the most common cause) vascular disease e.g. vasculitis abnormally weak blood vessels e.g. collagen defects local high blood pressure (e.g. severe exertion) abnormal clotting Haemorrhage can be focal (e.g. localised trauma) or diffuse (e.g. from mucosal surfaces in some clotting defects). Diapedesis is a small momentary spurting loss of blood that can occur between endothelial cells of otherwise normal capillaries. A haematoma is a localised collection of blood, usually clotted, that forms a tumour-like swelling in tissues. Very small haemorrhagic spots up to 1-2mm in diameter are referred to as petechiae (petechial haemorrhage). Slightly larger foci of haemorrhage are referred to as ecchymoses (ecchymotic haemorrhage). 33 227.313/W Roe/General Path/2022 Purpura is a clinical term meaning a disease characterised by haemorrhages in the skin, mucous membranes and viscera. Effects of haemorrhage (a) Local effects. Small haemorrhages as a rule have very little effect per se unless they are in some vital area such as the brain stem. Larger haemorrhages may cause significant "space occupying lesions" and cause severe mechanical effects (e.g. haemopericardium which will interfere with ventricular filling of the heart in diastole). Haemorrhages into lung airways may cause asphyxia while those into joints cause pain. Small amounts of extravasated blood may be absorbed leaving little in the way of tissue stigmata. Larger haematomas are often replaced by fibrous tissue and there is persistence of degraded blood pigment, e.g. haemosiderin, within macrophages at the site of the haemorrhage for some considerable time. (b) Systemic effects of haemorrhage. This depends on the total amount of blood lost, and the speed at which it is lost. If blood loss is chronic, with small amounts of blood lost at any one time, an iron deficiency anaemia may develop. Bleeding gastrointestinal ulcers are a good example of this. Acute (sudden) loss of 1/3 or more of an individual’s total blood volume can cause shock and death. If the same volume of blood is lost over a period of 24 hours or more then compensatory mechanisms (see next section) have time to kick in and death may not occur. If blood loss is acute, but less than 20% of total blood volume is lost then the systemic effect will be slight, as the body can compensate fairly quickly. (There are of course individual variations in susceptibility to blood loss). 3. Compensatory mechanisms (a) Redistribution of blood to vital centres. This occurs by a combination of splenic contraction and selective arteriolar constriction. The spleen contains a large pool of red cells, and contraction of the spleen is a very early response to severe haemorrhage, helping to maintain blood pressure. Simultaneously, stimulation of adrenergic nerves results in constriction of arterioles that supply less essential organ systems such as the skin and intestine. This results in redistribution of blood to the vital centres (brain, heart, diaphragm). (b) Restoration of plasma volume This is the second phase of compensation, and occurs because the arteriolar constriction described above results in decreased intravascular pressure in capillary beds, and consequently there is a tendency for extravascular fluid to move into the blood vessels. This is also known as haemodilution. Haemodilution is complete about 2 days after the original haemorrhage. At this time the packed cell volume (PCV) will give a good indication of the size of the original blood loss. (Note that immediately 34 227.313/W Roe/General Path/2022 after a haemorrhage, the PCV will be normal and can’t be used as an indication of the total amount of blood lost. It can, however, serve as a base line with which to compare later blood samples.) (c) Replacement of lost erythrocytes. Loss of red cells stimulates the bone marrow to start producing and releasing more red cells (erythropoiesis). Within about 5 to 6 days the reticulocyte (immature red blood cell) count may reach 10% indicating active red cell formation in bone marrow. Normally, reticulocytosis abates by about 12 days. Failure to do so may indicate recurrent haemorrhage. If these compensatory mechanisms fail to overcome acute blood loss then shock occurs (see next section). SHOCK Definition. Shock is peripheral circulatory failure resulting from a disparity between the amount of blood present and the volume or capacity of the vascular system. Cause Shock is caused by: (a) a sudden decrease in circulating blood volume (hypovolaemia e.g. due to haemorrhage or severe dehydration). (b) an increase in the vascular space, caused by systemic vasodilation (widespread dilation of blood with pooling of blood in tissues) (c) failure to effectively circulate blood throughout the vascular system Classification of shock (a) Hypovolaemic shock This occurs when there is a dramatic decrease in the volume of blood present and can be due to loss of fluid only (e.g. severe dehydration) or loss of whole blood (haemorrhage). Examples include: - severe acute haemorrhage (> 20 ml blood/kg body weight) - prolonged diuresis (i.e. loss of dilute fluid through the urine) - persistent emesis (vomiting) or diarrhoea - torsion (twisting) or gastrointestinal blockage with leakage of plasma - extensive burns (b) Vasculogenic shock Vasculogenic shock occurs when there is either widespread vasodilation or widespread damage to a large number of capillary beds. Examples include: - vasodilation due to endotoxin produced by gram negative bacteria (septic shock) 35 227.313/W Roe/General Path/2022 - widespread endothelial damage due to hypoxia (c) Cardiogenic shock This is a result of decreased cardiac output, and can be caused by: - acute changes in myocardial function e.g. large infarcts, dysrhythmias, decreased contractility - decreased filling with blood (diastolic dysfunction) e.g. cardiac tamponade - the heart failing to empty adequately (systolic dysfunction) e.g. increased vascular resistance, ruptured chordae tendinae 4. Consequences of shock. Shock may be reversible or irreversible. If an animal loses enough blood to result in a marked drop in blood pressure but the blood is immediately replaced, the blood pressure will return to normal. This is known as reversible shock. If there is a longer period between the drop in blood pressure and replacement of the blood volume, then eventually there will be a ‘tipping point’ where transfusion can no longer reverse the shock, hypotension will worsen and the animal will die. The mechanism behind irreversible shock is systemic hypoxia - inadequate oxygen supply to cellular systems. Hypoxia results in widespread damage to endothelial cells with leakage of plasma from vessels, causing sludging and stasis of blood-flow. Organs switch to anaerobic metabolism in the absence of oxygen, causing a build up of acid metabolites in the circulation, which in turn result in loss of vascular tone. Hypoxia also has damaging effects on specific organs which contribute to the spiralling effects, including hepatic necrosis, renal failure, myocardial failure and severe depression of the central nervous system. One of the central concepts of treatment of shock is rapid recognition and intervention to maintain circulation, oxygenation and tissue function before irreversible damage occurs. 5. The effect of temperature on shock. In the shocked patient, the skin is cool because of the selective redistribution of blood away from this tissue. There is also a reduction in body heat output as a result of the interference with carbohydrate metabolism. Over warming a patient may merely serve to dilate skin vessels and further reduce the effective blood volume for more vital organs. Alcohol has the same effect and is contra-indicated. 36 227.313/W Roe/General Path/2022 Fig 7. Pathogenesis of shock. 37 227.313/W Roe/General Path/2022 Topic 9: Disturbances of circulation III – Thrombosis and embolism SPECIFIC LEARNING OBJECTIVES By the end of this topic you should be able to: - provide definitions for the terms written in bold - distinguish between ante-mortem and post-mortem blood clots - describe the formation of thrombi and the possible sequelae - demonstrate a detailed understanding of the importance, consequences and pathogenesis of thrombosis and embolism in common disease syndromes THROMBOSIS Definitions Coagulation is the process of blood clotting. This clotting can be ante-mortem, in which case it is known as thrombosis, or post-mortem. A clot that forms during life is known as a thrombus, while those that form after death are known simply as post mortem clots. The process by which a thrombus forms is known as thrombosis. While thrombosis is a relatively common event and can have serious clinical implications it is important to note that the vast majority of thrombi resolve without significant incident. Thrombosis can be divided into large vessel thrombosis and microvascular thrombosis (which is also called disseminated intravascular coagulation (DIC)). Pathogenesis Thrombi can form due to: (a) Damage to the blood vessel wall Damage due to direct trauma, inflammation, temperature extremes, infectious agents or chemical irritants results in activation of tissue factor, which then triggers a series of events that lead to clotting (see Haemostasis later). The blood clot that forms will usually be attached to the vessel wall at the site of damage. (b) Abnormal flow of blood. Factors such as blood stasis (e.g. dilated or varicose veins, aneurysms, poor peripheral circulation) or disturbances of laminar flow (e.g. at vessel branches or in areas where there are irregularities of the vessel wall) will promote clotting. 38 227.313/W Roe/General Path/2022 (c) Changes in blood composition Such changes may include haemoconcentration (e.g. severe dehydration) or increased concentrations of procoagulant substances. These categories constitute Virchow’s triad of thrombosis. Damage to vessel walls is the single most important cause, and is the most likely initiator of thrombosis. The other two categories are important because they influence the progression of thrombus growth and its severity. Progression of thrombosis The first phase of thrombus formation involves the deposition of platelets on damaged endothelium. In the second phase activation of the intrinsic clotting mechanism results in the formation of strands of fibrin which are layered on the platelet plug. A second layer of platelets may be deposited followed by further deposition of fibrin. The thrombus may thus have a layered or onion skin appearance due to sequential deposition of fibrin and platelet layers. The precise pattern of alternating bands may be influenced by flow patterns and eddy currents and may be quite complex. Large vessel thrombosis is particularly common in humans, for example in coronary thrombosis due to atherosclerosis, deep vein thrombosis, and varicose veins. There are also some conditions in domestic animals also where thrombosis of large vessels is a feature, for example in cats with dilated left atria, and cattle with thrombosis of the vena cava following hepatic abscessation (see liver notes). 39 227.313/W Roe/General Path/2022 Microvascular thrombosis is widespread clotting within multiple small vessels, particularly capillaries. It can be triggered by anything that damages a large number of capillary beds, e.g. sepsis, hyperthermia, severe hypotension. It is also known as disseminated intravascular coagulation (DIC). Consequences of thrombosis. There are several things that can happen to a thrombus. Thrombi that fill an entire vessel and block blood flow are known as occluding thrombi. If the thrombus is in a small vessel, it may undergo lysis by the plasminogen-plasmin system and disappear, with little or no damage to the surrounding tissue. Occluding thrombi in slightly larger vessels can shrink and be organised over time, allowing blood to pass by to supply distal tissues. The new channel that results becomes lined with endothelial cells: this process is called recanalization. If neither of these two process occur (lysis or recanalization), then ischaemia and infarction will result. The severity of the consequences will depend on the tissue involved, the size of the affected vessel and the presence or absence of a collateral blood supply. When a thrombus only partially fills a vessel (i.e. it is not occlusive), then blood will constantly flow over the surface of the thrombus, and can dislodge part of the clot which will then become an embolus (thromboembolism). Fig 15. Possible sequelae of non-fatal thrombi 40 227.313/W Roe/General Path/2022 Ante and post mortem clots In many (but not all) cases gross examination of a blood clot can determine whether it formed before or after death: (a) Ante mortem thrombi - produced in flowing blood - attached to wall of vessel at least at site of initial injury - usually not adapted fully to shape of the vessel - gross appearance irregular and rough with a stringy texture - colour depends on proportion of red cells in it, usually a dirty red-grey colour - fibrin, cells and platelets appear to be laid down in layers (b) Post mortem clots - produced after death in static blood - not attached to vessel wall though may intertwine with chordae tendinae of the heart valves - moulded exactly to shape of vessel - smooth and glistening - texture rubbery - either dark red (currant jelly) or in larger vessels separated into a ventral red clot below a yellow "chicken fat” clot - no layering EMBOLI AND EMBOLISM Definition An abnormal mass circulating in the blood stream is an embolus. Should an embolus become arrested in a vessel and occlude it, then the process is known as embolism. Types of emboli (a) Thromboemboli - formed from thrombi, and can be either bland (sterile) or septic. (b) Gas - e.g. air or nitrogen. Injection of air accidentally or on purpose may cause a fatal brain embolism. Injection of air may be used as a method of euthanasia particularly in poultry. In decompression sickness (bends), bubbles of nitrogen come out of solution as pressure reduces. (c) Fat - commonly follows orthopaedic injuries of surgery (d) Neoplasms (cells from cancerous growths). 41 227.313/W Roe/General Path/2022 Neoplastic emboli may initiate secondary cancers elsewhere in the body. The process is known as metastasis. (e) Foreign bodies, parasites, clumps of bacteria, pus, injected material. (d) Cartilage derived from intervertebral discs (lodge in vessels in the spinal cord) Causes of embolism One of the most important causes of embolism is when fragments of a thrombus detach and begin to circulate freely within the bloodstream – this is known as thromboembolism. Arterial thromboemboli usually arise from the left side of the heart where the initial thrombus has developed on the heart valves as part of an endocarditis. These thrombi are usually septic. Venous thromboemboli can also occur – this is more common in humans following the development of deep vein thrombosis. Sequelae of embolism - spread of infection for septic emboli - infarction of a tissue if the embolus fully occludes a vessel - nothing: if emboli are small and sterile or if the organ has a good collateral circulation then there may no observable effects Tutorial 3 – Case studies Details will be provided in class 42 227.313/W Roe/General Path/2022 Session 9: Tutorial (self-directed) This time has been set aside as self-directed time. You don’t need to come to class for this session. You can use this time to work on the online case module tutorials, which you will find on Stream under “Online cases for General Pathology (Wendi Roe)”. At this point you should be able to work through Cases 1-8, but may need to have your lecture notes nearby to refer to. Note that although there is class time set aside to do this, you can access the cases at any time you like. 43 227.313/W Roe/General Path/2022 Topic 10: Oedema SPECIFIC LEARNING OBJECTIVES: By the end of this topic you should be able to: - describe in detail the four mechanisms of oedema development and give examples of each - be able to interpret basic laboratory data to diagnose the likely mechanism of oedema - demonstrate your understanding of the different possible causes of local vs. generalised oedema Definition The term oedema refers to an excessive accumulation of extracellular fluid. When oedema fluid accumulates in serous cavities it has a variety of names. In the peritoneal cavity - ascites In pleural cavity - hydrothorax In pericardial sac - hydropericardium When oedema is generalised it is known as anasarca. Formation and drainage of tissue fluid. The development of oedema is related to mechanisms that control normal fluid balances between blood and tissue i.e. variations in hydrostatic and osmotic pressure relationships between the vascular system and tissue spaces as explained by the Starling equilibrium. At the arterial end of the terminal vascular bed the combined differences in hydrostatic and osmotic pressure cause fluid and low molecular weight solutes (not protein) to flow into the tissue spaces. These bring oxygen, nutrients and minerals to tissues and help to maintain electrolyte and water balance. Much of this tissue fluid is drawn off by lymphatics, which eventually return it to the blood stream. There is some reabsorption of fluid at the venous end of the terminal vascular bed where the combined pressure differences favour such reabsorption. Oedema occurs when there is unbalanced and excessive out flow of fluid, which accumulates in and distends tissue spaces. It is important to note that lymphatics are not static components of the equation and in situations of increased extravascular fluid accumulation, lymphatic drainage will increase several-fold (if they are not blocked of course). Oedema appears when the enhanced lymphatic drainage is exceeded. The following diagram depicts these processes. 44 227.313/W Roe/General Path/2022 Mechanisms of oedema development. There are four mechanistic causes of oedema: (a) Increased hydrostatic pressure Increased hydrostatic pressure results from increased fluid within vessels, which overcomes the attractive osmotic force