pH Measurement Unit 4 PDF
Document Details
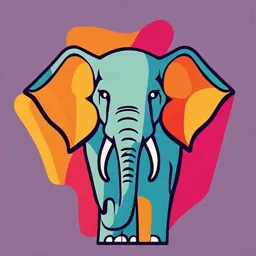
Uploaded by AdoredSwaneeWhistle
Tags
Summary
This document provides an overview of pH measurement, including the theory behind ion selective electrodes (ISEs), different electrode types, and the Nernst equation. It's suitable for chemistry or science students at secondary school or undergraduate level to understand the basic principles of pH measurement.
Full Transcript
pH Measurement Need of pH measurement To produce products with defined propertie For research and development Introductio n Ion selective electrode (ISE) is an analytical technique used to determine the activity of ions in aqueous solution by meas...
pH Measurement Need of pH measurement To produce products with defined propertie For research and development Introductio n Ion selective electrode (ISE) is an analytical technique used to determine the activity of ions in aqueous solution by measuring the electrical potential. Specific ion dissolved in a solution create an electrical potential, which can be measured by a voltmeter or pH meter. The strength of this charge is directly proportional to the concentration of the selected ion. Ion Selective Electrode Electrode Body Ion Electrical Sensitive Connection Area Electrochemical Measuring System Meter Reference Sensing Electrode Electrode Current Flow Principle consists of a thin membrane Only specific ion can be diffuse. by measuring the electric potential generated across a membrane by “selected” ions, and comparing it with reference electrode. net charge is determined. Terms used Potentiometry Use of Electrodes to Voltages that Measure Provide Chemical concentration Indicator Electrode: Electrode that responds to analyte Reference Electrode: Second ½ cell at a constant potential Cell voltage is difference between the indicator and reference electrode Combination Probe Reference Sensing Element Element Glass Membrane Electrode This method uses the electrical potential of pH-sensitive electrodes as a measurement signal. The glass electrode is the most commonly used sensor. Not having the disadvantages of the optical methods, it can be used almost universally. Solid State Electrode Electrode body of Inorganic crystalline polymer. E.g. Special Epoxide Resin with excellent mechanical properties. High temperature stability. Electrochemical Measuring System Meter Reference Sensing Electrode Electrode Current Flow NERNST EQUATION T Log C E = E + 2.3 n o E = Measured Voltage Eo = Reference Constant T = Temperature n = Charge on Ion = Ionic Strength C = Concentration NERNST EQUATION T Log C E = E + 2.3 n o E = Measured Voltage Eo = Reference Constant T = Temperature n = Charge on Ion = Ionic Strength C = Concentration NERNST EQUATION T Log C E = E + 2.3 n o } E = Measured Voltage What the Meter Tells Us Eo = Reference Constant Meter Reading Also T = Temperature Affected By All This n = Charge on Ion = Ionic Strength C = Concentration What We Want To Know NERNST EQUATION T Log C E = E + 2.3 n o } E = Measured Voltage What the Meter Tells Us Eo = Reference Constant Must Be Accounted T = Temperature For To Get True Concentration n = Charge on Ion = Ionic Strength C = Concentration What We Want To Know NERNST EQUATION T Log C E = E + 2.3 n o E = Measured Voltage What the Meter Tells Us Eo = Reference Constant T = Temperature Must Maintain Reference Electrode n = Charge on Ion = Ionic Strength C = Concentration What We Want To Know NERNST EQUATION T Log C E = E + 2.3 n o E = Measured Voltage What the Meter Tells Us Eo = Reference Constant Will Be Constant for Specific Ion, T = Temperature Whole Number, 1, 2, 3, etc. n = Charge on Ion + or - = Ionic Strength C = Concentration What We Want To Know NERNST EQUATION T Log C E = E + 2.3 n o E = Measured Voltage What the Meter Tells Us Eo = Reference Constant Must Be Controlled By T = Temperature Making It A Very High Value n = Charge on Ion = Ionic Strength C = Concentration What We Want To Know NERNST EQUATION T Log C E = E + 2.3 n o E = Measured Voltage What the Meter Tells Us Eo = Reference Constant T = Temperature Add Ions n = Charge on Ion ISAB OH - Na + = Ionic Strength C = Concentration What We Want To Know NERNST EQUATION T Log C E = E + 2.3 n o E = Measured Voltage What the Meter Tells Us Eo = Reference Constant Follow T = Temperature The Directions! n = Charge on Ion = Ionic Strength C = Concentration What We Want To Know NERNST EQUATION T Log C E = E + 2.3 n o E = Measured Voltage What the Meter Tells Us Eo = Reference Constant T = Temperature Must Be n = Charge on Ion Controlled = Ionic Strength C = Concentration What We Want To Know NERNST EQUATION T Log C E = E + 2.3 n o E = Measured Voltage Eo = Reference Constant T = Temperature n = Charge on Ion = Ionic Strength C = Concentration NERNST EQUATION T Log C E = E + 2.3 n o y = mx + b T 2.3 n = s = slope Slope is Direction of “Curve” When Plotting E vs. C Effect of Temperature s is the Direction (Angle) of the Line Electrode Potential (mv) 100o C 50o C 0o C Concentration (mg/L) Temperature Affects the Slope Effect of Temperature Electrode Potential (mv) O 100o C 50o C O O 0o C Concentration (mg/L) One Concentration will Result in Different Readings Dependent on Temperature Effect of Temperature Sample Temperature Electrode Potential (mv) X Calibration Temperature O O Concentration (mg/L) When the Sample Temperature is Different from the Calibration Temperature, an Incorrect Sample Concentration will be Obtained Effect of Temperature Electrode Potential (mv) 100o C 50o C 0o C Isopotential Point Concentration (mg/L) Effect of Temperature Electrode Potential (mv) 100o C 50o C 0o C Isopotential Point Concentration (mg/L) At the Isopotential Point, One Concentration will Result in the Same Reading Independent of Temperature Effect of Temperature Electrode Potential (mv) 100o C 50o C 0o C Normal Range of Analysis } Concentration (mg/L) The Range of Analysis is Far From the Isopotential Point for Most Samples Effect of Temperature Electrode Potential (mv) 100o C 50o C 0o C Normal Range of Analysis } Concentration (mg/L) Temperature Must be Accounted For in All Analyses Automatic Temperature Compensation ATC Adjusts Slope in Relation to Temperature Sounds Good! Does Not Work! Automatic Temperature Compensation ATC Adjusts Slope in Relation to Temperature Is Accurate Only When Analysis is Close to Isopotential Point Only For pH Automatic Temperature Compensation ATC (Except For pH) Temperature compensators correct for the change in millivolts per pH unit per the Nernst equation but do not correct for this change in the actual pH of the solution with temperature. The change in pH readings with temperature of solution pH changes is often larger than that per the Nernst equation, microprocessor- based pH transmitters have added algorithms for solution pH correction The input to the pH measurement circuit is a potential difference between the external glass surface that is exposed to the process (E1) and the internal glass surface that is wetted by a 7 pH solution (E2). If the external glass surface is in exactly the same condition as the internal glass surface, the Nernst equations simplify to the following equation, where the potential difference in millivolts is proportional to the deviation of the process pH from 7 pH at 25°C Effect of Temperature Calibrate With Standards and Electrode Potential (mv) Samples At The SAME Temperature (Usually Room Temperature) Concentration (mg/L) Calibration Control Electrode Potential (mv). Standard Solution Concentration (mg/L) The Calibration Control Adjusts the “Curve” to the Point of Standard Used Slope Control Electrode Potential (mv). Standard Concentration (mg/L) The Slope Control Rotates the “Curve” Around the Point Of the First Standard Slope Control Second. Standard Electrode Potential (mv). Standard Concentration (mg/L) The Slope Control Adjust the “Curve” through the Point of the Second Standard Used NERNST EQUATION E1 = Eo + s Log C1 E2 = E + s Log C2 o If C2 = 10 x C1 Then: E2 = E + s Log 10C1 o NERNST EQUATION E1 = Eo + s Log C1 E2 = E + s Log C2 o If C2 = 10 x C1 E2 - E 1 = s The Slope of an Electrode is the millivolt Change that is seen for a Ten Times Change in Concentration. ISE Checking Electrode Slope Carefully Prepare Two Standards 10 X Concentration Difference Set Meter to Read in millivolts (or Relative mv) Record mv Reading for Each Standard Determine Difference = Slope ISE Checking Electrode Slope T Log C E = E + 2.3 n o Electrode Potential (mv) Concentration (mg/L) ISE Checking Electrode Slope T Log C E = E + 2.3 n o The Slope Value Determined is Affected By: Temperature (T) Charge on the Ion of Interest (n) Positive or Negative 1, 2, etc. ISE Checking Electrode Slope T Log C E = E + 2.3 n o The Slope Value Determined is Affected By: Temperature (T) Charge on the Ion of Interest (n) Quality of the Standards Efficiency of the Electrode ISE Checking Electrode Slope The Slope of the Electrode Will Change {Loss of Efficiency} The Slope Should be Checked Regularly to Assure Reliable Results Most Meters Give Slope When Calibrating ( In mv or %) Record Slope for QA/QC Daily (or at least every two weeks) ISE Checking Electrode Slope Ideal Slope Depends on Ion of interest (n) Charge on the Ion of Interest (+ or -) Approximately 59 mv for n=1 or 29 mv for n=2 Check Manufacturer for Acceptable Slope Range Usually ± 10 % of Ideal ISE Checking Electrode Slope If Determined Slope is Outside of Acceptable Range May Be Due To: Poor Quality of Standards Improper Probe Maintenance Faulty Electrode The Slope Should be Checked Regularly to Assure Reliable Results Basics about pH pH is the abbreviation of pondus hydrogenii pH = -log [H+] [H+] is in molar concentration Basics about pH Basics about pH In any aqueous solution Kw = [H+] [OH-] = Dissociation constant It depends on temperature Acids [H+] increases and [OH-] decreases [OH-] increases and [H+] decreases Bases Nernst Equation E=E0 + 2.3RT*log aH+ Comparing with F standard formula for E0 = constant straight line R = the gas constant Y= a+bx a= offset T = temperature(k) b= slope of the line t 25˚C,slope=59.18mV/pH Glass electrode Dependent on hydrogen activity Glass membrane sensitive to H+ ions Glass membrane Reference electrode insensitive to the H+ ions in the solution most widely used is the silver/silver chloride electrode another commonly used reference electrode is the calomel Combined electrode Calibration 1 Calibration Offset adjustment Slope adjustment Two point calibration Temperature effect Temp Slope mV/pH 15 -57.2 20 -58.2 25 -59.2 30 -60.1 35 -61.1 Offset adjustment Sodium Error Occurs at high pH [H+] less than [Na+] Electrode respond to [Na+] Measured pH is lower than the actual pH Liquid Junction Potential Equitransferent KCL is used as fill solution Different mobility of ions result in liquid junction potential Diagnostics Glass pH Electrode Impedance - normally in the range 0f 10-100 MΩ - crack in glass electrode will result in sho Reference Electrode Impedance - Coating or blockage of the liquid junctio will increase the result Other methods Optical Methods -Indicator papers ISFET electrode -CMOS based electrode ISFET reference electrode in the solution replaces the metal gate silicon nitride, Si3N4, overlying the SiO2 is used to improve pH response Turbidity meter Turbidity measurement Turbidity measurement Turbidity measurement Single beam Turbidity meter Double beam Turbidity meter Double beam Turbidity meter Double beam Turbidity meter Double beam Turbidity meter In line Turbidity Meter In line Turbidity Meter Light scattering Measurement Light scattering Measurement Turbidity transmitter Turbidity Turbidity measurement The turbidity (T) and Total suspended solids (TSS) concentration is related as K: Turbidity factor For type of soil (i) and particle class (j) such as clay, silt and sand in each soil, the turbidity is given as The turbidity for combination of soils is given as Simulink model for turbidity measurement Simulation Result Conductivity measurement measure ionic concentration of electrolyte samples. Cells and instrumentation are designed to measure the electrical resistance (or its reciprocal) The electric field applied to the cell is E/d. The current density i/A is the sum of the individual charge carriers in the field, and therefore the conductivity L (which from Ohm’s law is the current divided by the voltage) Cell Constant Cell Constant Design Measurement of Salt, acid , alkalis in mining, paper and pulp industries, mining , aluminum industries where sample contains solids Oxygen Demand Objective – To know the different expressions of Oxygen Demand and their chemical basis, – their use in Environmental Engineering, – and the methods of laboratory determination. Oxygen Demand is a measure of organic carbon Organic pollutants complex carbon molecule Enormous range of organics Impossible to identify and quantify organics Oxygen consumed as bacteria consume organics Eg Aerobic Metabolism Quantity of oxygen consumed is a measure of the concentration of organics in water Options for measuring OD Biochemical Oxygen Demand BOD Chemical Oxygen Demand COD Total Organic Carbon TOC Biochemical Oxygen Demand "Amount of oxygen required by bacteria to break down decomposable organic matter under aerobic conditions" 45 Ultimate oxygen demand 40 5 Day oxygen demand BODu = L 35 BOD5 30 Oxygen 25 Demand 20 (mg/l) 15 10 5 Carbonaceous oxygen demand 0 0 5 10 15 20 25 Time (days) BOD Bioassay Amount of oxygen used up under defined conditions Temp 20 C Virtually all degradable matter oxidised in 20 days - BODu or ultimate BOD 5 day BOD used –BOD5 is 70-80% of the BODu BOD wastewater (mg/l) –