Stoelting Chapter 4 Notes PDF
Document Details
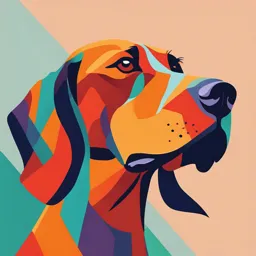
Uploaded by PreciousResilience
University of Puerto Rico, Medical Sciences Campus
Tags
Summary
Stoelting Chapter 4 provides an overview of inhaled anesthetics, including their history, development, and specific examples like halothane, methoxyflurane, enflurane, and isoflurane. It discusses the quest for ideal inhaled anesthetics and the challenges anesthesiologists face while managing their complexities.
Full Transcript
Stoel&ng Chapter 4: Inhaled Anesthe&cs History and Development of Inhaled Anesthe&cs: - Discovery of anesthe&c proper&es of nitrous oxide, diethyl ether, and chloroform in the 1840s. About 80-year hiatus before the introduc&on of other inhaled anesthe&cs. By 1950, all inhaled anesthe&cs, except ni...
Stoel&ng Chapter 4: Inhaled Anesthe&cs History and Development of Inhaled Anesthe&cs: - Discovery of anesthe&c proper&es of nitrous oxide, diethyl ether, and chloroform in the 1840s. About 80-year hiatus before the introduc&on of other inhaled anesthe&cs. By 1950, all inhaled anesthe&cs, except nitrous oxide, were flammable or liver-toxic. Introduc&on of the first halogenated hydrocarbon anesthe&c, fluroxene, in 1951 due to decreased flammability by replacing a hydrogen atom with a fluorine atom. Fluroxene was withdrawn from the market due to poten&al flammability and organ toxicity. Development and Issues with Specific Anesthe&cs: - Halothane, synthesized in 1951 and introduced in 1956, had issues with enhancing arrhythmogenic effects of epinephrine. Methoxyflurane, introduced in 1960, did not enhance epinephrine's arrhythmogenic effects but had high solubility leading to slow induc&on/recovery and caused hepa&c toxicity and nephrotoxicity. Methoxyflurane's analgesic proper&es at low concentra&ons, discon&nued in the US and Canada in the 1970s, s&ll used in Australia for brief procedures. Enflurane, introduced in 1973, did not enhance arrhythmogenic effects or cause hepatotoxicity but had side effects like metabolism to inorganic fluoride and CNS s&mula&on. Isoflurane, a structural isomer of enflurane introduced in 1981, was resistant to metabolism, reducing organ toxicity risk. Inhaled Anesthe&cs: Developments and Future Prospects: - - - The quest for ideal inhaled anesthe&cs con&nued a\er isoflurane, focusing on compounds with no halogens except fluorine, resul&ng in nonflammable, poorly lipid-soluble, and highly metabolism-resistant substances. Desflurane (introduced in 1992) and sevoflurane (introduced in 1994) are both totally fluorinated ethers (methyl ethyl for desflurane and methyl isopropyl for sevoflurane). These anesthe&cs' low solubility in blood allows for rapid induc&on, precise control during maintenance, and quick recovery from anesthesia, regardless of administra&on dura&on. The popularity of desflurane and sevoflurane is driven by market demands, such as ambulatory surgery and rapid awakening needs, rather than significant pharmacological improvements over isoflurane. Challenges for anesthesiologists: leveraging pharmacokine&c benefits while managing risks like airway irrita&on, sympathe&c s&mula&on, carbon monoxide produc&on (desflurane), compound A produc&on (sevoflurane), and the higher costs of these anesthe&cs. Cost Considera&ons in Inhaled Anesthe&cs: - The cost is a cri&cal factor in the adop&on of new inhaled anesthe&cs. Influen&al cost factors include: 1. Price per milliliter of liquid. 2. Anesthe&c characteris&cs: § vapor pressure (milliliter of vapor per milliliter of liquid) 1 - - § potency § solubility 3. Selected fresh gas flow rate for anesthe&c delivery. Using low fresh gas flow rates can reduce the costs of new inhaled anesthe&cs. Less soluble anesthe&cs, like desflurane, are ideal for low gas flow rates due to beaer control over delivered concentra&on and less deple&on from inspired gases. This conserva&on balances out lower potency drugs compared to more potent ones like isoflurane. Example: Desflurane's potency is one-fi\h that of isoflurane, but the required amount to maintain minimal alveolar concentra&on (MAC) is only slightly more than three &mes that of isoflurane. Similarly, while sevoflurane's MAC is 74% greater than isoflurane, the amount needed to sustain MAC is only 30% more. Clinically Useful Inhaled Anesthe&cs: Commonly used inhaled anesthe&cs include: - Inorganic gas: Nitrous oxide. - Vola&le liquids: Isoflurane, desflurane, and sevoflurane. - Halothane and enflurane are less frequently used but important for compara&ve pharmacology studies, especially halothane. - Vola&le liquids are administered as vapors, vaporized in devices called vaporizers. - Diethyl ether and chloroform, now mostly used in veterinary medicine, are s&ll available. - Xenon, an inert gas with anesthe&c proper&es, is clinically limited due to its high cost. Nitrous Oxide in Anesthesia: Characteris&cs and Use: - Low-molecular-weight, odorless to sweet-smelling nonflammable gas. - Low potency and poor blood solubility (blood:gas par&&on coefficient 0.46). - Commonly administered with opioids or vola&le anesthe&cs for general anesthesia. - Supports combus&on despite being nonflammable. - Rapid achievement of alveolar and brain par&al pressure due to poor blood solubility. Effects and Muscle Relaxa&on: - Prominent but short-lived analgesic effects, dissipa&ng a\er about 20 minutes. - Seda&ve effects persist longer than analgesic effects. - Causes minimal skeletal muscle relaxa&on. - No effect on &ssue PO2 measurements but slightly increases P50. Clinical Applica&ons and Side Effects: - Acute analgesic ac&vity and may aaenuate hyperacute hyperalgesia by remifentanil. - Efficacy in reducing chronic pain. - Adverse effects include high-volume absorp&on in gas-containing spaces and inac&va&on of vitamin B12. - Impact on postopera&ve nausea and vomi&ng (PONV) is debated. Postopera&ve Nausea and Vomi&ng (PONV): - Associated with increased incidence of PONV in some anesthe&c techniques. - Meta-analysis suggests lower risk of PONV when avoiding nitrous oxide. 2 - Alterna&ves and comparison of risks with vola&le anesthe&cs are considered. IMPACT trial: Highlighted clinical context of PONV, showing reduced incidence with propofol alone compared to combina&ons involving nitrous oxide. Halothane in Anesthesia: Physical Proper&es and Administra&on: - Halothane is a halogenated alkane deriva&ve. - Appears as a clear, nonflammable liquid at room temperature. - Its vapor has a sweet, bland odor. - Administered either alone or in combina&on with nitrous oxide or injected drugs like opioids. Solubility, Potency, and Anesthe&c Characteris&cs: - Intermediate solubility in blood and high potency. - Allows for intermediate onset and recovery &mes from anesthesia. - Developed for its intermediate blood solubility, anesthe&c potency, and molecular stability. - Chemical Structure and Stability: - The carbon-fluorine bond decreases flammability. - Trifluorocarbon contributes to molecular stability. - The presence of carbon-chlorine, carbon-bromine bonds, and a hydrogen atom ensures anesthe&c potency. - Suscep&ble to decomposi&on into harmful substances like hydrochloric acid, hydrobromic acid, and phosgene. 3 - Stored in amber-colored boales with thymol as a preserva&ve to prevent spontaneous oxida&ve decomposi&on. Thymol residue can cause malfunc&on in vaporizer turns&les or temperature-compensa&ng devices. Enflurane in Anesthesia: Usage and Physical Proper&es: - Enflurane is no longer commonly used in the United States. - It is a halogenated methyl ethyl ether. - Appears as a clear, nonflammable vola&le liquid at room temperature. - Has a pungent, ethereal odor. Solubility, Potency, and Anesthe&c Characteris&cs: - Intermediate solubility in blood and high potency. - Permits intermediate onset and recovery from anesthesia. - Can be used alone or in combina&on with nitrous oxide or opioids. - Clinical Effects and Considera&ons: - Decreases the seizure threshold, making it useful in specific procedures. - Oxidized in the liver, producing inorganic fluoride ions, which can be nephrotoxic. - Was primarily used in procedures favoring a low threshold for seizure genera&on, like electroconvulsive therapy. Isoflurane in Anesthesia: Physical Proper&es and Administra&on: - Isoflurane is a halogenated methyl ethyl ether. - Exists as a clear, nonflammable liquid at room temperature. - Has a pungent, ethereal odor. - Used alone or in combina&on with nitrous oxide or opioids. Solubility, Potency, and Anesthe&c Characteris&cs: - Intermediate solubility in blood and high potency. - Allows for intermediate onset and recovery from anesthesia. Stability and Storage: - Characterized by extreme physical stability. - No detectable deteriora&on over 5 years of storage. - Stable when exposed to carbon dioxide absorbents or sunlight. - No need for preserva&ves like thymol in its commercial prepara&on Desflurane in Anesthesia: Chemical Composi&on and Proper&es: - Desflurane is a fluorinated methyl ethyl ether. - Differs from isoflurane by subs&tu&ng a fluorine atom for the chlorine atom in isoflurane. - Fluorina&on increases vapor pressure, enhances molecular stability, and decreases potency. 4 - Vapor pressure is three &mes that of isoflurane, requiring specialized heated and pressurized vaporizers. Metabolism and Potency: - Minimal metabolism, with low concentra&ons of serum and urinary trifluoroacetate. - About fivefold less potent than isoflurane, as indicated by MAC values. Clinical Use and Considera&ons: - Pungent odor makes inhala&on induc&on unpleasant, unlike halothane and sevoflurane. - Can cause airway irrita&on, saliva&on, breath holding, coughing, or laryngospasm in awake pa&ents at >6% inspired concentra&ons. - Produces the highest carbon monoxide concentra&ons among inhaled anesthe&cs when degraded by desiccated carbon dioxide absorbents. Intraopera&ve Diagnosis of Carbon Monoxide Poisoning and Desflurane Characteris&cs: Detec&on Difficul&es: - Pulse oximetry can't differen&ate between carboxyhemoglobin and oxyhemoglobin. - Moderately decreased pulse oximetry readings despite adequate arterial oxygen may suggest carbon monoxide exposure. - No rou&ne means to iden&fy carbon monoxide in the breathing circuit or detect desiccated carbon dioxide absorbent. Warning Signs and Gas Analyzer Readings: - Erroneous gas analyzer readings may indicate carbon monoxide forma&on. - Trifluoromethane, produced with carbon monoxide from isoflurane, enflurane, and desflurane degrada&on, causes false enflurane readings. - This erroneous reading does not occur with sevoflurane. Measurement of Carboxyhemoglobin: - Carboxyhemoglobin can be acutely measured with CO-oximetry, which is rou&nely available. - Delayed Effects of Carbon Monoxide Poisoning: - Cogni&ve defects, personality changes, gait disturbances may occur 3 to 21 days post-anesthesia. - Intraopera&ve hemolysis can mimic carbon monoxide produc&on from vola&le anesthe&c degrada&on. Desflurane Solubility and Potency: - Blood:gas par&&on coefficient is 0.45, with a MAC of 6.6%. - Allows rapid achievement of alveolar par&al pressure for anesthesia and prompt awakening upon discon&nua&on. - Lower blood-gas solubility and more precise control dis&nguish desflurane (and sevoflurane) from earlier anesthe&cs. Sevoflurane in Anesthesia: Chemical Composi&on and Vapor Pressure: - Sevoflurane is a fluorinated methyl isopropyl ether. - Vapor pressure similar to halothane and isoflurane, allowing use with conven&onal unheated vaporizers. Solubility and Anesthe&c Proper&es: - Blood: gas par&&on coefficient of 0.69, similar to desflurane. 5 - Ensures prompt induc&on and recovery from anesthesia. - Faster recovery compared to isoflurane, especially in longer surgeries > 3 hours. Odor, Bronchodila&on, and Airway Irrita&on: - Nonpungent with minimal odor. - Produces bronchodila&on similar to isoflurane. - Causes the least airway irrita&on among current vola&le anesthe&cs. - Suitable for inhala&on induc&on like halothane. Metabolism and Biodegrada&on: - More prone to metabolism than desflurane, with 3-5% undergoing biodegrada&on. - Metabolites include inorganic fluoride and hexafluoroisopropanol. - Does not form trifluoroacetylated liver proteins, reducing the risk of hepatotoxicity and cross-sensi&vity. - Least likely to form carbon monoxide when exposed to carbon dioxide absorbents. Degrada&on Products and Nephrotoxicity: - Forms toxic compounds (including compound A) in the presence of carbon dioxide absorbent bases. - Compound A is nephrotoxic in animal studies. - Levels of these compounds in pa&ents are below speculated toxic levels, even at low total gas flows. Xenon in Anesthesia: Ideal Anesthe&c Characteris&cs: - Inert gas with proper&es ideal for an inhaled anesthe&c. - MAC of 63% to 71%, more potent than nitrous oxide (MAC 104%). - Gender-dependent MAC, lower in females. - Nonexplosive, odorless, chemically inert, and environmentally friendly. Cost and Applica&on Limita&ons: - High cost limits its widespread use in anesthesia. - Poten&al offset by low fresh gas flow rates and xenon-recycling systems. - Acceptance depends on demonstra&ng unique benefits or significantly lower morbidity and mortality compared to current anesthe&cs. Pharmacokine&cs and Effects: - Extremely low blood:gas par&&on coefficient (0.115), allowing rapid onset and offset. - Minimal effect on bowel gas exchange, less than nitrous oxide. - Does not trigger malignant hyperthermia. - Rapid emergence from anesthesia, 2-3 &mes faster than nitrous oxide plus isoflurane or sevoflurane. - Potent hypno&c and analgesic, suppressing hemodynamic and catecholamine responses. - Does not produce hemodynamic depression in healthy adults. Interac&ons and Unique Proper&es: - Neuromuscular-blocking effects of rocuronium similar during propofol and xenon anesthesia. - No observed risk of recall in small pa&ent groups. - Antagonist effects at NMDA receptors without psychotomime&c behavioral changes. - Neuroprotec&on without dopamine release s&mula&on, unlike ketamine and nitrous oxide. 6 Pharmacokine&cs of Inhaled Anesthe&cs: Absorp&on, Distribu&on, Metabolism, and Elimina&on: - Inhaled anesthe&cs are absorbed from alveoli into pulmonary capillary blood. - Distributed throughout the body, metabolized minimally, and mainly eliminated via the lungs. Age-Related Pharmacokine&c Changes: - Aging affects pharmacokine&cs due to changes in body composi&on. - Decreased lean body mass and increased body fat alter distribu&on volumes. - Smaller plasma volume but larger apparent steady-state volume for fat-soluble anesthe&cs in the elderly. - Impaired pulmonary gas exchange and reduced cardiac output can affect anesthe&c clearance and &ssue perfusion. Par&al Pressure Gradients and Anesthe&c Delivery: - Series of par&al pressure gradients from anesthe&c machine to CNS. - Goal: Constant and op&mal brain par&al pressure of inhaled anesthe&c. - Tissues, including the brain, equilibrate with arterial blood par&al pressures (Pa) of anesthe&cs. - Alveolar par&al pressures (PA) of anesthe&cs mirror brain par&al pressure (PBRAIN) at steady state. Control and Monitoring of Anesthe&c Depth: - PA used as an index for depth of anesthesia, recovery, and anesthe&c potency (MAC). - Equilibra&on means equal par&al pressures, not concentra&ons, in biophases. - Understanding PA-PBRAIN rela&onship allows control of anesthe&c depth. - Vola&le anesthe&cs' minimal metabolism and lung excre&on facilitate dosing control. - "Online" readout of end-&dal par&al pressure aids in vola&le anesthe&c dosing, offering an advantage over intravenous anesthe&c dosing. Determinants of Alveolar Par&al Pressure in Anesthesia: Alveolar Par&al Pressure (PA) and Brain Par&al Pressure (PBRAIN): - Determined by the balance of input (delivery) and uptake (loss) of inhaled anesthe&cs. - PA influences PBRAIN, affec&ng anesthe&c depth and effects. Input Factors into Alveoli: - Inhaled Par&al Pressure (PI): Concentra&on of anesthe&c in the inhaled gas mixture. 7 - Alveolar Ven&la&on: Rate and volume of gas exchange in the lungs. - Characteris&cs of Anesthe&c Breathing System: Efficiency and design of the system delivering the anesthe&c. Uptake Factors from Alveoli into Blood: - Solubility of Anesthe&c in Body Tissues: Higher solubility leads to greater uptake and slower equilibra&on. - Cardiac Output: Higher cardiac output increases distribu&on of anesthe&c in the body, affec&ng uptake. - Alveolar-to-Venous Par&al Pressure Differences (A-vD): Gradient between alveolar gas and venous blood concentra&ons. Inhaled Par&al Pressure and Concentra&on Effect in Anesthesia: Role of Inhaled Par&al Pressure (PI): - High PI is needed ini&ally from the anesthe&c machine for rapid induc&on. - High ini&al PI offsets uptake impact, accelera&ng anesthesia induc&on. - As blood uptake decreases over &me, PI should be reduced to maintain op&mal PBRAIN. - Constant PI over &me would lead to progressively increasing PA and PBRAIN as uptake diminishes. Concentra&on Effect: - Refers to how PI impacts the rate of rise of the PA of an inhaled anesthe&c. - Higher PI leads to more rapid equaliza&on of PA to PI. - High PI compensates for uptake, speeding up the increase in PA. Mechanisms Behind the Concentra&on Effect: - Concentra&ng Effect: Concentra&on of inhaled anesthe&c in a reduced lung volume due to uptake of gases. - Augmenta&on of Tracheal Inflow: Increased anesthe&c input to fill the space created by gas uptake. 8 Second-Gas Effect in Anesthesia: Defini&on and Mechanism: - The second-gas effect describes how the rapid uptake of a high-volume primary gas (first gas) enhances the uptake of a concurrently administered secondary gas (second gas). - Example: Large-volume uptake of nitrous oxide (first gas) accelerates the uptake of oxygen and vola&le anesthe&cs (second gases). Implica&ons for Gas Uptake and Lung Volume: - Increased uptake of the second gas occurs due to: - Increased tracheal inflow of both first and second gases. - Higher concentra&on of the second gas in a reduced lung volume. - This is a result of the high-volume uptake of the first gas. Compensatory Changes in Ven&la&on: - Loss of lung volume may be compensated by changes in ven&la&on: - Decreased expired ven&la&on. - Increased inspired ven&la&on (increased tracheal inflow). - The no&on that extra gas is drawn into the lungs to offset lung volume loss is nuanced, including both reduced expired ven&la&on and a decrease in lung volume. Alveolar Ven&la&on and Its Impact on Inhaled Anesthe&cs: Increased Alveolar Ven&la&on: - Enhances input of anesthe&cs, accelera&ng induc&on by increasing the rate at which PA approaches PI. - Decreased PaCO2 from hyperven&la&on lowers cerebral blood flow (CBF), poten&ally offsemng increased anesthe&c delivery to the brain. Decreased Alveolar Ven&la&on: - Reduces input, slowing the establishment of PA and PBRAIN necessary for anesthesia induc&on. - The higher the alveolar ven&la&on to func&onal residual capacity (FRC) ra&o, the quicker the increase in PA. - Faster induc&on in neonates due to a higher metabolic rate compared to adults. Spontaneous Versus Mechanical Ven&la&on: - Inhaled anesthe&cs decrease alveolar ven&la&on, crea&ng nega&ve feedback to prevent excessive anesthesia depth. - Mechanism is lost with mechanical ven&la&on, poten&ally increasing anesthesia depth with more bloodsoluble anesthe&cs. Impact of Solubility on Alveolar Ven&la&on: - Changes in alveolar ven&la&on affect soluble anesthe&cs (halothane, isoflurane) more than poorly soluble ones (nitrous oxide, desflurane, sevoflurane). - Poorly soluble anesthe&cs like nitrous oxide have rapid PA increase regardless of alveolar ven&la&on due to limited uptake. - More soluble anesthe&cs have larger uptake; increased alveolar ven&la&on speeds up PA increase. 9 Anesthe&c Breathing System: Influence on Alveolar Par&al Pressure (PA): 1. The rate of increase of PA is affected by the anesthe&c breathing system's characteris&cs. Key Factors of the Breathing System: 2. Volume of External Breathing System: - Acts as a buffer, slowing the achievement of the desired PA. 3. Solubility of Anesthe&cs in Breathing System Components: - Ini&ally slows the increase of PA. - Materials like rubber or plas&c in the system can absorb anesthe&c gases. 4. Gas Inflow from Anesthe&c Machine: - High gas inflow rates (5-10 L/min) can counteract the buffering effect of the system's volume. 5. Impact During and A\er Anesthe&c Administra&on: - At the start, solubility in the system's components delays PA increase. - A\er anesthe&c administra&on, a reverse gradient causes elu&on of the anesthe&c, slowing the decrease of PA. Solubility of Inhaled Anesthe&cs: Par&&on Coefficient: - Reflects how an inhaled anesthe&c distributes between two phases at equilibrium. - A ra&o describing the concentra&on of an anesthe&c in two different media when their par&al pressures are equal. - Blood:gas and brain:blood par&&on coefficients are key measurements. - Examples of Par&&on Coefficients: Blood:Gas Par&&on Coefficient: - A coefficient of 0.5 indicates the concentra&on in blood is half that in alveolar gases at equal par&al pressures. Brain:Blood Par&&on Coefficient: - A coefficient of 2 signifies the concentra&on in the brain is double that in the blood at iden&cal par&al pressures. Interpreta&on and Temperature Dependence: - Par&&on coefficients show the capacity of each phase (e.g., blood, brain, gas) to hold anesthe&c. - These coefficients are temperature-dependent, with solubility of gases in liquids decreasing as the liquid's temperature rises. 10 Blood:Gas Par&&on Coefficients of Inhaled Anesthe&cs: Influence on Alveolar Par&al Pressure (PA): - Rate of PA increase towards the inhaled par&al pressure (PI) is inversely related to the anesthe&c's solubility in blood. - Anesthe&cs are tradi&onally categorized based on their blood:gas par&&on coefficients: soluble, intermediately soluble, and poorly soluble. Effect of High Blood Solubility: - Large amounts of anesthe&c must dissolve in blood before Pa equilibrates with PA. - High blood solubility (e.g., methoxyflurane) slows PA and Pa increase rela&ve to PI, leading to slow anesthesia induc&on. - Overpressure technique (increasing PI above maintenance level) can offset this slow induc&on but risks anesthe&c overdose. Effect of Low Blood Solubility: - Minimal anesthe&c dissolu&on needed before equilibra&on, results in rapid PA and Pa increase, and fast onset of anesthesia. - Nitrous oxide, desflurane, and sevoflurane demonstrate this with rapid PA rise a\er about 10 minutes of constant PI inhala&on. - Overpressure with sevoflurane is beaer tolerated due to its lower pungency. Nitrous Oxide Absorp&on and Unique Effects: - Rapid Pa increase associated with absorp&on of several liters of nitrous oxide. - Unique effects when administered with vola&le anesthe&cs or in air-containing cavi&es. Influence of Individual Varia&ons: - Blood:gas par&&on coefficients vary with individual water, lipid, protein content, and hematocrit. - Lower hematocrit results in decreased solubility, poten&ally leading to faster PA increase and quicker anesthesia induc&on. 11 - Inges&on of faay meals can increase solubility of vola&le anesthe&cs in blood. Age-Related Solubility Varia&ons: - Blood solubili&es of halothane, enflurane, methoxyflurane, and isoflurane are lower in neonates and the elderly. - Solubility of less soluble anesthe&cs like sevoflurane does not differ significantly between neonates and adults. Tissue:Blood Par&&on Coefficients of Inhaled Anesthe&cs: Role in Anesthe&c Uptake and Tissue Equilibra&on: - Determine the uptake of anesthe&c into &ssues and the &me needed for &ssues to equilibrate with arterial par&al pressure (Pa). - Equilibra&on &me can be es&mated using &me constants, calculated based on anesthe&c amount dissolved in &ssue divided by &ssue blood flow. Time Constants and Equilibra&on: - One &me constant represents 63% equilibra&on. - Three-&me constants equal 95% equilibra&on. - For vola&le anesthe&cs, equilibra&on between Pa and brain par&al pressure (PBRAIN) typically takes 5 to 15 minutes (three-&me constants). Fat Tissue Considera&ons: - Fat has a high capacity to absorb anesthe&c and receives low blood flow, extending the &me to narrow anesthe&c par&al pressure differences between arterial blood and fat. - Equilibra&on of fat with isoflurane, for example, is es&mated to be 25 to 46 hours (three-&me constants). Impact of Fas&ng on Anesthe&c Uptake: - Fas&ng before surgery leads to fat transport to the liver, poten&ally increasing anesthe&c uptake in the liver. - This could modestly slow the rate of increase in alveolar par&al pressure (PA) of a vola&le anesthe&c during induc&on. Oil:Gas Par&&on Coefficients and Anesthe&c Requirements: Correla&on with Anesthe&c Potency: - Oil:gas par&&on coefficients are closely related to the potency of inhaled anesthe&cs. - These coefficients indicate how an anesthe&c distributes between oil and gas phases at equilibrium. Es&ma&ng MAC (Minimum Alveolar Concentra&on): - MAC can be es&mated using the formula: MAC = 150 / Oil:Gas Par&&on Coefficient. - The constant 150 represents the average product of oil:gas solubility and MAC for various anesthe&cs with different lipid solubili&es. Example Calcula&on: - For a hypothe&cal anesthe&c with an oil:gas par&&on coefficient of 100, the calculated MAC would be 1.5% (150 / 100 = 1.5%). 12 Nitrous Oxide Transfer to Closed Gas Spaces: Accumula&on in Closed Spaces: - Nitrous oxide can accumulate in closed air-filled spaces, causing increased pressure and poten&al damage. Differen&al Solubility with Nitrogen: - Blood:gas par&&on coefficient of nitrous oxide is 0.46, much higher than nitrogen's 0.014. - Nitrous oxide enters air-filled cavi&es faster than nitrogen exits, leading to increased volume or pressure in these spaces. Effects on Various Body Cavi&es: - Compliant walls (e.g., intes&nes, pneumothorax, pulmonary blebs): Nitrous oxide causes expansion of the gas space. - Noncompliant walls (e.g., middle ear, cerebral ventricles): Results in increased intracavitary pressure. Factors Influencing Volume or Pressure Increase: - Nitrous Oxide Par&al Pressure: Higher pressure increases transfer rate. - Blood Flow to the Cavity: Higher flow speeds up the process. - Dura&on of Nitrous Oxide Administra&on: Longer exposure leads to greater effects. Specific Cases and Implica&ons: - Pneumothorax: Rapid volume increase in animal models. - Air emboli: Rapid expansion when exposed to nitrous oxide. - Neurosurgical pa&ents: Discon&nuing nitrous oxide upon detec&on of venous air embolism can mi&gate effects. - Bowel gas: Slow increase in volume can lead to postopera&ve disten&on and pain. - Middle ear: Increased pressure due to rapid nitrous oxide diffusion, poten&ally causing tympanic membrane rupture or serous o&&s. - Intraocular gas bubbles: Brief exposure to nitrous oxide can significantly increase gas volume, risking re&nal artery compression and visual loss. Cardiopulmonary Bypass and Blood-Gas Solubility: Impact on Blood-Gas Solubility: - Cardiopulmonary bypass (CPB) leads to changes in blood-gas solubility. - These changes are influenced by the cons&tuents of the priming solu&on and the temperature during CPB. Effects of Hypothermic CPB and Crystalloid Prime: - The overall effect on blood-gas solubility is rela&vely small, around 2%. - Hypothermia and crystalloid prime are common aspects of CPB procedures. Implica&ons for Vola&le Anesthe&cs: - Vola&le anesthe&cs introduced during CPB have a prolonged equilibra&on &me. - Anesthe&cs present before the ini&a&on of CPB are diluted, which may reduce the depth of anesthesia. 13 Cardiac Output and Its Effect on Inhaled Anesthe&cs: Influence of Cardiac Output on Uptake and Alveolar Par&al Pressure (PA): - Cardiac output affects anesthe&c uptake from the alveoli, impac&ng the PA. - Increased cardiac output leads to more rapid anesthe&c uptake, slowing the rate of PA increase and anesthesia induc&on. - Decreased cardiac output results in less anesthe&c uptake, speeding up the PA increase. Paradoxical Effects of Cardiac Output: - Higher cardiac output increases &ssue anesthe&c par&al pressures, narrowing alveolar-to-venous par&al pressure differences (A-vD). - However, the arterial par&al pressure (Pa) is lower with higher cardiac output. - Change in cardiac output is analogous to a change in solubility; higher output increases blood's capacity to hold anesthe&c. Impact on Soluble vs. Poorly Soluble Anesthe&cs: - Soluble anesthe&cs are more affected by changes in cardiac output. - Poorly soluble anesthe&cs like nitrous oxide have a rapid PA increase regardless of cardiac output changes. Effects of Vola&le Anesthe&cs on Cardiac Output: - Vola&le anesthe&cs can depress cardiac output, increasing PA and anesthe&c depth. - This can lead to a posi&ve feedback loop, further depressing cardiac func&on. - Combina&on of controlled ven&la&on and decreased cardiac output due to anesthe&c depression can cause a rapid increase in PA and excessive anesthesia depth. Distribu&on of Cardiac Output and Anesthe&c Uptake: - Increased cardiac output does not equally increase blood flow to all &ssues. - Preferen&al perfusion of vessel-rich &ssues results in a quicker PA increase. - Infants, with rela&vely greater perfusion of vessel-rich &ssues, exhibit faster PA increase towards the inhaled par&al pressure (PI). Impact of Shunts on Inhaled Anesthe&cs: Right-to-Le\ Shunt Effects: - In normal condi&ons, alveolar par&al pressure (PA) and arterial par&al pressure (Pa) of inhaled anesthe&cs are nearly iden&cal. - A right-to-le\ shunt (intracardiac or intrapulmonary) dilutes the anesthe&c in blood from ven&lated alveoli, reducing Pa and slowing anesthesia induc&on. - End-&dal concentra&on monitoring shows a gradient between PA and Pa, with PA underes&ma&ng Pa. - Similar mechanism affects PaO2, crea&ng a gradient between PA and Pa with a right-to-le\ shunt. Solubility and Shunt Impact: - The impact of a right-to-le\ shunt varies with anesthe&c solubility. - Poorly soluble anesthe&cs are more affected, as their uptake is minimal, leading to unopposed dilu&onal effects on Pa. - Soluble anesthe&cs' uptake offsets dilu&onal effects of shunted blood, lessening the shunt's impact. - This effect is contrary to changes observed with cardiac output and alveolar ven&la&on altera&ons. - A right-to-le\ shunt alone is unlikely to significantly alter the speed of anesthesia induc&on. Le\-to-Right Tissue Shunts: - Arteriovenous fistulas or increases in cutaneous blood flow from vola&le anesthe&cs deliver blood with higher anesthe&c par&al pressure to the lungs. - These shunts counteract the dilu&onal effects of right-to-le\ shunts on Pa. 14 - The impact of le\-to-right shunts on Pa increase is only no&ceable with concurrent right-to-le\ shunts. - The effect of right-to-le\ shunts on PA increase is greatest in the absence of le\-to-right shunts. Alveolar-to-Venous Par&al Pressure Differences (A-vD) and Tissue Uptake of Inhaled Anesthe&cs: A-vD and Tissue Uptake: - A-vD indicates the uptake of anesthe&cs by &ssues, affec&ng lung uptake by controlling the rate of increase of mixed venous anesthe&c par&al pressure. - Determinants parallel lung uptake factors: &ssue solubility, blood flow, and arterial-to-&ssue par&al pressure differences. Vessel-Rich Group Tissues: - Comprise less than 10% of adult body mass but receive 75% of cardiac output. - Rapid equilibra&on with arterial par&al pressure (Pa) due to small mass and high blood flow. - A\er three-&me constants (5-15 minutes), venous blood from these &ssues nearly matches alveolar par&al pressure (PA). - Results in a significant decrease in vola&le anesthe&c uptake a\er this period. Muscle and Fat Tissue Uptake: - Comprise about 70% of body mass but only receive about 25% of cardiac output. - Sustained &ssue uptake of anesthe&c due to large mass. - Effluent venous blood maintains lower par&al pressure than PA, sustaining A-vD difference and lung uptake even a\er prolonged administra&on. Body Tissue Composi&on (as per Table 4.4): - Vessel-rich group: 10% body mass, 75% blood flow. - Muscle group: 50% body mass, 19% blood flow. - Fat group: 20% body mass, 6% blood flow. - Vessel-poor group: 20% body mass, less than 1% blood flow. Differences in Neonates and Infants: - Faster equilibra&on of vessel-rich group &ssues compared to adults. - Higher cardiac output to these &ssues and decreased anesthe&c solubility in neonatal &ssues. - Skeletal muscle comprises a smaller frac&on of body weight in neonates and infants. Recovery From Anesthesia: Depic&on of Recovery: 15 - Recovery is indicated by a decrease in brain par&al pressure (PBRAIN) as shown by alveolar par&al pressure (PA). - Rapid washout from the brain is expected due to low solubility of anesthe&cs in the brain and its high blood flow. Differences Between Induc&on and Recovery: - Unlike induc&on, recovery cannot be accelerated by a concentra&on effect since anesthe&c input cannot be less than zero. - At anesthesia end, &ssue anesthe&c concentra&ons are influenced by drug solubility and dura&on of administra&on, unlike at induc&on start. - Recovery rate is faster than induc&on due to incomplete &ssue equilibrium with PA during maintenance. - Tissues like skeletal muscles and fat likely haven't equilibrated with PA, ini&ally not contribu&ng to drug transfer back to blood. Tissue Uptake During Recovery: - Tissues con&nue to uptake anesthe&c during recovery, speeding the PA decrease. - This con&nued uptake depends on anesthe&c solubility and anesthesia dura&on, being more pronounced with soluble anesthe&cs. Influence of Anesthe&c Dura&on: - Recovery &me is longer with prolonged administra&on of soluble anesthe&cs (halothane, isoflurane). - Poorly soluble anesthe&cs (sevoflurane, desflurane) show minimal impact of administra&on dura&on on recovery &me. Context-Sensi&ve Half-Time: - The differences in recovery &mes based on anesthe&c solubility and dura&on are related to the concept of context-sensi&ve half-&me. Role of Anesthe&c Breathing System: - Anesthe&c absorbed into breathing system components re-enters the gases at the end of anesthesia, slowing PA decrease. - Exhaled gases containing anesthe&c may be rebreathed unless fresh gas flow rates are increased at anesthesia conclusion. Summary of Elimina&on and Time to Recovery: - Elimina&on is faster with anesthe&cs least soluble in blood (nitrous oxide, desflurane, sevoflurane). - Dura&on of anesthesia affects recovery &me, especially with blood-soluble anesthe&cs. Context-Sensi&ve Half-Time of Inhaled Anesthe&cs: Defini&on and Dependence: - Context-sensi&ve half-&me refers to the &me required for the concentra&on of an anesthe&c to decrease by half a\er discon&nua&on. - It varies based on the dura&on of anesthe&c administra&on and its blood-gas solubility. Recovery Times for Different Anesthe&cs: - Recovery is fastest with desflurane (least soluble) and slowest with isoflurane (most soluble). - Longer anesthe&c dura&on typically results in longer recovery &mes. Detailed Insights and Clinical Implica&ons: - For short procedures (around 30 minutes), there's liale difference in recovery &me between desflurane, sevoflurane, and isoflurane. - Significant benefits of desflurane over sevoflurane are only no&ceable in longer procedures (around 2 hours). - Strategies like turning off sevoflurane early and switching to nitrous oxide can ensure rapid recovery. 16 Phase of Elimina&on and Decrement Times: - The ini&al phase of elimina&on, mainly influenced by alveolar ven&la&on, is quick (<5 minutes) for a 50% decrease in anesthe&c concentra&on. - Larger decrements (80% and 90%) show more significant differences between anesthe&cs. - Desflurane and sevoflurane have shorter 80% decrement &mes, not significantly increasing with anesthesia dura&on. - Enflurane and isoflurane have longer 80% decrement &mes, increasing a\er 60 minutes. - Desflurane’s 90% decrement &me increases only slightly with prolonged anesthesia, much less than sevoflurane, isoflurane, and enflurane. Impact of Anesthe&c Choice in Recovery: - Liale difference in recovery &me among these vola&le anesthe&cs in short, pure inhala&on techniques. - Major differences occur in the final 20% of elimina&on, especially in longer procedures. Diffusion Hypoxia and Nitrous Oxide Discon&nua&on: Occurrence and Mechanism: - Diffusion hypoxia occurs when nitrous oxide (N2O) is abruptly stopped. - Causes a reverse gradient, leading to the movement of N2O from blood to alveoli. - Impact on Alveolar and Arterial Gases: - High volume of N2O entering alveoli can dilute alveolar oxygen (PAO2), reducing arterial oxygen (PaO2). - Simultaneously, alveolar carbon dioxide (PACO2) is also diluted, diminishing the respiratory drive. Exacerba&on of Hypoxia: - Decreased respiratory s&mulus further lowers PaO2 due to the outlow of N2O into the alveoli. - Greatest outpouring of N2O into alveoli occurs within the first 1 to 5 minutes a\er stopping N2O. Preven&ve Measures: - Common prac&ce to administer oxygen at the end of anesthesia. - Helps prevent arterial hypoxemia caused by N2O dilu&on of PAO2. Pharmacodynamics of Inhaled Anesthe&cs: Minimal Alveolar Concentra&on (MAC) Defini&on of MAC: - MAC is the concentra&on at 1 atmosphere pressure that prevents movement in response to a painful s&mulus in 50% of pa&ents. - It is essen&ally the ED50 (50% effec&ve dose) for inhaled anesthe&cs. Mechanism of Ac&on: - MAC primarily reflects the effects of inhaled anesthe&cs on the spinal cord. - Lesser contribu&on from cerebral effects to immobility. - Evidence: Isoflurane's MAC increases significantly when delivered only to the brain. - Decerebra&on does not alter MAC, suppor&ng spinal cord media&on. MAC in Anesthe&c Pharmacology: - Provides a standardized measure of potency for inhaled anesthe&cs. - Used to establish uniform dosages, determine equivalent amounts for specific endpoints (like MACawake), and guide research into mechanisms of ac&on. - Exhibits minimal pharmacodynamic variability (10-15% varia&on among individuals). MAC and Other Organ Effects: 17 - Equal MACs of different anesthe&cs may have varied effects on organs like the heart and lungs. - MAC only represents one point on the dose-response curve, and these curves are not parallel among different anesthe&cs. MAC-awake and MAC-memory: - MAC-awake, the concentra&on preven&ng consciousness in 50% of pa&ents, is about half of MAC. - MAC-memory, associated with amnesia in 50% of pa&ents, is lower than MAC-awake. - Importance: ED50 is a suitable clinical endpoint, as higher rates of awareness would be unacceptable in anesthesia prac&ce. Factors that Alter Minimal Alveolar Concentra&on (MAC): Age and Body Temperature: - Age is a significant factor, with MAC decreasing about 6% per decade. - Body temperature also influences MAC. MAC Changes in Pregnancy: - Decreased by nearly 30% during pregnancy and early postpartum period. - Postopera&ve awareness is higher a\er cesarean sec&ons. - Brain concentra&on response remains unchanged in pregnancy. Gender and Gene&c Factors: - Gender does not influence MAC. - Increased MAC in women with natural red hair, linked to melanocor&n-1 receptor gene muta&ons. Anesthesia Dura&on and Cardiopulmonary Bypass: - Isoflurane MAC decreases during prolonged anesthesia and surgery. - Effects of cardiopulmonary bypass on MAC are inconsistent. S&mulus Type and MAC Values: - Different types of s&muli (e.g., tracheal intuba&on, tetanic s&mula&on) require varying MAC levels. - MAC for tracheal intuba&on is higher compared to other s&muli. Addi&vity and Synergis&c Effects: - MAC values for inhaled anesthe&cs are addi&ve. - Opioids synergis&cally decrease anesthe&c requirements for vola&le anesthe&cs. - Combina&on of inhaled anesthe&cs and intravenous anesthe&cs typically results in synergis&c effects. Dose-Response Curves: - Dose-response curves for inhaled anesthe&cs are steep. 18 - 1 MAC dose prevents movement in 50% of pa&ents; a slight increase to 1.3 MAC prevents movement in 95%. Mechanisms of Anesthe&c Ac&on: Meyer-Overton Theory (Cri&cal Volume Hypothesis) Mystery of Vola&le Anesthe&c Ac&on: - The exact mechanism of vola&le anesthe&cs, par&cularly their ability to induce unconsciousness, remains a significant unsolved mystery. - Unlike most systemically administered drugs, specific molecular binding sites for vola&le anesthe&cs are not clearly iden&fied. Lipid Solubility and Anesthe&c Potency: - Historically, there has been a presumed correla&on between lipid solubility (oil:gas par&&on coefficient) of inhaled anesthe&cs and their potency. - The theory suggests that anesthe&cs act by disrup&ng nerve membrane structure or dynamics, par&cularly lipid por&ons. Cri&cal Concentra&on in Lipid Membranes: - Disrup&on occurs when enough anesthe&c molecules dissolve in hydrophobic sites like lipid cell membranes. - This dissolu&on can distort ion channels and affect synap&c transmission. - Changes in lipid matrix may alter cell membrane protein func&ons, decreasing sodium conductance. Evidence Against Meyer-Overton Theory: - Effects of anesthe&cs on lipid bilayer fluidity are minimal and comparable to minor temperature changes. - Not all lipid-soluble substances are anesthe&cs, and some even induce convulsions. - Anesthe&cs' effec&veness might relate more to binding to protein pockets than to lipid membrane ac&on. Refinement of Lipid Theories: - Recent theories suggest specialized membrane domains around proteins are sensi&ve to anesthe&cs and crucial for membrane func&on. - The Meyer-Overton correla&on could be explained by either protein binding or lipid dissolu&on. Stereoselec&vity in Anesthe&c Ac&on: Effects on Ion Channels: - Inhaled anesthe&cs demonstrably affect ion channels that are crucial for neuronal ac&on. - These effects provide insight into the mechanism of anesthe&c ac&on. - Evidence from Stereoselec&vity: - Strongest evidence for protein binding comes from the stereoselec&vity of anesthe&cs. - Inhaled anesthe&cs exist in isomeric forms, with different effects observed for each isomer. Isoflurane's Stereoselec&ve Ac&on: - Isoflurane shows stereoselec&vity in its ac&on on neuronal channels. - The levoisomer is more potent in enhancing potassium conductance in neurons and in loss of righ&ng reflex in animals. - MAC for levoisomer of isoflurane is 60% more potent than the dextroisomer in rats. - Varied Findings in Anesthe&c Research: - Some studies have not found significant differences in effects of enan&omers of isoflurane and desflurane. - Differences in anesthe&c effects between isomers suggest receptor specificity. 19 Molecular Volume and Anesthe&c Ac&on: - Conver&ng an anesthe&c to a nonanesthe&c by increasing molecular volume, despite higher lipid solubility, indicates receptor specificity. - However, molecular shape and size offer limited insight into the anesthe&c ac&on site's structure. Poten&al Mediators of Anesthe&c Ac&on in Inhaled Anesthe&cs: Ionotropic and Metabotropic Receptors: - Ionotropic receptors (ligand-gated ion channels) directly bind neurotransmiaers, affec&ng ion flow and membrane poten&al. - Metabotropic receptors ac&vate G proteins, influencing signaling molecules like protein kinases or ion channels. Inhaled Anesthe&cs and Opioid Release: - Do not s&mulate endogenous opioid release or suppress ven&latory responses to surgical s&mula&on. - Opioids reduce MAC due to their analgesic effect, not shared with inhaled anesthe&cs. Glycine and GABAA Receptors: - Glycine receptors in the spinal cord are poten&ated by vola&le anesthe&cs, possibly media&ng immobility. - GABAA receptors are poten&ated by anesthe&cs, but their influence on MAC is minimal. Glutamate Receptors (NMDA, AMPA, Kainate): - Inhaled anesthe&cs decrease excitatory neurotransmission via glutamate receptors. - Different anesthe&cs vary in their efficacy of NMDA receptor blockade. Two-Pore Potassium Channels: - These channels maintain the cell's res&ng poten&al and are sensi&ve to vola&le anesthe&cs. - TREK and TASK channel ac&vity is poten&ated in an agent-specific manner. - TASK-3 receptors play a role in EEG theta oscilla&ons associated with anesthesia and deep sleep. Voltage-Gated Sodium Channels: - Sodium channels that modulate neurotransmiaer release are sensi&ve to anesthe&cs. - Anesthe&cs inhibit neurotransmiaer release, especially glutamate, at the presynap&c junc&on. Hyperpolariza&on-Ac&vated Cyclic Nucleo&de–Gated Channels: - These channels regulate rhythmogenicity in heart and brain. - Halothane may affect these channels in motor neurons to induce anesthe&c immobility. Mechanism of Immobility Induced by Inhaled Anesthe&cs: Role of the Spinal Cord: - Immobility caused by inhaled anesthe&cs is primarily due to their ac&ons on the spinal cord, not higher brain centers. - This is evidenced by the lack of correla&on between immobility during noxious s&mula&on and electroencephalographic ac&vity. Effects on Neurotransmiaer Receptors: - Inhaled anesthe&cs depress excitatory AMPA and NMDA receptor-mediated currents. - They also act independently of inhibitory GABAA and glycine receptor-mediated currents. - Ac&ons on two-pore potassium channels may contribute to immobility. Roles of Other Receptors: - Nico&nic acetylcholine receptors do not significantly influence anesthe&c-induced immobility at the spinal level. 20 - Although opioids and α2-adrenergic receptor s&mula&on (e.g., clonidine) decrease MAC, they are unlikely to be responsible for immobility caused by inhaled anesthe&cs. Inhaled anesthe&cs do not act via opioid receptors. Complexity of Anesthe&c Ac&ons: - No single receptor group ac&on can fully explain immobility caused by inhaled anesthe&cs. - It is improbable that immobility results from concurrent ac&ons on mul&ple receptors. Mechanism of Anesthesia-Induced Unconsciousness: Lack of Comprehensive Explana&on: - The precise mechanism by which vola&le anesthe&cs cause loss of consciousness is not fully understood. Hypnosis and Loss of Consciousness: - Studied in animals as loss of righ&ng reflex and in humans as loss of response to command. - Techniques like the spared arm technique in the presence of neuromuscular blockers are used to assess consciousness. Separate Phenomena of Anesthe&c Effects: - Loss of consciousness, amnesia, and response to noxious s&muli (immobility) are considered separate effects rather than a con&nuum of anesthe&c depth. Hierarchical Process of Clinical Anesthesia: - Sensory impulses are diminished by drugs like opioids, regional anesthesia, and ketamine. - Central ac&va&ng systems are depressed by benzodiazepines, barbiturates, propofol, etomidate, ketamine, and vola&le anesthe&cs. - Motor reflexes are depressed by vola&le anesthe&cs, propofol, etomidate, and barbiturates. Vola&le Anesthe&cs as Total Anesthe&cs: - They can provide general anesthesia alone, but their pain transmission effects are biphasic, with pronocicep&ve ac&vity at very low concentra&ons. - Inhibi&on of heteromeric nico&nic receptors occurs at low anesthe&c concentra&ons. - Presynap&c Inhibi&on of Neurotransmiaer Release: - Certain inhaled anesthe&cs inhibit synap&c transmission by inhibi&ng neurotransmiaer release. - Ac&ons may be on ion channels that regulate neurotransmiaer release probability or on the release machinery itself. - Halogenated vola&le anesthe&cs inhibit certain types of sodium channels, affec&ng neurotransmiaer release. Mechanism of Anesthesia-Induced Unconsciousness: Lack of Comprehensive Explana&on: - The precise mechanism by which vola&le anesthe&cs cause loss of consciousness is not fully understood. Hypnosis and Loss of Consciousness: - Studied in animals as loss of righ&ng reflex and in humans as loss of response to command. - Techniques like the spared arm technique in the presence of neuromuscular blockers are used to assess consciousness. Separate Phenomena of Anesthe&c Effects: - Loss of consciousness, amnesia, and response to noxious s&muli (immobility) are considered separate effects rather than a con&nuum of anesthe&c depth. 21 Hierarchical Process of Clinical Anesthesia: - Sensory impulses are diminished by drugs like opioids, regional anesthesia, and ketamine. - Central ac&va&ng systems are depressed by benzodiazepines, barbiturates, propofol, etomidate, ketamine, and vola&le anesthe&cs. - Motor reflexes are depressed by vola&le anesthe&cs, propofol, etomidate, and barbiturates. Vola&le Anesthe&cs as Total Anesthe&cs: - They can provide general anesthesia alone, but their pain transmission effects are biphasic, with pronocicep&ve ac&vity at very low concentra&ons. - Inhibi&on of heteromeric nico&nic receptors occurs at low anesthe&c concentra&ons. Presynap&c Inhibi&on of Neurotransmiaer Release: - Certain inhaled anesthe&cs inhibit synap&c transmission by inhibi&ng neurotransmiaer release. - Ac&ons may be on ion channels that regulate neurotransmiaer release probability or on the release machinery itself. - Halogenated vola&le anesthe&cs inhibit certain types of sodium channels, affec&ng neurotransmiaer release. Compara&ve Pharmacology of Gaseous Anesthe&c Drugs: Dose-Response Curves and MAC Comparisons: - Inhaled anesthe&cs show different pharmacologic effects at similar MAC percentages. - Their dose-response curves are not parallel, meaning the effects vary across different anesthe&cs even at comparable potency levels. Basis of Comparison: - Compara&ve studies o\en use data from normothermic volunteers under controlled ven&la&on to maintain normocapnia. - Responses in surgically s&mulated pa&ents with other variables may differ from those in healthy volunteers. Advantages of Desflurane and Sevoflurane: - Lower blood and &ssue solubility allows for more precise control over anesthesia induc&on and faster recovery post-discon&nua&on. - Other proper&es of these newer vola&le anesthe&cs are similar to older ones, par&cularly at ≤1 MAC concentra&ons. Central Nervous System Effects of Inhaled Anesthe&cs: Mental Impairment at Low Concentra&ons: - No detectable mental impairment in volunteers exposed to very low concentra&ons (0.16% nitrous oxide or 0.0016% halothane). - Implies that opera&ng room personnel are unlikely to experience mental impairment from inhaling trace anesthe&c concentra&ons with modern scavenging techniques. Effects on Reac&on Times: - Significant increase in reac&on &mes occurs only when higher concentra&ons (10% to 20%) of nitrous oxide are inhaled. Cerebral Metabolic Oxygen Requirements: - Decrease in parallel with reduc&ons in cerebral ac&vity due to the drug. - Drug-induced increases in cerebral blood flow (CBF) can elevate intracranial pressure (ICP) in pa&ents with space-occupying brain lesions. Compara&ve CNS Effects of Desflurane and Sevoflurane: 22 - Effects on the CNS by desflurane and sevoflurane are similar to those of older inhaled anesthe&cs. Electroencephalogram (EEG) and Vola&le Anesthe&cs: Effects at Low Concentra&ons (<0.4 MAC): - Increase in frequency and voltage on the EEG, represen&ng the “excitement stage” of anesthesia. - At approximately 0.4 MAC, there's a notable shi\ in high-voltage ac&vity from posterior to anterior brain regions. Changes in Cerebral Metabolic Requirements: - Cerebral metabolic oxygen requirements decrease abruptly at around 0.4 MAC. - These changes are likely indica&ve of the transi&on from wakefulness to unconsciousness. - Amnesia and Higher Anesthe&c Concentra&ons: - Amnesia likely occurs around 0.4 MAC. - As concentra&on approaches 1 MAC, EEG frequency decreases with increased voltage. - Isoflurane shows burst suppression on the EEG at about 1.5 MAC and electrical silence at 2 MAC. - Enflurane does not cause electrical silence; halothane only at very high concentra&ons (>3.5 MAC). Nitrous Oxide's EEG Effects: - Similar to vola&le anesthe&cs, with slower frequency and higher voltage as the dose increases or when combined with a vola&le anesthe&c. Desflurane and Sevoflurane: - Cause dose-related EEG changes similar to isoflurane. - Desflurane transi&ons from increased frequency and lower voltage at low concentra&ons to higher voltage at anesthe&c concentra&ons. - Higher concentra&ons of desflurane lead to decreasing voltage and more periods of electrical silence, reaching an isoelectric EEG at 1.5 to 2.0 MAC. - Adding nitrous oxide to desflurane doesn't significantly change the EEG. Seizure Ac&vity Induced by Various Inhaled Anesthe&cs: Enflurane: - Can induce fast frequency, high voltage EEG changes resembling seizure ac&vity. 23 - Seizure-like EEG ac&vity and tonic-clonic muscle twitching can occur at concentra&ons >2 MAC or with significant hyperven&la&on (PaCO2 < 30 mm Hg). - Repe&&ve auditory s&muli may trigger seizures with enflurane. - Enflurane generally doesn't enhance preexis&ng seizure foci, except in specific epilepsy types. Isoflurane: - Does not evoke seizure ac&vity, even under deep anesthesia, hypocapnia, or auditory s&mula&on. - Exhibits an&convulsant proper&es, suppressing seizure ac&vity from other causes. - The higher MAC of enflurane compared to isoflurane might be to suppress CNS s&mula&on. Desflurane and Sevoflurane: - Generally, do not cause convulsive EEG ac&vity, even in deep anesthesia or hypocapnia. - Some reports of seizure ac&vity during sevoflurane anesthesia in certain individuals. - Sevoflurane can suppress convulsive ac&vity induced by other drugs like lidocaine. Nitrous Oxide: - May increase motor ac&vity with clonus and opisthotonus, even at clinical concentra&ons. - High concentra&ons in a hyperbaric semng can cause muscle rigidity and catatonic movements. - Rare cases of tonic-clonic seizure ac&vity reported in children a\er nitrous oxide administra&on. - Withdrawal seizures observed in animals and possible delirium or excitement in humans during recovery may suggest acute nitrous oxide dependence. Effects of Vola&le Anesthe&cs on Evoked Poten&als: General Impact on Evoked Poten&als: - Vola&le anesthe&cs typically reduce the amplitude and increase the latency of evoked poten&als. - This includes median nerve somatosensory evoked poten&als, visual evoked poten&als, and auditory evoked poten&als. - Reduc&on in amplitude is more pronounced than the increase in latency. Specific Anesthe&cs and Evoked Poten&als: - Under 60% nitrous oxide, adequate waveforms for cor&cal somatosensory evoked poten&als monitoring can be maintained with 0.50 to 0.75 MAC halothane and 0.5 to 1.0 MAC enflurane and isoflurane. - Desflurane at peri-MAC levels (0.5-1.5 MAC) increasingly depresses somatosensory evoked poten&als in pa&ents. - Nitrous oxide alone can also decrease the amplitude of cor&cal somatosensory evoked poten&als. Mental Func&on and Awareness Under Inhaled Anesthe&cs: MAC-awake and Response to Commands: - At MAC-awake concentra&ons, inhaled anesthe&cs typically result in loss of response to verbal commands. - MAC-awake is the anesthe&c concentra&on at which 50% of pa&ents are awake and responsive. - Effects on Mental Func&on at Lower Concentra&ons: - Subtle impacts on mental func&ons, such as learning, may occur at concentra&ons as low as 0.2 MAC. Variability in Preven&ng Awareness: - Different gaseous anesthe&cs have varying effec&veness in preven&ng awareness. - 0.4 MAC of isoflurane can prevent recall and response to commands. - Nitrous oxide requires higher concentra&ons (>0.5 to 0.6 MAC) for similar effects. - Influence of Surgical S&mula&on: 24 - Surgical s&mula&on can increase the anesthe&c requirement to prevent awareness during procedures. Cerebral Blood Flow (CBF) and Vola&le Anesthe&cs: Dose-Dependent CBF Increase: - Vola&le anesthe&cs cause dose-dependent increases in CBF. - The balance between vasodilatory ac&ons and flow-metabolism coupling affects this increase. - Effects at >0.6 MAC During Normocapnia: - Administra&on of vola&le anesthe&cs at >0.6 MAC leads to cerebral vasodila&on, reduced cerebral vascular resistance, and increased CBF. - This increase occurs despite reduced cerebral metabolic requirements. Compara&ve Effects of Different Anesthe&cs: - Sevoflurane causes dose-dependent cerebral vasodila&on, though less than isoflurane. - Desflurane and isoflurane similarly increase CBF and preserve CO2 reac&vity. - Nitrous oxide also increases CBF but less extensively due to its limited use at <1 MAC. - Time-Dependent Changes in CBF: - CBF increase occurs quickly a\er star&ng anesthe&c administra&on. - In animals, CBF returns to baseline over &me with halothane; in humans, CBF remains elevated during extended anesthesia. Autoregula&on of CBF: - Autoregula&on in response to blood pressure changes is maintained with 1 MAC isoflurane but not with halothane. - Desflurane and sevoflurane do not significantly alter CBF autoregula&on. - CO2 reac&vity is mostly intact with 1 MAC desflurane, but higher doses may impair autoregula&on. Cerebral Protec&on and Vola&le Anesthe&cs: Effect on Neurologic Outcome: - No significant differences in neurologic outcomes based on the type of vola&le anesthe&c used. - Isoflurane and Cerebral Protec&on: - Isoflurane may provide some cerebral protec&on during transient regional cerebral ischemia, such as in caro&d endarterectomy. - This protec&on could be due to its ability to blunt necro&c processes resul&ng from cerebral ischemia. Isoflurane in Controlled Hypotension: - During controlled hypotension for procedures like cerebral aneurysm clipping, isoflurane maintains unchanged CBF while decreasing cerebral metabolic oxygen requirements. - Suggests a favorable altera&on in the global cerebral oxygen supply-demand balance with isoflurane. Intracranial Pressure (ICP) and Inhaled Anesthe&cs: ICP Increase Linked to CBF: - Inhaled anesthe&cs increase ICP in correla&on with their induced increases in cerebral blood flow (CBF). - Pa&ents with intracranial space-occupying lesions are par&cularly sensi&ve to these ICP increases. Effects of Specific Anesthe&cs: - In hypocapnic humans with intracranial masses, desflurane at <0.8 MAC does not significantly raise ICP, but at 1.1 MAC, ICP can increase by 7 mm Hg. 25 - Hyperven&la&on to reduce PaCO2 counteracts ICP increases from inhaled anesthe&cs. - Enflurane use requires cau&on; hyperven&la&on increases seizure risk, poten&ally eleva&ng CBF and ICP. - Nitrous oxide's poten&al to increase ICP is likely less than that of vola&le anesthe&cs, due to its limited dosing (<1 MAC). Cerebrospinal Fluid (CSF) Produc&on and Inhaled Anesthe&cs: Isoflurane's Effects on CSF: - Isoflurane does not change CSF produc&on but decreases resistance to its reabsorp&on. - These effects align with the minimal increases in intracranial pressure (ICP) observed with isoflurane administra&on. Nitrous Oxide and ICP Increase: - Increases in ICP during nitrous oxide administra&on are likely due to enhanced cerebral blood flow (CBF). - Nitrous oxide does not enhance CSF produc&on, separa&ng its mechanism of ICP increase from CSF dynamics. Circulatory Effects of Inhaled Anesthe&cs: Dose-Dependent and Drug-Specific Effects: - Inhaled anesthe&cs cause circulatory effects that vary in intensity based on the dose and specific drug. - Desflurane's circulatory effects resemble those of isoflurane, while sevoflurane shares characteris&cs with both isoflurane and halothane. Manifesta&ons of Drug-Induced Circulatory Effects: - Changes occur in systemic blood pressure, heart rate, cardiac output, stroke volume, right atrial pressure, systemic vascular resistance, cardiac rhythm, and coronary blood flow. Variability Based on Condi&ons: - Effects may differ based on controlled ven&la&on versus spontaneous breathing. - Preexis&ng cardiac disease can modify the circulatory response to these anesthe&cs. - Concurrent use of drugs that affect the heart can also influence the circulatory effects. Mechanisms Behind Circulatory Effects: - These effects o\en result from the anesthe&cs' impact on myocardial contrac&lity, peripheral vascular smooth muscle tone, and autonomic nervous system ac&vity. Mean Arterial Pressure and Inhaled Anesthe&cs: General Effects on Mean Arterial Press