Protein Synthesis Inhibitors: Macrolides, Lincosamides, Aminoglycosides, Streptogramins, and Oxazolidinones PDF
Document Details
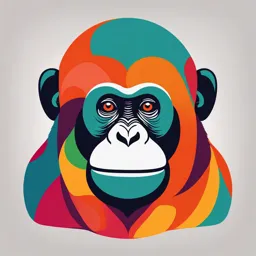
Uploaded by HappySard8182
Al-Quds University
Hussein Hallak, Ph.D.
Tags
Related
Summary
This document provides an overview of protein synthesis inhibitors, including various classes like macrolides, lincosamides, aminoglycosides, and their mechanisms of action, adverse effects, pharmacokinetics, and clinical uses. Focusing primarily on the pharmacology of these types of drugs.
Full Transcript
Protein Synthesis Inhibitors: Macrolides, Lincosamides, Aminoglycosides, Streptogramins, and Oxazolidinones Hussein Hallak, Ph.D. Al-Quds University Objectives Differentiate between the various antimicrobials that act by inhibiting protein synthesis, on the basis of:...
Protein Synthesis Inhibitors: Macrolides, Lincosamides, Aminoglycosides, Streptogramins, and Oxazolidinones Hussein Hallak, Ph.D. Al-Quds University Objectives Differentiate between the various antimicrobials that act by inhibiting protein synthesis, on the basis of: Mechanism of action Spectrum of activity and resistance mechanisms Toxicity Appropriate use Bacterial Protein Synthesis Macrolides Roxithromycin (not approved in USA) Roxithromycin has similar antimicrobial spectrum as erythromycin, but is more effective against certain gram-negative bacteria Macrolides: Mechanism of Action Inhibit protein synthesis by reversibly binding to the 50s ribosomal subunit and interfering with the elongation phase Binding stimulates dissociation of peptidyl tRNA from the ribosome Bacterial Protein Synthesis Macrolides-pharmacokinetics All oral drugs, erythomycin and azithromycin have IV preparations Absorption: must be coated to avoid destruction by gastric acid. Distribution is widespread, most tissues have higher concentrations that persist longer than in the serum Readily diffuses into intracellular fluids except CSF. Macrolides concentrate inside macrophages and neutrophils General Characteristics of Erythromycin Metabolism: concentrated in liver; Some demethylation. t½ = 1.6 hr. Elimination: Partially metabolized by the liver (N - demethylation) Partially excreted in the bile Partially excreted by the kidneys Erythromycin-drug Interactions Strong inhibitor of the P450 3A4 isoenzymes Reduced metabolism/elimination of numerous drugs -> increased drug levels Some of these interactions are life- threatening midazolam, quinidine, warfarin Erythromycin-adverse Effects ~30% Gastrointestinal Cramps, abdominal pain, N&V, diarrhea Dose related, may be reduced with food Hepatic effects, cholestatic hepatoxicity Generally considered to be a hypersensitivity reaction; but is much more common in patients with pre-existing liver dysfunction Ototoxicity Most common with high doses of parenteral erythromycin Usually reversible Macrolides: Clarithromycin More acid stable than erythromycin Longer t½ (4 hr) Adverse effects: Better tolerated than erythromycin, although GI side effects still occur Taste disturbances (more common with higher doses) Drug interaction profile similar to erythromycin Macrolides: Azithromycin More acid stable than erythromycin Longer t½ (35 – 40 hr) Adverse effects: Extraordinarily well-tolerated antibiotic N&V, headache < 1% of patients Very few drug interactions Major advantage over Erythromycin and Clarithromyin Common Properties of Clarithromycin and Azithromycin More acid stable than erythromycin. Both produce significantly less GI toxicity than erythromycin. Same mechanism of action as erythromycin; Cross- resistance with erythromycin. Azithromycin is least likely of the 3 macrolides to inhibit cytochrome P450 enzymes. Four Common Mechanisms of Antibiotic Resistance: Macrolide resistance mechanisms Decreased permeability Primary resistance among most Gram negatives Active transport out of cell Plasmid encoded efflux pumps Common in Streptococcus spp. (low level resistance) mef genes Macrolide Resistance (Continued) Target site alteration Methylation of the 23s rRNA of the 50s ribosomal subunit (MLS resistance) Chromosomally-encoded Mediated by erm (erythromycin ribosomal methylase) Methylation affects overlapping ribosomal targets Confers resistance to all macrolides, lincosamides, streptogramins (MLSB) High level resistance (MIC > 64 µg/ml) Macrolide resistance-continues Antibiotic modification Enzymatic inactivation of erythromycin by esterases or phosphotransfereases Plasmid mediated Associated with high-level resistance Erythromycin-Spectrum Gram-positive Streptococci Increasing resistance among Streptococcus pneumonia Methicillin-susceptible staphylococci Gram negative Haemophilus influenzae, Moraxella catarrhalis, Bordetella pertussis “Atypical” pathogens and other organisms Legionella, Mycoplasma pneumoniae, Chlamydia What Do Clarithromycin and Azithromycin Add to Spectrum? More potent against selected pathogens H. influenzae Helicobacter pylori Selected Mycobacteria spp. (M. avium complex, M. fortuitum, M. chelonae) Macrolides-Appropriate Use Mild to moderate upper respiratory tract infections caused by group A streptococci or S. pneumoniae Often drug of choice in patients with PCN allergy STDs (often second-line drug): Erythro or azithro Chancroid Chlamydia: azithro is only single dose therapy (half life) Coverage of atypicals in moderate to severe lower respiratory tract infections Legionella, Mycoplasma Clarithromycin and Azithromycin Mycobacterium avium complex infections Azithromycin used as prophylaxis in AIDS “Rapid grower” mycobacteria M. chelonae, M. fortuitum H. Pylori-associated peptic ulcer disease Clarithromycin can be given with metronidazole and omeprazole Ketolides: Newly introduced Modification of macrolide structure Overcomes inducible MLSB and efflux resistance Does not overcome constitutive resistance Acts by binding to the peptidyl transferase site of 23S rRNA of 50S subunit Superior to macrolides for some resistant Gram positives (e.g. S. pneumoniae) Telithromycin recently FDA approved Lincosamides Although clindamycin and lincomycin are both available, clindamycin is the most widely used product (better absorption) Binds to same site as macrolides (50S subunit) CLINDAMYCIN CLINDAMYCIN General Features. Mechanism of action: inhibition of bacterial protein synthesis by binding to 50S ribosome. Binds to a site similar to that of erythromycin. Resistance: due to decreased affinity of drug for bacterial 50S ribosome. Cross-resistance between clindamycin and erythromycin. CLINDAMYCIN Absorption: well-absorbed orally. Bioavailability ~90%. Available as PO, IV, IM, topical. Distribution: widely distributed; Does not penetrate CSF. High concentrations in bone and middle ear. Concentrates within neutrophils. Metabolism: extensively metabolized to inactive forms; T1/2 = 2.7 hr. Excretion: renal, bile; 10% excreted unchanged in urine. Clindamycin Spectrum of Activity Gram positive Good activity against methicillin-susceptible S. aureus, and streptococci Retains activity against some macrolide-resistant strains (efflux) Gram negative Essentially no activity against gram negative aerobes Anaerobes Excellent drug for Bacteroides species, Peptostreptococus Antiprotozoal activity Toxoplasma, Babesia, Pneumocystis Clindamycin-Resistance Target alteration MLS resistance as for macrolides Most common mechanism in Gram positives Drug inactivation (4-clindamycin 0- nucleotidyltransferase) Leads to high level resistance Found in Staphylococcus spp. Intrinsic resistance Gram negatives-poor penetration of drug Clindamycin Major Adverse Effects Diarrhea (10-20%). Antibiotic-associated pseudomembraneous colitis (clostridium difficile). Reported to occur between 1-10% of patients treated (may occur with any antibiotic treatment). Characterized by diarrhea (bloody), fever, cramps, yellow- white plaques on colonic mucosa. Can be fatal if not treated. Caused by a toxin produced by overgrowth of clostridium difficile. Diagnosed by antigen detection of C. difficiletoxin in stool. Rx: stop clindamycin, give metronidazole, or oral vancomycin-> avoid antiperistaltic agents. Clindamycin-Clinical uses Potential for C. difficile colitis has somewhat limited the role of clindamycin Clindamycin is considered the drug of choice for respiratory tract infections caused by anaerobes Abscesses Aspiration pneumoniae Severe Group A streptococcal infections (given in combination with PCN); soft tissue infections Osteomyelitis Given in combination with ciprofloxacin for mixed infxns Clindamycin Clinical Uses Antiprotozoal agent 2nd line regimens for selected opportunistic infections in AIDS patients (sulfa intolerant) Toxoplasmosis (clindamycin plus pyrimethamine) Pneumocystis cariniipneumonia (clindamycin plus primaquine) Babesiosis (clindamycin plus quinine) TETRACYCLINES: Doxycycline, Tetracycline, Minocycline Broad spectrum drugs Bind metal ions (eg. Ca2+, Mg2+, Al3+) Renal and hepatic elimination Adverse Reactions GI distress Nephrotoxicity Stains teeth, bones Photosensitivity Mechanism of Action The site of action of TET is the bacterial ribosome and all TET function in the same manner. They are bacteriostatic compounds. They inhibit protein synthesis by binding specifically to the 30S ribosome. This appears to prevent access of AA-tRNA to the acceptor site on the mRNA-ribosome complex; preventing the addition of AA to the growing peptide chain. These compounds also impair protein synthesis in mammalian cells at high concentration. Step 1 -Passive diffusion through hydrophilic pores in the outer cell membranes. Step 2 -Energy-dependent active transport system that pumps all TET through the inner cytoplasmic membrane. Minocyline & perhaps Doxycycline are more lipophilic than the other TET and pass directly through the lipid bilayer. Resistance Lack of active transport of Tetracyclines across bacterial cell membrane Efflux of tetracyclines from microbe Ribosomal alteration TETRACYCLINES: PK Absorption: oral absorption variable. Impaired by di- and trivalent cations. Distribution: Vd can be greater than body water. Metabolism: concentrated in liver. T½= 6 to 16 hr. Excretion: renal for most; Doxycycline, Minocycline are eliminated hepatically. Tetracyclines: Toxicity GI side effects are common Irritation of GI mucosa Deposition in teeth causes discoloration, demineralization, predisposition to decay Contraindicated during tooth development Hepatotoxicity: rare but potentially fatal Phototoxicity: rash after sun exposure Effects on TEETH Children receiving long-or short term therapy with TET may develop brown discoloration of the teeth. The drug deposits in the teeth and bones probably due to its chelating property and the formation of a TET -calcium orthophosphate complex. This discoloration is permanent. Avoid giving to pregnant women and children under the age of 8. Other Effects Hyersensitivity Rxn -rash, hives with itching, itching anaphylactic rxn (decrease in BP, increase in HR, release of histamine, etc.). Photoxicity: darkening of skin & sunburn when patient exposed to sunlight. Effects on Microbial Agents Tetracycline possess a wide range of antimicrobial activity against gram-positive and gram-negative bacteria, aerobic and anaerobic organisms. These drugs are primarily bacteriostatic. Only multiplying microorganisms are affected. Minocycline is usually the most active followed by Doxycycline then Tetracycline and Oxytetracycline (least active). Tetracyclines: Spectrum of Activity Tetracyclines have a broad spectrum of activity, but most valuable activity is against: Selected intracellular pathogens. Rickettsia, Coxiella burnetti, Chlamydia spp. Spirochetes. Borellia spp., Treponema pallidum. Mycobacterium marinum, plasmodium spp. Tetracyclines: Appropriate Use Tetracycline and Doxycycline: Rickettsial infections (RMSF, others) Chlamydia ( pneumoniae, psittici, trachomatis) Borrelia burgdorferi, B. recurrentis Lyme disease, relapsing fever Brucellosis In combination with rifampin or aminoglycoside Doxy: prophylaxis for malaria in some areas Tetracyclines: Appropriate Use Minocycline: Nocardia infections Therapy for rheumatoid arthritis Effectiveness due to antimicrobial activity? OR other non-antimicrobial effects NO production Effects on WBC chemotaxis, phagocytosis, TNF production Inhibition of phospholipase A2 Therapeutic Uses The TET has been used extensively for the treatment of infections diseases. This has resulted in bacterial resistance to these drugs. Thus the number of indications for TET has declined. TET should not be used in pregnant women and children under 8. Should not be given to patient with severe liver disease. Aminoglycosides Bind to 30S ribosomal subunit, inducing misreading of mRNA codons during translation as well as inhibiting translocation Gentamicin Tobramycin Amikacin Streptomycin Neomycin, paromomycin Aminoglycosides: Spectrum Primarily active against aerobic gram negative rods Some activity against gram positive cocci Streptococci, enterococci, staphylococci Selected agents active vs. mycobacteria Streptomycin: Mycobacteria tuberculosis Amikacin: non-tuberculous mycobacteria Aminoglycosides: Resistance Enzyme inactivation: Aminoglycoside modifying enzymes Acetyltransferases Adenyltransferases Phosphotransferases Target alteration Less common Primary resistance due to decreased uptake Aminoglycosides: Pharmacokinetics Polar cations, poorly absorbed from GI tract IV or IM preparation Tissue concentrations generally lower than serum Concentrate in kidney and middle ear Cleared by the kidneys Clearance proportional to GFR Aminoglycosides: Toxicity Nephrotoxicity Acute tubular necrosis (ATN) Usually reversible with discontinuation Ototoxicity Auditory Destruction of sensory hair cells in cochlea/ vestibular labyrinth Less common: neuromuscular blockade Aminoglycosides: Appropriate Use Serious aerobic gram-negative infections Pseudomonas aeruginosa and others Used in combination with beta-lactams Broaden spectrum of activity and may display SYNERGY for serious infections Serious enterococcal infections Used in combination combination with beta- lactams SYNERGY even with low level resistance Aminoglycosides: Nonabsorbable Neomycin Reduce bacterial burden in gut Bowel prep prior to surgery Reduce bacterial burden to tx hepatic encephalopathy Paromomycin Used as a lumenal agent for management of amebiasis and helminth infections CHLORAMPHENICOL Broad spectrum, very active drug Adverse reactions Gray baby syndrome Reversible, dose-dependent bone marrow suppression Aplastic anemia Adverse effects cont. Hematologic Toxicity The most important adverse effect of chloramphenicol is on the bone marrow. Chloramphenicol affects the hematopoietic system in two ways: by a dose-related toxic effect that presents as anemia, leukopenia, or thrombocytopenia by an non-dose-related idiosyncratic response manifested by aplastic anemia, leading in many cases to fatal pancytopenia. Reversible Bone Marrow Suppression A dose-related, toxic hematologic effect of chloramphenicol is a common and predictable (but reversible) erythroid suppression of the bone marrow. probably due to inhibitory action of the drug on mitochondrial protein synthesis. It occurs regularly when plasma concentrations are 25 mg/ml or higher and is observed with the use of large doses of chloramphenicol, prolonged treatment, or both. Reversible Bone Marrow Suppression The clinical picture is marked initially by reticulocytopenia, which occurs 5 to 7 days after the initiation of therapy, followed by a decrease in hemoglobin, an increase in plasma iron Gray Baby Syndrome Fatal chloramphenicol toxicity may develop in neonates, especially premature babies, when they are exposed to excessive doses of the drug. The illness, the gray baby syndrome, usually begins 2 to 9 days after treatment is started. The manifestations in the first 24 hours are vomiting, refusal to suck, irregular and rapid respiration, abdominal distention, periods of cyanosis, and passage of loose, green stools. Soon they turn to ashen-gray color, and become hypothermic Gray Baby Syndrome Cont Two mechanisms are apparently responsible for chloramphenicol toxicity in neonates (1) failure of the drug to be conjugated with glucuronic acid, owing to inadequate activity of glucuronyl transferase in the liver, which is characteristic of the first 3 to 4 weeks of life; and (2) inadequate renal excretion of unconjugated drug in the newborn. both exchange transfusion and charcoal hemoperfusion have been used to treat overdose with chloramphenicol in infants. CHLORAMPHENICOL Clinical Uses Restricted to life threatening infections. Should be used when other drugs fail if it is superior to all other alternatives. Primary indications include typhoid fever and topical treatment of eye infections. Leukocyte and differential count to monitor bone marrow function. From: The Medical Letter, and “The Antimicrobial Drugs”, Pratt and Fekety Very important The risk of aplastic anemia does not contraindicate the use of chloramphenicol in situations in which it is necessary; however, it emphasizes that the drug should never be employed in undefined situations or in diseases readily, safely, and effectively treatable with other antimicrobial agents. Quinupristin/Dalfopristin Streptogramin class antibiotic Quinupristin/Dalfopristin in a 30:70 ratio Inhibits protein synthesis at two unique steps associated with the 50s ribosome Individually bacteriostatic, bactericidal in combo Broad activity against gram-positive aerobes Very active against MRSA, VREF ( E. faecium) Quinupristin-dalfopristin: Use Infections due to the most problematic resistant gram-positive organisms Methicillin-resistant staphylococcus aureus Vancomycin-resistant Enterococcus faecium No activity against E. faecalis Use is now limited by availability of linezolid Broader spectrum Better tolerated Oral bioavailability Oxazolidinones: Linezolid Brand new class of agent Broadly active against gram positives Including MRSA, VRE Bacteriostatic against most organisms Excellent (100%) oral bioavailability Generally well tolerated thrombocytopenia with prolonged therapy Weak MAOI activity, clinical significance unclear Linezolid : Mechanism of Action Linezolid-Resistance Alteration of target site Mutations identified in 23S rRNA gene S. aureus, G2447U E. faecalis, G2576U Low spontaneous mutation frequency