PI 29 - Neurotransmission II: Neurotransmitters PDF
Document Details
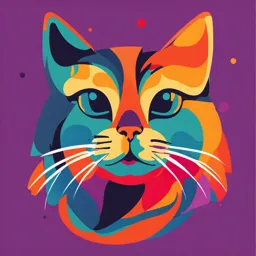
Uploaded by AffordableRhinoceros2341
Wright State University
2024
Eric Bennett
Tags
Summary
This document is pre-session material for a presentation (PI 29) on neurotransmission. It covers the topics of neurotransmitters, postsynaptic signaling, synaptic integration, and modulation. The document includes learning objectives, key concepts, and references to readings from neuroscience textbooks.
Full Transcript
PI 29 - Neurotransmission II: Neurotransmitters, postsynaptic signaling, and synaptic integration and modulation in the CNS Tuesday, November 19, 2024, 9 AM Eric Bennett Pre-session text resource – Purves, “Neuroscience”,...
PI 29 - Neurotransmission II: Neurotransmitters, postsynaptic signaling, and synaptic integration and modulation in the CNS Tuesday, November 19, 2024, 9 AM Eric Bennett Pre-session text resource – Purves, “Neuroscience”, 6th edition, Chapters 6 and 8. PDFs of these chapters are uploaded. References to pages, figures, tables, etc. from these readings will be included below. As typical for this series of PIs, there is a list of supplemental reading from two other texts at the end of the pre-session preparatory materials. Origin’s Learning Objectives: OW120 Compare and contrast fast and slow transmission, and excitatory and inhibitory transmission OW121 Describe how the motor neuron transmits signals to the neuromuscular junction to activate muscle contraction OW122 Explain how neuromuscular transmission is disrupted by neurotoxins, pharmacological blocking agents, and autoimmune disease OW132 Describe the distinguishing characteristics of neurotransmission, and identify the receptors and the associated cell signaling, for the major neurotransmitters in the central nervous system, including glutamate, ACh, GABA, neuropeptides (esp. opioids); monoamines (except histamine); cannabinoids. OW133 Explain the mechanism of action of common pharmacologic agents, including benzodiazepine, pentobarbital, serotonin reuptake inhibitors, cocaine and amphetamines OW134 Explain the roles of glutamate neurotransmission in long term plasticity and excitotoxicity Objectives: We will complete our discussion of the life cycle of neurotransmitters, from synthesis through inactivation in the chemical synaptic cleft. We will then discuss the postsynaptic events following activation of both types of neurotransmitter receptors in the CNS: ionotropic and metabotropic receptors. We will discuss how modulation of the synapse alters the signal sent by the presynaptic neuron and/or the signal received and processed by the postsynaptic cell. We will discuss in a bit more detail excitatory and inhibitory postsynaptic potential and the neurotransmitters that typically produce EPSPs and IPSPs, and how temporal and spatial summation of EPSPs and IPSPs determine the excitability of the postsynaptic cell. Finally, we will discuss synaptic integration and modulation. The following concepts are key to your understanding (many of the first 5-6 items were introduced during PI 26, neurotransmission I, but are very important for our discussion today, as several will be discussed in much more detail than we did previously as we discuss CNS neurotransmission): 1. Chemical synapses are the translation of the electrical signal in the presynaptic cell to the release of chemical messengers call neurotransmitters that bind to receptors on the postsynaptic cell ultimately altering the electrical properties of the postsynaptic cell. 2. A substance must exhibit at least three well established characteristics in order to be considered a neurotransmitter: 1) The substance must be expressed in the presynapse, 2) The substance must be released through Ca++ dependent mechanisms following stimulation of the presynaptic neuron, and 3) the postsynaptic neuron must express receptors specific for the substance. PI 29 - NT2 - Objectives and Key points – November 19, 2024 1 3. Neurochemistry – distribution and pathways for major neurotransmitter pathways in the CNS. Please 4. Neurotransmitter synthesis and packaging (Steps 1 and 2). Small molecule transmitters are made at the axon terminus and stored in clear-core vesicles. Larger, peptide transmitters are made at the cell body as propeptides, and then transported in large, dense core vesicles along the axon in preparation for release. 5. Exocytosis (Step 3). Transmitters are released into the synaptic cleft through exocytosis following an influx of Ca2+ due to activity of voltage-gated Ca2+channels that are activated by the membrane depolarization of the propagating action potential. Exocytosis is a well- orchestrated phenomenon that involves the activity of several proteins expressed in the plasma and vesicular membranes as well as in the axonal cytoplasm. 6. Receptor binding and activation (Step 4). Released transmitters then bind to receptors on the postsynaptic membrane. Receptor binding either directly activates (or inactivates) a ligand-gated ion channel, or activates (deactivates) an ion channel through a G-protein coupled process. 7. A postsynaptic potential (PSP – a change in postsynaptic membrane potential localized to the postsynaptic membrane) will be produced following activation of the postsynaptic receptor(s). This response can be across many timelines, from the fastest (activation of an ionotropic receptor channel) to the slowest (likely activation of a G protein coupled peptide NT receptor). Examples of PSP following receptor activation – Purves, chapter 6, figs. 6.6, 6.8., 6.11, and 6.24, with accompanying reading. Timeline of PSP onset/response depending on the receptor-type activated can be found in supplemental reading, Boron and Boulpaep, Fig. 13.2. 8. Transmitter inactivation and/or removal (Step 5). Transmitters are removed from the synaptic cleft through various processes, thus terminating transmission. 9. Synthesis, storage, and degradation of various neurotransmitters. Important Purves table summarizing all NT – Table 6.1. Important Purves, chapter 6 figures and accompanying reading – small molecule NT - 6.1, 6.2, 6.5, 6.10, 6.14, and 6.18; peptide NT - 6.21, and 6.22, Table 6.2; NO – Fig. 6.25 10. Neurotransmitters can bind to one of two receptor types: ionotropic (ligand-gated ion channel) or metabotropic (G-protein coupled receptor). The changes in postsynaptic membrane permeabilities resulting from receptor activation is typically much faster for ionotropic than for metabotropic receptors because metabotropic receptors must activate a multi-step intracellular biochemical cascade prior to ion channel activation (deactivation). Overview, Purves chapter 6 Figures 6.3 and 6.4 with accompanying reading 11. Metabotropic receptor structure – a 7 transmembrane protein with a transmitter-binding pocket in the center of the extracellular portions of the receptor. G-proteins interact with the receptor on the cytoplasmic face of the receptor. 12. Biochemical events following metabotropic receptor activation, and examples of different second messenger pathways used in metabotropic receptor signaling. 13. Neurotransmitters and their receptors. Modulation of signal received through receptor diversification. Details of receptor structures/functions can be found in Purves chapter 6, Figs. 7-9, 11-13, 16, 19, and 20, with accompanying reading. PI 29 - NT2 - Objectives and Key points – November 19, 2024 2 14. Excitatory postsynaptic potentials (EPSPs) and inhibitory postsynaptic potentials (IPSPs). 15. Release of more than one neurotransmitter by a single presynaptic neuron. Modulation of signal received through activation of multiple neurotransmitter receptors. 16. Convergence and divergence. Several neurons may synapse onto a single neuron. This is called convergence of input. Conversely, a single neuron may synapse onto several neurons through the divergence of output. 17. Temporal and spatial summation. Temporal summation of PSPs is the additive effect on the membrane potential of PSPs that fire nearly coincidentally. Spatial summation is the additive effect on the membrane potential of PSPs that fire in near proximity to one another. The total change in membrane potential (Grand postsynaptic potential) is dependent on the combined effects of both temporal and spatial summation. 18. Long-term potentiation (LTP) – please read the LTP section in Purves, Chapter 8. Two of these figures (8.7 and 8.10) are included in the note section below. 19. Presynaptic modulation – inhibition and facilitation. Presynaptic inhibition is the inhibition of axon A that synapses onto neuron B through presynaptic inhibition from neuron C. That is, C causes A to release less neurotransmitter, thus causing fewer PSPs in B. Conversely, presynaptic facilitation is the enhancement of neurotransmitter release by A from the excitatory effects of C pre-synaptically synapsing on A, causing an increase in PSPs in B. PI 29 - NT2 - Objectives and Key points – November 19, 2024 3 Chemical synapse Release of neurotransmitter propagates signal Types of neurotransmitters Small molecule transmitters Three main types: 1) Acetylcholine 2) Amino Acids 3) Biogenic Amines a) Catecholamines b) Indolamines c) Imidazolamines 4) Purines Nitric Oxide (NO) Atypical neurotransmitter PI 29 - Neurotransmission 2 - Nov. 19, 2024 1 Peptide neurotransmitters Synthesis of neurotransmitters Small molecular NT synthesis/packaging: 1. Enzymes and possibly NT precursors transported to axon terminus (AT) through slow transport 2. Precursors also transported into AT from extracellular space 3. NT produced and packaged at AT space Peptide NT synthesis/packaging: 1. Translated as pre-pro-peptide in ER 2. Processed in Golgi as pro-peptide 3. Pro-peptide and protease-containing vesicles bud off Golgi 4. Transported through fast axonal transport to axon terminus 5. Fast transport – protein motor proteins (e.g., kinesin) and ATP hydrolysis to rapidly move vesicles along axonal microtubules 6. Pro-peptide degraded to active NT within vesicle PI 29 - Neurotransmission 2 - Nov. 19, 2024 2 Packaging of neurotransmitter - Formation of synaptic vesicles Dense-core vesicles: - Larger, peptide transmitters are synthesized Clear-core vesicles: within the cell body, with the propeptide and - Typically, small molecule transmitters enzymes packaged together in large are synthesized at the presynaptic nerve dense-core vesicles that bud from the terminal. Transmitters are stored in Golgi apparatus. These vesicles are then endosomes which then bud off small, transported along the axon to the terminus clear-core vesicles. as described. 3) Recovering vesicular membrane - endocytosis The life cycle of a synaptic vesicle PI 29 - Neurotransmission 2 - Nov. 19, 2024 3 Proteins involved in exocytosis - a second view 4) Released neurotransmitters bind to postsynaptic receptors, and alter postsynaptic ion channel activity PI 29 - Neurotransmission 2 - Nov. 19, 2024 4 Two mechanisms of transmitter binding Direct gating of channels, or gating through G-protein-coupled receptors Receptor is a ligand-gated channel Receptor is coupled to a G-protein Many neurotransmitters bind to Transmitter binds to a G-protein receptors that are also ion channels. receptor that activates a G-protein The channel activates after a responsible for some type of sufficient number of transmitter intracellular messaging that molecules are bound. results in channel activation. 5) Inactivation (removal) of neurotransmitter - The postsynaptic receptors will be active until the bound neurotransmitter is removed. - Thus, methods of transmitter inactivation and/or removal must exist in order to terminate synaptic transmission. - Three methods are known to terminate transmission: 1) Cleaving the transmitter into inactive constituents through enzyme activity. Acetylcholine is broken down into acetate and choline through cholinesterase activity in the synaptic cleft. 2) Diffusion of the transmitter from the synaptic space. 3) Reuptake of transmitter back into the presynaptic neuron through activity of neurotransmitter transporters. PI 29 - Neurotransmission 2 - Nov. 19, 2024 5 Approximate time frame for exocytosis Putative frequency dependent release of small versus peptide transmitters PI 29 - Neurotransmission 2 - Nov. 19, 2024 6 Synthesis and degradation of Acetylcholine Synthesis: Acetylcholine (ACh) is synthesized through the activity of choline acetyl- transferase, combining Acetyl-CoA with Choline. Storage: ACh is stored in vesicles, and released into the synaptic cleft through exocytosis in response to an action potential. Termination: An enzyme in the synaptic cleft called acetylcholinesterase degrades ACh into its inactive precursors. Choline is taken back into the presynaptic cell via a transporter, and ACh is again synthesized and packaged for a subsequent transmission. Biogenic Amines - Dopamine, Norepinephrine, and Serotonin Produced from aromatic amino acids, stored in vesicles, released through exocytosis, and taken back into the presynapse through activity of specific transport proteins. Synthesis : - Dopamine and Norepinephrine: The catecholamines are produce from the precursor tyrosine through a series of well characterized enzymatic events. - Serotonin (5-HT): Serotonin is produced from the precursor, tryptophan. Storage: - All three biogenic amines are stored in synaptic vesicles. Following synthesis, the transmitter is taken up into vesicles through activity of the vesicular monoamine transporter. It appears that an identical transport protein is responsible for the vesicular uptake of all three biogenic amine neurotransmitters. Termination: The major pathway for transmission termination of all three biogenic amines is through activity of a transporter expressed in the presynaptic plasma membrane that is specific for that amine. PI 29 - Neurotransmission 2 - Nov. 19, 2024 7 Catecholamine synthesis pathway Tyrosine hydroxylase (rate limiting step) DOPA decarboxylase (aka L-Aromatic Amino Acid Decarboxylase – AAAD) Dopamine Beta-hydroxylase Phenylethanolamine-N-methyltransferase (PNMT) Catechol-O-methyltransferase (COMT) is the other enzyme besides MAO that breaks down catecholamines. COMT is cytosolic and MAO is mitochondrial. Distribution of catecholamines PI 29 - Neurotransmission 2 - Nov. 19, 2024 8 Distribution of other biogenic amines serotonin and histamine Glutamate as a transmitter - Synthesis: Glutamate is synthesized in the presynaptic terminus from glutamine through activity of glutaminase. Also, glutamate is a product of the Kreb’s cycle through transamination of a-ketoglutarate. - Termination: 1) Glutamate is directly taken up into the presynapse through glutamate transporter activity. These transporters are called, excitatory amino acid transporters, or EAAT. 2) Glutamate is also taken up into the neighboring glial cell forming glutamine through glutamine synthetase activity. The newly synthesized glutamine is then released from the glia through transporter activity, and then taken into the presynapse through the activity of a second glutamine transporter. PI 29 - Neurotransmission 2 - Nov. 19, 2024 9 GABA as a transmitter Synthesis: GABA is synthesized from glutamate through the activity of glutamic acid decarboxylase (GAD) and the cofactor, pyridoxal phosphate. Termination: GABA is transported both into glia and into the presynapse through GABA transporters (GAT). GABA is then converted to succinate which enters the Kreb’s cycle. Glycine as a neurotransmitter Synthesis: Glycine is synthesized from Serine. Termination: Glycine is transported both into glia and into the presynapse through Glycine transporters. PI 29 - Neurotransmission 2 - Nov. 19, 2024 10 Neurotransmitter receptor activation typically causes a change in membrane potential 1. Presynaptic neuron is stimulated 2. Released neurotransmitter binds to postsynaptic receptor 3. Receptor activation leads to change in postsynaptic membrane potential – a PSP is produced. PI 29 - Neurotransmission 2 - Nov. 19, 2024 11 Postsynaptic responses can be fast or slow -Postsynaptic responses to presynaptic stimulation can be fast or slow. - When neuron 1 is stimulated, neurotransmitter is released, and activates receptors on the postsynaptic neuron, causing a relatively rapid depolarization. - When neuron 2 is stimulated, the postsynaptic response is much slower. Why are the postsynaptic responses different? Two mechanisms of receptor activation Ionotropic versus metabotropic “Neuroscience”, 3e Ionotropic Receptor - Metabotropic Receptor - Receptor is a ligand-gated channel Receptor is coupled to a G-protein Many neurotransmitters bind to receptors Transmitter binds to a G-protein receptor that are also ion channels. The channel that activates a G-protein responsible for activates after a sufficient number of some type of intracellular messaging that transmitter molecules are bound. results in channel activation. PI 29 - Neurotransmission 2 - Nov. 19, 2024 12 Different receptor types are activated Ionotropic - fast response Metabotropic - slower response - Activation of an ionotropic receptor typically produces a rapid postsynaptic response, because an ion channel is activated upon binding of the neurotransmitter. - Activation of a metabotropic receptor usually produces a much slower response because an ion channel is activated or deactivated only following a series of intracellular biochemical events that include activation of G-proteins and effector molecules. This takes time. Ionotropic vs. metabotropic receptors Ionotropic – ligand-gated ion channel receptors 1. Acetylcholine – nicotinic acetylcholine receptors (nAChR) e.g. – Receptor at neuromuscular junction 2. Glutamate - NMDA, AMPA (kainate) receptors 3. 5-HT3 – serotonin receptor channel 4. GABA (types A and C) 5. Glycine Metabotropic – G-protein coupled receptors 1. Acetylcholine – muscarinic acetylcholine receptors (mAChR) 2. Metabotropic glutamate – mGluR 3. Biogenic Amines – Serotonin, Dopamine, Norepinephrine, Histamine 4. GABA (Type B) PI 29 - Neurotransmission 2 - Nov. 19, 2024 13 Ligand-gated ion channel receptors Cys-loop - nAch, Glutamatergic – GABA (A, C), AMPA, NMDA, Purinergic (P2x) GlyR, 5-HT3R Kainate (subunit & agonist) Stoichiometry A A A A A A A A A A = ligand (agonist) Diversity of postsynaptic receptors – Different subunits combine to form channels with varying functions - Signal interpretation by the postsynaptic cell can be manipulated through many different methods. - Ionotropic receptors are typically heteromultimers, comprised of four or five different subunits. -A list of the current known isoforms of several types of ionotropic receptors is shown to the left. -The possible combinations within a given type of receptor are large. - Exactly which combinations are expressed where are still under investigation. -These various combinations can alter function of the channel, and therefore change the postsynaptic potential produced by receptor activation. PI 29 - Neurotransmission 2 - Nov. 19, 2024 14 Metabotropic Receptors - G-protein coupled 7 transmembrane segments -The structure of a metabotropic receptor is much different than an ionotropic receptor. -The active receptor is a single gene product, that weaves in and out of the membrane seven times (hence, 7 transmembrane segments). -The extracellular transmitter binding pocket is in the center of the protein. - G-proteins are then coupled to the intra- cellular portion of the protein. - Neurotransmitter binds. The binding of the neurotransmitter somehow causes activation of the G-protein, which then activates an effector protein. Biochemical events involved in metabotropic receptor activation “Neuroscience”, 3e PI 29 - Neurotransmission 2 - Nov. 19, 2024 15 Metabotropic receptor activation produces a change in postsynaptic membrane potential by increasing/decreasing ion channel activity Receptor activated by binding of neurotransmitter G-protein is activated Directly activates/deactivates channel Activation of effector molecule Increases/decreases Directly activates/ kinase activity deactivates channel Leads to phosphorylation/dephosphorylation of ion channels causing change in channel activity Ion channels are usually phosphorylated following metabotropic receptor activation “Neuroscience”, 3e - Typically, activation of a metabotropic receptor results in the phosphorylation of an ion channel. - This can either open or close the affected channel. - If, for example, a K+ channel is opened following receptor activation and the resultant phosphorylation of the channel, then the cell will hyperpolarize. Channel activity will make it less likely for the postsynaptic cell to fire an action potential. - On the other hand, if phosphorylation of the K+ channel following receptor activation closes the channel, then the cell will depolarize due to the lowered K+ conductance (permeability). The postsynaptic cell will be moved closer to its threshold potential. PI 29 - Neurotransmission 2 - Nov. 19, 2024 16 Activation of kinases that lead to protein phosphorylation and regulation of protein function Second messengers and their targets PI 29 - Neurotransmission 2 - Nov. 19, 2024 17 Second messengers and their targets Neuronal second messengers and their targets PI 29 - Neurotransmission 2 - Nov. 19, 2024 18 Examples of second messenger pathways involved in metabotropic receptor activation (deactivation) of postsynaptic ion channels “Neuroscience”, 3e Metabotropic receptor activation can alter gene expression PI 29 - Neurotransmission 2 - Nov. 19, 2024 19 Excitatory Cell Death Increased excitatory stimulation can lead to increased glutamate release. Decreased uptake by excitatory amino acid transporters (EAATs) can also lead to increased extracellular glutamate concentrations. This leads to activation of post- synaptic NMDA receptors allowing Na+ and Ca++ to pass through. Excess glutamate can spread to extrasynaptic NMDA receptors and increase overall Ca++ conductance. Increased cytosolic Ca++ can lead to apoptosis or necrosis Long term potentiation Ca2+ activates kinases e.g., CaMKII Purves 6e, Figure 8.10 Multiple glutamate AMPA EPSPs needed to depolarize enough to relieve Mg2+ block PI 29 - Neurotransmission 2 - Nov. 19, 2024 20 Long term potentiation Ca2+ influx through NMDA receptors Potential (mV) After pairing before pairing Time (ms) EPSP is mediated By AMPA receptors Induces long lasting change in postsynaptic potentials Postsynaptic potentials A local change in postsynaptic membrane potential. The PSP then spreads through local current flow. If the spreading PSP is at or above threshold as it reaches the axon hillock, the postsynaptic cell will fire an action potential. PI 29 - Neurotransmission 2 - Nov. 19, 2024 21 Neurotransmitter receptor activation typically causes a change in membrane potential This change in membrane potential is due to a change in membrane permeability – i.e., a change in ionic conductance is induced following receptor activation that results in a change in postsynaptic membrane potential, producing a PSP. The reversal potential (Erev) of the induced conductance is the equilibrium potential of this induced conductance. It is the membrane potential at which the net current flowing through the induced conductance is zero. It is the membrane potential at which the induced current switches from moving inward to outward, or vice versa. For an induced conductance in which the membrane permeability for a single ionic species is changed, E rev = Eeq. for this ion. When the induced conductance changes permeability for more than one ionic species, then Erev reflects the balance between the electrochemical potentials of these ions. EPSP and IPSP excitatory and inhibitory postsynaptic potentials Following neurotransmitter receptor activation, the postsynaptic membrane potential will always move toward the reversal potential of the activated neurotransmitter receptor induced conductance This change in membrane potential can either increase or decrease the likelihood for a given neuron to fire an action potential Postsynaptic potentials that increase the probability of causing the postsynaptic cell to fire an action potential are Excitatory PostSynaptic Potentials (EPSP). Postsynaptic potentials that decrease the probability of causing the postsynaptic cell to fire an action potential are Inhibitory PostSynaptic Potentials (IPSP). How does one define whether a given PSP is excitatory or inhibitory? PI 29 - Neurotransmission 2 - Nov. 19, 2024 22 EPSP and IPSP Excitatory and inhibitory postsynaptic potentials -An EPSP is produced when the reversal potential of the conductance activated by the neurotransmitter is more positive than threshold. - An IPSP is produced when the reversal potential of the conductance activated by the neurotransmitter is more negative than threshold. -Thus, an EPSP always produces a depolarization, while an IPSP can either hyperpolarize or depolarize the membrane. - An IPSP simply makes it less likely that threshold will be reached, while an EPSP makes it more likely. Neurotransmitters - Excitatory and Inhibitory Major excitatory neurotransmitters - The general effect is the depolarization of the postsynaptic membrane caused by the activation (or deactivation) of cationic channels. Acetylcholine Glutamate Catecholamines - epinephrine, norepineprhine Serotonin Histamine Major inhibitory neurotransmitters - The general effect is the hyperpolarization (although slight depolarizations might be produced) of the postsynaptic membrane caused by the activation of chloride channels. -aminobutyric acid (GABA) Glycine PI 29 - Neurotransmission 2 - Nov. 19, 2024 23 Examples of PSPs produced by various neurotransmitters The previous figure showed the expected PSPs produced at postsynaptic glutamatergic and gabaeric neurons. -Glutamate, activates a cationic channel, with a reversal potential (Erev) around 0 mV. This is above the threshold potential, and therefore, glutamate will produce an EPSP. -GABA activates a chloride channel, with Erev around -60 mV. Even though activation of the GABA channel produces a slight depolarization, the maximal depolarization that could be produced is to -60 mV. If this is below the threshold potential, then an IPSP is produced. How to determine whether an EPSP or IPSP will be produced following receptor activation In order to determine whether an EPSP or IPSP will be produced following activation of a receptor, the following questions should be addressed: 1) What is the reversal potential (Erev) of the receptor-activated conductance? 2) What is the threshold potential? 3) Is Erev above or below the threshold potential? If Erev is above threshold, then an EPSP results. If Erev is below threshold, then an IPSP results. PI 29 - Neurotransmission 2 - Nov. 19, 2024 24 Examples of conductance changes that produce EPSPs and IPSPs Increasing postsynaptic conductance of: Produces: EPSP Na+ ------------------------------------------------------------------------------------------------ IPSP K+ ------------------------------------------------------------------------------------------------ EPSP Ca+ ----------------------------------------------------------------------------------------------- IPSP Cl- ------------------------------------------------------------------------------------------------ Na+/K+ equally ------------------------------------------------- EPSP Decreasing postsynatpic conductance of K+ produces --- EPSP Several neurotransmitters/receptors can affect a single synapse PI 29 - Neurotransmission 2 - Nov. 19, 2024 25 Convergence and Divergence - Convergence of input: Convergence Divergence several neurons synapse onto a single neuron. - Divergence of output: a single neuron projects onto several neurons. Grand postsynaptic potential Sum of all PSPs determines whether the postsynaptic cell fires an action potential How is the Grand PSP determined? PI 29 - Neurotransmission 2 - Nov. 19, 2024 26 Summation Effects of all EPSPs and IPSPs determine the excitability of the cell - Temporal and spatial summation of EPSPs and IPSPs determines the excitability of the postsynaptic cell. Presynaptic inhibition and facilitation C -Presynaptic modulation occurs when a third neuron (C) synapses onto the presynaptic membrane (A), impacting the + facilitation amount of neurotransmitter released by A. A - Presynaptic facilitation results when B neuron C causes neuron A to release greater amounts of neurotransmitter. - Presynaptic inhibition results when C neuron C causes neuron A to release less neurotransmitter. A - inhibition -The change in the amount of neurotransmitter released is typically caused by a change in rise in presynaptic B Ca++. PI 29 - Neurotransmission 2 - Nov. 19, 2024 27 Drugs of abuse and therapy that alter synaptic transmission Topic Self-assessment 1. Describe the lifecycles, from synthesis through inactivation, of the major classic neurotransmitters. 2. Understand the distinctions between peptide and small molecular NTs – synthesis, storage, and receptors activated. 3. What are the central pathways for the biogenic amines? 4. Describe the differences between an ionotropic and metabotropic receptors. 5. Describe the events following activation of a metabotropic receptor. 6. What second messenger pathways are involved in chemical neurotransmission? 7. How does the membrane potential change during an EPSP; during an IPSP? 8. Why are ACh and glutamate excitatory neurotransmitters, while GABA is generally an inhibitory neurotransmitter, and how does one define a neurotransmitter as excitatory or inhibitory? 9. When will a postsynaptic cell fire an action potential? 10. How can a presynaptic cell be modulated, and what affect does that have on the excitability of the postsynaptic cell? 11. Describe the different types of postsynaptic events that might affect a single neuron. PI 29 - Neurotransmission 2 - Nov. 19, 2024 28 Supplemental Readings PI 29 - Neurotransmission II: Neurotransmitters, postsynaptic signaling, and synaptic integration and modulation in the CNS - Tuesday, November 19, 2024, 9 AM I. Berne and Levy Physiology: Chapter 6 Synaptic Transmission Notable Figures and Tables: Table 6.1 Figures 6.1, 6.2, 6.6, 6.8, 6.11, 6.12A,B, Box 6.1 – Do not memorize this list. You will re-encounter MANY of these peptide neurotransmitters during “Beginning to End” in Year 2 as part of Endocrine and GI segments. When the text refers to “the initial segment,” they’re leaving out some important additional information. The “initial segment” refers to the initial segment of the axon and is synonymous with the term “axon hillock.” Synaptic Transmission: 1) What are the two types of synapses? How are they similar to each other and how are they different? What are the molecular structures underlying these two types of synapses? 2) What are the ways that neurotransmitter can be released from nerve terminals? Which one is more likely in a “fast” synapse and which in a “slow” synapse? 3) What is a post-synaptic potential and why is synaptic integration important for transmission of signals? 4) What are the different types of modulaton that can occur with post-synaptic neurons? What types of effects can produce these modulations? What is the outcome of these modulations? Neurotransmitters: 1) Where/how is the neurotransmitter (NT) synthesized and packaged? 2) How is the NT removed from the synapse? Is it enzymatically broken down or taken up by specific transporters? Is the NT recycled or resynthesized? 3) What type of NT is it? (“classical”/”nonclassical”, amino acid, biogenic amine, neuropeptide) 4) What are the biochemical pathways for catecholamines, serotonin, and histamine? Receptors: 1) Does the NT have receptors that are ionotropic? Metabotropic? Both? Describe the differences between an ionotropic and metabotropic receptor. 2) Are the receptors located post-synaptically or pre-synaptically? 3) What effect does activating the receptor have? (Depolarization, hyperpolarization, activate a G-protein, excitatory post-synaptic potential, inhibitory post-synaptic potential, pre-synaptic inhibition of NT release, pre-synaptic potentiation of NT release) 4) What happens when a metabotropic receptor is activated? What second messenger pathways are involved in chemical neurotransmission? 5) Are there notable exogenous substances that act as agonists or antagonists for these receptors? (Hint – the names of many of the receptor types are based on a substance that acts as an agonist) 6) How does the membrane potential change during an EPSP; during an IPSP? 7) Why are ACh and glutamate excitatory neurotransmitters, while GABA and glycine are generally inhibitory neurotransmitters? How does one define a neurotransmitter as excitatory or inhibitory? Note: Do not worry about the specific molecular organization (number/type of subunits, number of transmembrane domains, subunit(s) responsible for ligand binding, etc.) of the receptors beyond recognizing that these influence the properties of the receptors – e.g. ion conductance, G-protein association, localization in plasma membrane, etc. II. Boron and Boulpaep “Medical Physiology” Chapter 13 Sections titled: “Some transmitters are used by diffusely distributed systems of neurons to modulate the general excitability of the brain” (P311-314) 1) Where are the soma of the neurons found for the neurotransmitters mentioned in this section? 2) What functions/roles do these neurotransmitters have or are suspected to have? 3) Where do these neurons project to? “Synaptic transmitters can stimulate, inhibit, or modulate the postsynaptic neuron” (P318-320) 1) What are the differences between excitatory, inhibitory, and modulatory synapses? Can a single neuron have examples of all three? 2) What neurotransmitters are associated with excitatory and inhibitory synapses? 3) What does the reversal potential indicate? “Fast Amino Acid–Mediated Synapses in the CNS” (P322-328) [Essentially starting here, read to the end of the chapter] 1) What are the three types of ionotropic glutamate receptors? What are some differences between them? Which ion(s) flow through the pore when activated? 2) What type of ion flows through GABAA and GABAC receptors? What are some examples of allosteric modulators of GABAA? 3) What is the importance of stimulus frequency in neurotransmission? “Plasticity of Central Synapses” (P328-333) 1) What are some types of short-term synaptic changes? Where are they found? 2) What are some types of long-term synaptic changes? Where are they found? 3) What is long-term potentiation and how can it be generated? Notable Figures: Figure 13-7, 13-8, 13-11, 13-16, 13-17, 13-19, 13-20, 13-21 Table 13-2