Renal Physiology PDF
Document Details
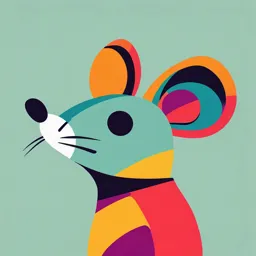
Uploaded by PeacefulAcer
Tags
Summary
This document provides an overview of renal physiology, covering topics such as total body water balance, body fluid compartments, osmotic equilibria, renal structure, glomerular filtration, and glomerular filtration rate (GFR). It details the processes involved in maintaining homeostasis and the regulation of various electrolytes and hormones.
Full Transcript
TOTAL BODY WATER - Intake vs. Output Water INTAKE (2,300 ml/day) 2/3 liquids 1/3 solids ~200 ml metabolic oxidation of food Water OUTPUT (2,300 ml/day) 1400 ml urine (60%) 450 ml skin1 & sweat2 350 ml respiratory tracts 100 ml faeces3 1 loss due to burns 2 loss due to hot...
TOTAL BODY WATER - Intake vs. Output Water INTAKE (2,300 ml/day) 2/3 liquids 1/3 solids ~200 ml metabolic oxidation of food Water OUTPUT (2,300 ml/day) 1400 ml urine (60%) 450 ml skin1 & sweat2 350 ml respiratory tracts 100 ml faeces3 1 loss due to burns 2 loss due to hot weather, strenuous exercise 3 loss due to diarrhoea Body Fluid Compartments Total Body Fluid (40 l) = ICF (25 l) + ECF (15 l) ECF (15 l) = Interstitial fluid (12 l) + Plasma (3 l) Blood (5 l) = Haematocrit (2 l) + Plasma (3 l) Plasma communicates continually with the interstitial fluid through pores in capillaries Principal Constituents of ICF and ECF Osmotic Equilibria OSMOSIS: NET DIFFUSION OF WATER MOLECULES TO THE SIDE WITH THE GREATER CONCENTRATION OF NON-PERMEANT MOLECULES. The greater the difference in solute (non-permeant molecule) concentration, the greater the tendency for osmosis. OSMOTIC PRESSURE: refers to the pressure which has to be applied across the semi-permeable membrane in the direction opposite to that of osmosis, to prevent osmosis from occurring. Osmotic pressure is therefore created by the non-permeant ions and molecules. Note that the size of the molecule is irrelevant: 1 molecule of albumin (70kDa) exerts same osmotic pressure as 1 molecule of glucose (180Da). Osmotic Equilibria Osmotic pressure is determined by the number of non-permeant particles in a solute. [osmolality] Osmotic Pressure (mmHg) = 19.3 x Osmolality (mOsm/kg water) 4/5 of the total osmolality of ECF is carried by Na+ and Cl- ions 1/2 of the total osmolality of ICF is carried by K+ ions Interstitial Fluid Plasma ICF 5430 5450 5430 Plasma proteins maintain ~ 20 mmHg greater Total Osmotic Pressure (mmHg) pressure in capillaries. Osmotic Equilibria A fluid into which normal body cells can be placed is either: Isotonic - same osmolality; no net movement of water (e.g. 0.9% NaCl [normal saline], 5% dextrose in W, Ringer’s Lactate) Hypotonic - lower osmolality; net movement of water into cell (swells) (e.g. 0.45% NaCl, 2.5% dextrose in W) Hypertonic - higher osmolality; net movement of water out of cell (shrinks) (e.g. 3% NaCl, 10% dextrose in W, 5% dextrose in 0.45% saline) A. Maintenance of RENAL Body Fluid Homeostasis PHYSIOLOGY WATER and ELECTROLYTE balance Regulation of 1. Regulation of sodium (Na+) and water balance ECF Osmolality 2. Regulation of potassium (K+) balance ECF Volume 3. Regulation of calcium (Ca2+) and phosphate ([PO4]3-) balance 4. Regulation of acid-base (H+) balance (pH) Blood Volume Clearance of METABOLIC END-PRODUCTS Blood Pressure EPO production B. Hormogenesis Activation of Vitamin D3 Overview of Renal Structure The basic functional unit of the kidney is the nephron. A. Glomerulus - an epithelial capsule (Bowman’s capsule), which houses a tuft of 20-40 capillary loops. B. Renal tubule (1) Proximal convoluted tubule (2) Loop of Henle (3) Distal convoluted tubule (4) Collecting tubule and duct Schematic Overview of Renal Function PT, loop of Henle, DT, CD [ 2b ] Blood 180 l/day Urine 1 - 1.5 l/day [ 2a ] Glomerulus blood cells proteins Reabsorbed filtrate (>99%) [ 1 ] Filtering of Blood a) Reabsorption of required elements1 [ 2 ] Processing of Filtrate b) Excretion of non-required elements2 1 water, Na+, K+, Ca++, [PO4]3-, glucose, aminoacids, vitamins 2 metabolic end-products (urea, creatinine, uric acid), drugs, toxins Blood Supply of Kidney Blood Supply of Kidney Blood enters the glomerulus through the afferent arteriole, which subdivides into a tuft of capillary loops (renal glomerulus), enclosed in Bowman’s capsule. The internal layer of the capsule envelopes the glomerular capillaries - podocytes. These are cell bodies from which arise several processes that embrace capillaries of the glomerulus. The podocyte processes interdigitate, forming 25 nm filtration slits. Between the ext. and int. layers of Bowmans capsule is the urinary space which receives fluid filtered through the glomerular membrane. This fluid then proceeds to pass through the urinary pole and thence into the renal tubule. Blood leaves the glomerulus through the efferent arteriole (formed from glomerular capillary tuft). These arterioles then divide again to form an extensive network of capillaries called the peritubular capillary network. This network surrounds and lies alongside the whole length of the renal tubule. Water and electrolytes are reabsorbed from the filtrate (as it flows through the tubules) back into the plasma of the peritubular capillaries. This is due to rapid osmosis resulting from the high oncotic pressure of the plasma proteins. The capillary network collects into venules --> veins. Each collecting duct collects urine from ~ 4000 nephrons Glomerular Filtration The glomerular membrane has 3 major layers: Endothelial layer of the capillary : thousands of 50- 100 nm holes (fenestrae) Basement membrane : a meshwork of cross-linked collagen fibrils, proteoglycans, laminin Interdigitating foot processes of podocytes form a uniformly wide, porous slit diaphragm Glomerular Filtration The glomerular membrane is: highly permeable (several hundred times that of a typical capillary membrane) highly selective for the size of molecules it allows to pass through. e.g. albumin (69kDa - smallest plasma protein): 0.005%; inulin (5.2kDa): 100%. The glomerular membrane is in fact almost completely impermeable to all plasma proteins, but is highly permeable to essentially all other dissolved substances in normal plasma. 1. ANIONIC (negatively charged) FILTRATION BARRIER: Glomerular Basement Membrane 2. SIZE-SELECTIVE FILTER: Podocyte Slit Diaphragm; nephrin molecules from two neighbouring foot processes interact in the centre of the slit to form a zipper-like structure. Podocyte Slit Diaphragm Glomerular Filtration Composition of filtrate [primary urine] = composition of plasma - plasma proteins In a number of glomerular diseases, the negative filtration barrier is lost secondary to immunologic damage and inflammation (glomerulonephritis). As a result, proteins appear in urine (proteinuria). 1.0 0.8 i 0.6 l\ Loss of nega tive charges on filtration barri er > ·..:: 0 0.4 Q) a::: 0.2 0 j__ --- ,-- --, --- =:: ;::: :::= :=~ --- --~ =;.__, 18 22 26 30 34 38 42 46 Effective molecular radiu s (A) Figu re 3-5 Redu ction of the nega tive char ges on the filtr atio n barr ier of the glom cur in man y imm unol ogic ally med iated erul us, as can oc- rena l dise ases , resu lts in the filtr atio n sis of size only. In this situa tion, the· relat of prot eins on the ba- ive ftlte rabil ity of prot eins depe nds only radiu s. Acco rding ly, the excr etio n of poly on the mol ecul ar anio nic prot eins (20 to 42 A) in the urin caus e mor e prot eins of this size are filte e incr ease s be- red. Glomerular Filtration In congenital nephrotic syndrome, massive proteinuria at birth because of lack of the podocyte slit diaphragm. Caused by mutations in the gene encoding nephrin (NPHS1). Glomerular Filtration Rate (GFR) The GFR is defined as the quantity of glomerular filtrate formed each minute by both kidneys. The GFR is tightly-controlled, and kept around 125 ml/min. This is very important because: at a very HIGH GFR, kidney would fail to reabsorb water & solutes at a very LOW GFR, kidney would fail to eliminate waste products Autoregulation of GFR is carried out by local feedback control mechanisms Glomerular Filtration Rate Afferent arteriole Efferent arteriole +HP -OP Kf GFR = Filtration pressure (PUF) x Filtration coefficient (KF) Filtration Pressure (PUF) is the net pressure forcing fluid through the glomerular membrane: PUF = Hydrostatic pressure (HP) gradient - Oncotic pressure (OP) gradient Filtration Coefficient (KF) depends on the µ and s/area of the glomerular membrane. Glomerular Ultrafiltration Pressure (PUF) Filtration Pressure (PUF) is the net pressure forcing fluid through the glomerular membrane: PUF = Hydrostatic pressure (HP) gradient - Oncotic pressure (OP) gradient Autoregulation of GFR by kidney GFR is autoregulated by alterations in Hydrostatic Pressure (and hence in the PUF) that are mediated by changes in glomerular arteriolar resistance. A fferent Arteriolar Constriction E fferent Arteriolar Constriction* resistance to inflow into glomeruli resistance to outflow from glomeruli decreased hydrostatic pressure in glomeruli increased hydrostatic pressure in glomeruli ¯ PUF ¯ GFR PUF GFR Autoregulation of GFR by kidney A fferent Arteriolar Constriction E fferent Arteriolar Constriction Autoregulation of GFR by kidney \ in response to a LOW GFR : i. Afferent arteriolar dilatation GFR ii. Efferent arteriolar constriction \ in response to a HIGH GFR : i. Afferent arteriolar constriction ¯ GFR ii. Efferent arteriolar dilatation Tubuloglomerular Feedback Mechanism Alterations in afferent/efferent arteriolar resistance are effected by the TUBULOGLOMERULAR feedback mechanism - responds to changes in [NaCl] of tubular fluid. responds to changes in the sodium concentration The JUXTAGLOMERULAR COMPLEX. The distal tubule passes in the angle between the afferent and efferent arterioles, actually abutting each of these two arterioles (macula densa). Macula densa cells detect changes in the Na+ (black patch) filled with renin and atp and Cl- concentrations in the tubular fluid. in order to detect changes in GFR Change in GFR Change in [NaCl] in DT Detected by MACULA DENSA cells Tubuloglomerular Feedback Mechanism Alterations in afferent/efferent arteriolar resistance are effected by the TUBULOGLOMERULAR feedback mechanism - responds to changes in [NaCl] of tubular fluid. The JUXTAGLOMERULAR COMPLEX. The distal tubule passes in the angle between the afferent and efferent arterioles, actually abutting each of these two arterioles (macula densa). Macula densa cells detect changes in the Na+ and Cl- concentrations in the tubular fluid. Change in GFR low GFR high GFR Change in [NaCl] in DT decrease in [NaCl] in DT increase in [NaCl] in DT Detected by MACULA DENSA cells Tubuloglomerular Feedback Mechanism Change in GFR Change in [NaCl] in DT Detected by MACULA DENSA cells afferent arteriolar vasoconstriction / dilatation AND efferent arteriolar vasodilatation / constriction Tubuloglomerular Feedback Mechanism Macula densa cells signal: (1) AFFERENT arteriolar vasoconstriction by releasing ATP (in response to a high GFR) into blood (2) EFFERENT arteriolar vasoconstriction by releasing RENIN, with formation of ANGIOTENSIN II [Renin-Angiotensin-System] (in response to a low GFR) Role of ATP in macula densa cell signaling An increase in [NaCl]L leads to an array of intracellular signaling events in the macula densa cells, which, in turn, increases the release of ATP. ATP can then activate P2 receptors on the vascular smooth muscle cells of the afferent arteriole, causing vasoconstriction. Paracrine action of ATP on the afferent arterioles causing a decrease in GFR Copyright ©2005 American Physiological Society When GFR is low ACE is found in endothelial cells in lungs (it is produced in them) SARS-CoV-2 People with covid-19 have high levels of angiotensin II Too much angiotensin II can cause harm and thus it needs to be broken down filtrate passes more rapidly Less absorption of High GFR through the tubules Na+ and Cl- ions in PT and loop of Henle \ macula densa cells in distal tubule detect [Na+] and [Cl-] in filtrate Release of ATP (mesangial cells) (¯ renin) Afferent arteriolar constriction Efferent arteriolar dilatation ¯ PUF ¯ GFR filtrate passes more slowly More absorption of Low GFR through the tubules Na+ and Cl- ions in PT and loop of Henle \ macula densa cells in distal tubule detect ¯ [Na+] and ¯ [Cl-] angiotensinogen (liver) in filtrate Release of RENIN from juxtaglom. cells (¯ ATP) ANGIOTENSIN I --> II Afferent arteriolar dilatation Efferent arteriolar constriction PUF GFR GFR Autoregulation When both these mechanisms function together, the GFR increases only a few percent even though the arterial BP changes between 80 and 180 mmHg. Decrease in blood pressure, decreases GFR Increase in blood pressure, increases GFR Despite changes in blood pressure, the autoregulatory However autoregulation keep GFR constant mechanism keeps GFR constant and keeps kidney working properly When the mean blood pressure is lower than 80, perfusion of kidney occurs as they are unable to maintain the filtration. Estimation of GFR CREATININE is used to estimate the GFR in clinical practice. Creatinine is a by-product of skeletal-muscle creatine metabolism, and is Creatine is used as a phosphate /energy store for muscle. Creatinine is a produced at a relatively constant rate. waste product produced at a relatively constant rate (it is equivalent to muscle mass) , it is excreted in urine leading to being able to know an estimate for GFR level Creatinine is not sensitive to changes in GFR Estimation of GFR Serum creatinine levels* Creatinine clearance provides a reasonably accurate measurement of GFR. *A fall in GFR (creatinine levels) may be the first sign of renal disease. A 50% loss of functioning nephrons will reduce the GFR by ~20-30%. GFR must decline substantially before an creatinine is detected! When the creatine really starts to A more accurate way of checking GFR is not by assuming creatinine level in blood but in urine increase, this means that the kidney has already lost a lot of its function Creatinine Clearance Processing of the Filtrate in the Renal Tubules Reabsorption plays a much greater role than does secretion in formation of urine (99% of water, almost all glucose and amino acids). Secretion is especially important in determining the amount of K+ and H+ ions. Can be secreted from the blood into the filtrate in order to control their levels better The basic mechanisms for transport through the tubular membrane are essentially the same as those used in other membranes, namely: (1) ACTIVE TRANSPORT (2) PASSIVE TRANSPORT 1º Active Transport through the Tubular Membrane Brush border increases surface are dramatically Proximal tubule cell Na-K-ATPase pumps located in basolateral membrane (energy consumption = mito) 3 Na+ ions pumped out for every 2 K+ ions taken in: Þintracellular [Na+] is reduced to very low levels Þintracellular negative potential of - 70 mV this gradient will cause sodium ions from the filtrate to move into the cell, this is very energy dependent These 2 factors cause Na+ to diffuse from the tubular lumen into the cell. 2º Active Transport through the Tubular Membrane same mechanism as used in the gut Secondary because absorption occurs as a result of (i.e. secondary to) the 1°active transport of sodium ions by Na-K-ATPase. Thus, no energy is used directly from ATP in secondary active transport. As Na+ moves down its electrochemical gradient to interior of the cell it pulls another molecule (e.g. glucose or amino acid) with it. This form of transport is called co-transport and is carried out by Na carrier proteins. These tubular cells are prone to ischemia, which can sometimes cause death of these cells Usually, carrier protein is specific for transport of one class of molecule/ion. Glucose, amino acids, chloride ions, phosphate, calcium, magnesium. 2º Active Glucose Transport (SGLT1) SGLT is sodium glucose transporter There are drugs that act as SGLT inhibitors by blocking SGLT and decrease glucose levels in blood by increasing glucose levels in urine. 2º Active Secretion into the Tubular Lumen Secondary because secretion occurs as a result of (i.e. secondary to) the 1°active transport of Na ions through the tubular membrane by Na-K-ATPase. As Na+ moves down its electrochemical gradient to the interior of the cell another ion is pumped out. This form of transport is called counter-transport. Some sodium carrier proteins in the brush border of tubular cells secrete an ion while taking up sodium. Examples of substances that are secreted in this way include H+ and K+ ions. Passive Absorption of Water by Osmosis High concentration of solutes (high osmolality) in interstitium [primary and secondary active transport mechanisms] Water moves from low to high osmolarity. Plasma proteins are non-permeant molecules which will pull water to be reabsorbed from filtrate. High oncotic pressure (i.e. by proteins) in peritubular capillaries Processing of Filtrate by Nephron Osmolarity refers to the number of solute particles per 1 L of solvent, whereas PT osmolality is the number of solute particles in 1 kg of solvent Reabsorption of 65% of glomerular filtrate Water, Na+, glucose, amino acids, proteins (pinocytosis), vitamins Ca++, Mg++, Cl-, HCO3- (as carbon dioxide) Osmolality unchanged [300 mOsm/L] Osmolarity Thin segment of descending limb of loop of Henle Passively permeable to water only - countercurrent mechanism Osmolality [1200 mOsm/L] Osmolarity Thick segment of ascending limb of Henle’s loop and first half of DT Only solutes absorbed Entirely impermeable to water and urea Active uptake of Na+, and co-transport of Cl-, K+ Osmolality ¯ [100 mOSm/L] Osmolarity Processing of Filtrate by Nephron Second half of DT and CD Normally impermeable to water and urea Made highly permeable to water and moderately permeable to urea by ADH (control over the extent of dilution of urine) allows absorption of water Reabsorption of Na+ and secretion of K+ in presence of aldosterone (control of K+ levels in ECF) Special cells that secrete H+ into the tubular lumen via primary active transport (control of acid-base balance) Urine osmolality [50-1200 mOsm/L] regulated by ADH, and to a lesser extent aldosterone. 10 0.8 Processing of Filtrate by Nephron Substances reabsorbed completely, or almost completely Na+, K+ (secreted by distal tubule & collecting-duct), Ca++, Mg++, Cl-, HCO3- Glucose Amino acids and proteins Vitamins Substances not at all reabsorbed, or very slightly, by kidneys Urea Creatinine (metabolic end-products) Reabsorption of Water by the Nephron Reabsorption of water * Proximal tubule 65 % Thin segment of descending limb of loop of Henle 15 % Thick segment of ascending limb of loop of Henle ---- Distal Tubule & Collecting duct 19.3 % ** * Occurs entirely by osmotic diffusion; i.e. ‘follows’ absorption of solutes ** Under control of ADH (and to a lesser extent, aldosterone); reabsorbed to varying degrees according to state of hydration Excretion of a CONCENTRATED Urine The mechanism for excreting excess solutes (conc. urine) is essentially : (1) presence of ADH (2) Countercurrent Mechanism In presence of ADH, the epithelium of the late distal tubule and collecting duct is highly permeable to water. However, water in the tubular fluid can be pulled by osmosis only if there is a hyperosmolar fluid in the medullary interstitium. The principal cause of the greatly increased medullary osmolality is active transport of Na+ (plus co-transport of K+ and Cl- ) out of the thick portion of the ascending limb of the loop of Henle and into the medullary interstitium - Countercurrent Multiplier Mechanism Countercurrent Multiplier Mechanism Countercurrent Multiplier Mechanism Anti-Diuretic Hormone The most important signal that regulates the amount of water that is reabsorbed by the kidney is Anti Diuretic Hormone (ADH; vasopressin). ADH is a 9 amino-acid peptide, synthesised in neuroendocrine cells located within the supraoptic and paraventricular nuclei of the hypothalamus. The hormone is packaged in granules and transported down the axon to be stored in the nerve terminals in the posterior pituitary, from where it is released. Anti-Diuretic Hormone The primary action of ADH on the kidneys is to the permeability of the late DT and CD to water (and urea). Binding of ADH to a receptor on the basolateral membrane of the cell results in insertions of water channels (aquaporins) into the apical membrane of the cells. Under the influence of ADH, cells of late distal tubule and collecting duct are made permeable to water, thereby allowing the kidney to conserve water. Conversely, in the absence of ADH, cells of late distal tubule and collecting duct remain impermeable to water, thereby the kidney excretes a dilute urine and excess water is lost from the body. H2 O No ADH ADH Impermeable to water Permeable to water Aquaporins - the mechanism of action of ADH Discovery of Aquaporins: NOBEL PRIZE IN CHEMISTRY 2003 Renin-Angiotensin system activated by macula densa Cells (low GFR) Ang II Stimuli for ADH release: in ECF osmolality (osmoreceptors) ¯ in ECF volume (atrial stretch) ¯ in blood pressure (baroreceptors) angiotensin II (low GFR; RAA system) HIGH OSMOLARITY LOW BLOOD VOLUME Excretion of a CONCENTRATED Urine - ADH 0.16% X 100 16% Control of ECF Osmolality and Volume Now that the mechanisms by which the kidney can excrete either a dilute or a concentrated urine have been discussed, we can explain how these mechanisms are manipulated to control ECF osmolality and sodium concentration (sodium ions play the dominant role in determining ECF osmolality). (1) Osmoreceptor-ADH mechanism (2) Thirst mechanism N.B. These mechanisms are also involved in regulation of blood (ECF) volume, since disorders of water balance are manifested by alterations in the body fluid osmolality. Osmoreceptor-ADH feedback mechanism THIRST Mechanism A person is continually being dehydrated - formation of urine, evaporation from skin and lungs. ECF volume continually ¯ and ECF osmolality continually When [Na+] rises slightly (1-3%) above normal, the sensation of thirst is triggered by THIRST CENTER in the hypothalamus. A person normally drinks precisely the required amount of fluid to bring the ECF fluid back to normal. Then, the process of dehydration and increasing [Na+] begin again…. Sodium balance Aldosterone regulates Na+ excretion (RAAS) [Na+] affects plasma & ECF osmolarity (directly) [Na+] affects BP & ECF volume (indirectly) ALDOSTERONE Aldosterone is synthesised by the adrenal cortex (zona glomerulosa). sex hormones not that imp, no need to know pathways ALDOSTERONE Control of Na+ and K+ secretion by ALDOSTERONE Aldosterone induces the cells of the late DT and CD to: - Uptake Na+ (hence also H2O reabsorption) - Secrete K+ In presence of aldosterone, almost all of the remaining 8% of sodium is reabsorbed. Major stimuli for aldosterone secretion: [K+] plasma ANGIOTENSIN-II (R.A.A. system) This mechanism is also involved in control of ECF volume. Brain R.A.A. system 1--..ADH - - Angiotensin II I I Angiotensin I -Ang ioten sin II t4------,. Angiotensinogen Ad ren al-A t t Aldosterone Renin - - - - - - - J, Na+ excretion j, H20 excretion com pone nts of the renin -ang ioten sin-a ldos- Figure 6-2 Sche mati c repr esen tatio n of the essen tial ease in the excr etion of Na+ and wate r by terone syste m. Acti vatio n of this syste m resu lts in a decr the_kidneys. NOTE:Ang ioten sin I is conv erted to angioten sin II by an angi oten sin-c onve rting enzy me, show n, the endo theli al cells with in the lung s ':!..,,hich is pres ent on all vasc ular endo theli al cells. As , Antidiuretic horm one. See text for deta ils. Play a sign fican t role in this conv ersio n proc ess. ADH Control of Na+ and K+ excretion by ALDOSTERONE ALDOSTERONE promotes K+ ion secretion by activating the Na-K-ATPase Overview of K+ homeostasis (3.5-5.1 mmol/L plasma) Overview of K+ homeostasis Skeletal muscle Red blood cells Kidneys excrete Liver Bone 90-95% of ingested K+ Excretion = intake even when intake increases 10-fold! HYPOKALAEMIA HYPERKALAEMIA Plasma K+ < 3.5 mmol/l Plasma K+ > 5 mmol/l Skeletal muscle weakness (mild to Muscle twitching, cramps total paralysis) Numbness GI hypomotility (ileus, constipation) ECG changes (peaked T wave) Ventricular arrhythmias, cardiac arrest Ventricular fibrillation, cardiac arrest Atrial Natriuretic Peptide ANP (28 a.a in length) is synthesised, stored, and released from atrial cardiocytes. The main stimulus for secretion of ANP is atrial distension (hypervolaemia). High blood volume Essentially, ANP antagonises the R.A.A. system by promoting excretion of NaCl and water by the kidneys. 1. ¯ renin secretion ; ¯ aldosterone secretion 2. GFR by dilatation of afferent arterioles and constriction of efferent arterioles. Atrial stretch receptors Arterial baroreceptors Same as ANP Renal Regulation of Blood Volume The extreme degree of precision with which the BV is controlled is shown below. Almost no change in BV occurs despite tremendous changes in daily fluid intake, except when intake becomes very low. Physiology of Diuretic Action A DIURETIC is a substance that increases the rate of urine output. The principal use of diuretics is to reduce the total amount of fluid in the body (diuresis). They are especially important in treating oedema and hypertension. Cause a natriuresis When a diuretic is used it is important that the rate of sodium loss in the urine is also increased. If not, i.e. if water alone were removed, the body fluids would become hypertonic. As a result, an osmoreceptor response would be stimulated, with release of ADH and conservation of water by the kidney. This would nullify the effect of the diuretic. Therefore, all diuretics cause marked natriuresis as well as diuresis. “Loop” Diuretics Frusemide (Lasix®); Bumetanide (Burinex®) Block active Na-K-2Cl co-transport in the thick ascending limb of the loop of Henle This causes diuresis for 2 main reasons: It allows greatly increased quantities of solutes to be delivered into the distal portions of the nephrons, and these then act as osmotic agents to prevent water reabsorption as well It results in a decreased solute concentration in the medullary interstitial fluid. Consequently, the concentrating ability of the kidney by the countercurrebnt mechanism is greatly decreased, so that reabsorption of fluid in the collecting ducts is further decreased. Because of these two effects, 20-30% of the glomerular filtrate may be delivered to the urine. Potassium supplementation (KCl - SlowK®) may be necessary. “Loop” diuretics Furosemide (Lasix®); Bumetanide (Burinex®) Block active Na-K-2Cl co- transport in the TAL of the loop of Henle (25% of filtered Na load) low potassium Hypokalaemia may result, in which case K+ supplementation (SlowK®). Or combination with K-retaining diuretic/ACE-I. Blocking of co-transporter also results in reduced lumen-positive voltage >> Mg, Ca excretion increased. Hypocalcaemia, hypomagnesaemia may result. low calcium and low magnesium Thiazide diuretics Chlorothiazide, Bendrofluazide Block active Na-Cl cotransport in early DT (5-10% of filtered Na load) Less risk of hypoK, HypoMg. K+-sparing/retaining diuretics The least used due to high risk of hyperkalaemia, normally used together with a loop diuretic Na+ channel blockers in late DT/CD– Amiloride (~3% of filtered Na load) Competitive aldosterone inhibitors - Spironolactone (Aldactone®) Risk of hyperkalaemia with spironolactone! (much less with amiloride) Solute excretion with diuretics Diuretic Na+ K+ excretion excreti on Loop 25% Diuretic Thiazide Diuretic 5-10% () Amiloride 3% (¯) Spironolactone 3% ¯ ACE-I and ARBs (dry cough!) Enalapril Perindopril angiotensin converting enzyme Valsartan Candesartan Atrial stretch receptors Arterial baroreceptors ACID-BASE BALANCE The normal pH of arterial blood is 7.4 (narrow: 7.35 - 7.45) The lower limit at which a person can live for a few hours is ~6.8 The upper limit is ~8.0 Intracellular pH around 6.0-7.4 Acid Input: Diet: foods contain organic acids e.g. fatty acids, amino acids. Metabolism: intermediates in the Krebs cycle, lactic acid; carbonic acid (from CO2) is biggest source on a daily basis; ketoacids (DKA). Defences against changes in pH 3 main (successive) lines of defence: (1) BUFFER SYSTEMS in body fluids Within seconds, buffer systems combine with or releases H+ ions accordingly to offset any changes in pH. (2) RESPIRATORY SYSTEM Within minutes to hours, the respiratory centre causes a change in the breathing rate (ventilation) which alters the PCO2 to cause pH to return towards normal. Can take care of 75% of most pH disturbances. (3) RENAL SYSTEM Within several days, the kidneys adjust excretion of bicarbonate and net acid, helping to readjust the [H+] of body fluids back to normal. Bicarbonate Buffer System CO2 + H2O Û H2CO3 Û H+ + HCO3- Addition of an acid: CO2 + H2O Ü H2CO3 Ü H+ + HCO3- (more buffer in the form of CO2) Addition of an alkali: CO2 + H2O Þ H2CO3 Þ H+ + HCO3- (more buffer in the form of HCO3- ) The bicarbonate buffer system is very important because the concentration of each of the two elements (i.e. CO2 and HCO3- ) can be regulated by the respiratory and renal systems, respectively. As a result, blood pH can be shifted up or down by these two systems. Respiratory Regulation of Acid-Base Balance CO2 + H2O Û H2CO3 Û H+ + HCO3- ¯ ventilation will [CO2] in ECF, [H+], ¯ pH (primary respiratory acidosis) ventilation will ¯ [CO2] in ECF, ¯ [H+], pH (primary respiratory alkalosis) Feedback Control of [H+] by the Respiratory System CO2 + H2O Û H2CO3 Û H+ + HCO3- * The overall ‘buffering power’ of the respiratory system is 1-2x as great as that of all the chemical buffers combined. [min-hr] Renal Regulation of Acid-Base Balance CO2 + H2O Û H2CO3 Û H+ + HCO3- Changes in pH (i.e. [H+]) are buffered by tubular secretion of H+ ions accompanied by HCO3- uptake. (days) Mechanisms for H+ secretion: Secondary Active Transport in PT & TAL Primary Active Transport in late DT/CCD Associated with HCO3- absorption. Reabsorption of filtered HCO3- in PT No HCO3- transporter: Na+/H+-countertransport (2nd active transport) Counter-transport of Na+ into cell (down an electrochemical gradient generated by the Na-K- ATPase pump) and H+ out of cell and into tubule Combination of H+ with HCO3- in tubules to form carbon dioxide and water [CA]; the former diffuses into cell and process is reversed to regenerate H+ and Na+ HCO3- intracellularly HCO3- is transported out of the PT cell in symport with Na+; H+ combines with another HCO3- or is PT buffered by HPO4- ion Reabsorption of HCO3- in late DT & CD (I cells) H+-ATPase and H+-K+-ATPase (1st active transport) In acidosis: primary active secretion of H+ from intercalated cells of late DT & CD. Note that 1 HCO3- ion is generated for every H+ secreted, and acts as a buffer to lower H+. In alkalosis: decrease in H+ secretion increases the renal excretion of HCO3- ion. High [H+] à é Aldosterone stimulates the secretion of H+ by the I cells. Late DT / CD Chronic Renal Failure Retention of salt and water ® (pitting) oedema, hypertension, hypo/hypernatraemia (weakness, fatigue) Hyperkalaemia Metabolic acidosis ® coma, and death when pH ~6.8 Uraemia : urea, uric acid, creatinine (pruritus; neurologic complications; coagulopathies – bruising, bleeding) Anaemia (partially because of ¯ EPO production by kidneys) Osteomalacia (weakening of bones) - reduced availability of calcium to bone. Chronic Renal Failure - Causes Diabetes and high blood pressure are controllable conditions and thus renal failure can be avoided with correct treatment Causes damages to arteries Other: congenital anomalies, kidney stones, chronic UTIs, tumors