Oxidative Phosphorylation PDF
Document Details
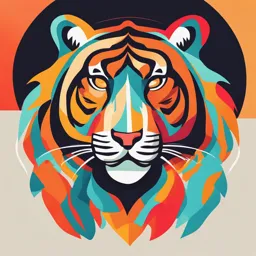
Uploaded by EffortlessCello8490
Ross University School of Medicine
Anthony Lyons PhD
Tags
Summary
This document provides an overview of oxidative phosphorylation, including learning objectives, the role of mitochondria in energy generation, and the concept of Gibbs free energy. It also discusses the coupling of reactions and common intermediates, including ATP.
Full Transcript
Oxidative Phosphorylation Anthony Lyons PhD [email protected] Learning Objectives 1. Understand the central role of the mitochondria in energy generation. 2. Describe Gibbs free energy and how it affects biochemical reactions. 3. Describe how endergonic reactions take place and the role played by...
Oxidative Phosphorylation Anthony Lyons PhD [email protected] Learning Objectives 1. Understand the central role of the mitochondria in energy generation. 2. Describe Gibbs free energy and how it affects biochemical reactions. 3. Describe how endergonic reactions take place and the role played by coupling to favorable processes 4. Describe the process of oxidative phosphorylation and the production of ATP within the mitochondria. 5. Identify inhibitors of the electron transport chain and their specific targets. 6. Understand uncoupling and describe its role in normal homeostasis. 7. List the inhibitors and uncouplers of electron transport, the biochemical derangements they cause, and possible treatment approaches. Goalof oxidativephosphorylation stake NASHand Fitbittomake Oxidative phosphorylation and electron Bioenergetics transport chain are basically the same thing Simplified Overview of Metabolism When foodstuffs are digested, they are broken down into units that are less complex than their parent compounds. Polysaccharides are broken down into monosaccharides such as glucose, proteins into amino acids and fats into glycerol and fatty acids. Digestion can be thought of as the first stage of energy generation. The potential energy that is CoA CO2 H20 Proteins Deamination contained in the food molecules is transferred into Complex Carbohydrates Glycolysis Acetyl CoA Citric Acid (TCA) Cycle a form which can be used by the body to fuel its various Fatty acid Triglycerides Electron and H+ carriers energy requiring activities. Oxidation O2 ATP Digestion Electron Transport Chain ADP H20 7 Gibbs Free Energy Free Energy Is the Useful Energy in a System Bioenergetics is the study of the energy changes accompanying biochemical reactions. can be obtained from a reaction at constant temperature and pressure. : B A If there is a greater concentration of B than of A at equilibrium, i.e. Keq > 1, the – that is, the reaction tends to move forward from a standard state in which A and B are present at equal concentrations. In this case, the reaction is said to be a spontaneous or exergonic reaction, and the free energy of this reaction is defined as negative: indicates that energy is liberated by the reaction. Gibbs Free Energy If the concentration of A is greater than that of B at equilibrium, the forward reaction is termed unfavorable, nonspontaneous or endergonic, and the reaction has a positive free energy: that is, when starting concentrations are equal, B tends to form A, rather than A to form B. forward from its equilibrium position to the standard state in which A and B are present at equal concentrations. The total free energy available from a reaction depends on both its (moles) of reactant converted to product. The units of free energy are Reactions can be classified according to the kcal/mol (kJ/mol). change in the free energy of the reaction: Endergonic - NON- Exergonic - How Do Endergonic Reactions Happen? Every metabolic pathway, overall, must be thermodynamically favoured (- G). In some cases reactions with a + G° proceed because the concentration of reactants and products are maintained far from equilibrium Reactants are maintained at high levels Products are constantly used up in other reactions In a lot of cases energy needed to drive endergonic reactions comes from coupling to exergonic reactions. Note: Standard free energies (at a concentration of 1M (mol/L) at 25°C) are represented by the symbol ° ° Coupling of Endergonic to Exergonic Reactions A is converted to B with release of free energy (exergonic). Some of this energy is used for the endergonic conversion of C to D The remaining energy is lost as heat The overall net change is exergonic. An extension of the coupling concept is provided by dehydrogenation reactions. These are coupled to hydrogenations by an intermediate carrier Free Energy is Additive in Coupled Reactions G, G° and G'° are additive in any sequence of consecutive reactions. if Go energy is equal to the sum of the free energies of each step A reaction pathway may occur spontaneously (- Remember: The free energy only indicates whether or not a reaction is possible. The rate at which reactions occur is determined by Kinetics Common intermediates In biochemistry, energy coupling occurs when the energy-requiring and the energy-yielding reactions share a common intermediate. D is the common intermediate 858 Common intermediates may be high-energy molecules May be formed from the energy of hydrolysis of high-energy molecules Example - High-energy phosphate compounds: ATP, phosphoenolpyruvate, creatine phosphate, etc. Sometimes they may not even be molecular Example - H+ gradient across mitochondrial membrane in the electron transport chain Question Assume standard biological conditions and that all the enzymes are present to catalyze the reactions. Given the following data: -6- o 2O, 2 I o - Calculate the o whether the reaction is spontaneous -- -6- A. –10.6 kcal/mol and spontaneous B. +10.6 kcal/mol and not spontaneous C. –4.0 kcal/mol and not spontaneous D. +4.0 kcal/mol and not spontaneous Mechanical model of the coupling of favorable and unfavorable processes. Figure 6.4 - Lippincott Illustrated OE. –4.0 kcal/mol and spontaneous Reviews: Biochemistry, 7e High-Energy Phosphates Act as the "Energy Currency" of the Cell ATP is able to act as a donor of high- energy phosphate to form those compounds below it in. Likewise, with the necessary enzymes, ADP can accept high-energy phosphate to form ATP from those compounds above 95g ATP in the table. Eh Sources of Phosphates A net formation of two P results from the formation of lactate from one molecule of glucose. One P is generated directly in the cycle The greatest quantitative source of P in aerobic organisms. 901 Cl 03 y Free energy comes from respiratory 4 chain oxidation using molecular O2 within mitochondria. www.khanacademy.org/science/ The transduction of energy from reduced coenzymes to ATP The major redox coenzymes involved in transduction of energy from fuels to ATP are nicotinamide adenine dinucleotide (NAD+), flavin adenine dinucleotide (FAD) and Flavin mononucleotide (FMN) During energy metabolism, electrons are transferred from carbohydrates and fats to these coenzymes. Forms energy-rich reduced coenzymes, NADH + H+ and FADH2 t t NADHand FADHL donate electors aremale t Amino acids baseand FAD Carbohydrates leadingto itbeing Fatty acids Stages of fuel oxidation. able - Fig. 9.1 Medical Biochemistry (ClinicalKey)to male Atf I C Adenosine triphosphate (ATP) These are the ve types of activities ATP is used for H is energeticallyexpensive to make AA The free energy that is released by hydrolysis of ATP is used to fuel five types of activities in the body; i. Synthesis of macromolecules ii. Active transport of ions Égf 1 molecule of GTP is used nearest iii. Themogenesis in each translation cycle iv. Contraction of muscles v. Detoxification FIG. 19.1. - Hydrolysis of other phosphorylated nucleotides is also used to provide energy for endergonic reactions (but not to the extent that ATP is used). Example - During the elongation stage of translation, GTP is used as an energy source for the binding of a new amino-bound tRNA to the A site of the ribosome. Mitochondrial ATP production Oxidative phosphorylation is the mechanism by which energy derived from fuel oxidation is conserved in the form of ATP. As the electron transport chain shuttles electrons through its machinery, hydrogen ions are drawn from the mitochondrial matrix and deposited in the intermembrane space, creating an poster sym electrochemical gradient. the proton- Egg Electron transport chain main goal - couple energy stored in electron acceptors to a proton gradient that Mitochondrial ATP production drives ATP synthesis ATP synthase - aka chemiosmosis Oxidative phosphorylation is the mechanismThebycombination whichof electron energy transport chain and chemiosmosis is oxidative derived Fuels(glucose) from enter fuel mitochondria oxidation via transporters is conserved and convert to in the form of ATP. phosphorylation pyruvate/fatty acids. As the electron transport chain shuttles Pyruvate then is turned into acetyl COa goes through TCA. electrons through its machinery, hydrogen The TCA ionsproduces are NADH, drawn FADH2, fromand electrons. These get fed into the mitochondrial proton pumps. matrix and deposited in the Theseintermembrane space,ionscreating proton pumps pump out hydrogen an into the Intermembrane space, creating a electrochemical gradient. electrochemical gradient. The hydrogen ions activate ATP synthase which makes ATP. the proton- Oxidative Phosphorylation Depends on Electron Transfer In oxidative phosphorylation, Standard reduction potentials of some reactions the electron-transfer potential of NADH or FADH2 is convertedi into the phosphoryl-transfer potential of ATP. The reduction potential of a compound refers to its tendency to acquire electrons and thereby to be reduced. A strong reducing agent is poised to donate electrons - has a negative reduction potential A strong oxidizing agent (such as O2) is ready to - has a positive reduction potential. Oxidative Phosphorylation l Step 1 - TCA creates NADH and FADH2. These enter electron Transport chain starting with Complex 1. At complex 1, NADH donates its electrons to complex 1 and becomes NAD+. When those electrons are donated to complex 1 it gets supercharged. When it gets supercharged, it now has the energy to pump the proton (H+) from the mitochondrial matrix into the intermembrane space. As it does this it pumps more and more hydrogen protons into the Intermembrane space, which leads to the accumulation of protons on the other side of the membrane, slowly forming a proton gradient. Step 2 - Complex 1 will now pass its electrons to Coenzyme Q. At Complex 2, FADH2 donates its electrons to complex 2 and turns into FAD. Complex 2 cannot however become supercharged and pump protons from matrix into Intermembrane space. This forces Complex 2 to pass its electrons to Coenzyme Q as well. CoEnzyme Q is the common electron acceptor from coenzyme 1 and coenzyme 2. Step 3 - the electrons are now sitting in Coenzyme Q and are now passed to complex 3. Complex 3 now gets supercharged and has enough energy to pump protons (H+) from mitochondrial matrix into the Intermembrane space (just like complex 1). Protein Gradient is continuing to be formed. Complex 3 will now pass its electrons on to cytochrome C. At cytochrome C it now passes its electrons to complex 4. Complex 4 now gets supercharged and has enough energy to pump protons (H+) from mitochondrial matrix in Intermembrane space, Proton Gradient (an imbalance between proton concentration in Intermembrane space and mitochondrial matrix) Step 4 - Complex 4 passes it’s electrons to the nal electron acceptor, which is oxygen, which splits into two oxygen ions. protons (H+) are added to the two oxygen ions creating two water molecules (H20). This is the end of electron transport chain, which has formed a massive proton gradient ( large amount of hydrogen ions in Intermembrane space compared to matrix). Step 5 - ATP synthase makes use of this proton gradient to generate massive amounts of ATP. The way this is done is an ADP molecule approaches ATP synthase. ATP synthase takes advantage of how molecules in general want to ow from high energy states to low energy states and travel down their concentration gradient. Hence Hydrogen ions are constantly trying to come back into the matrix from the Intermembrane space to achieve equilibrium. When Protons (H+) ow down into the Matrix, ATP synthase uses the proton to convert ADP to ATP. Protons are continually owing down hill making massive amount of ATP. Strongoxidisingagent like Electron Transport Chain oxygenreadyto take electrons The mitochondrial electron transport chain transfers electrons in a defined multi-step sequence from reduced nucleotides to oxygen. The entire electron transport NAE system, also known as the electron transport chain or respiratory chain, is. The chain consists of a number of protein complexes, designated as I through IV. Electron Transport Chain gig Electrons flow through the respiratory chain through a redox span of 1.1 V from NAD+/NADH to O2/2H2O passing through three large protein complexes: - g I), where electrons are transferred Yotam from NADH to coenzyme Q (Q) (also called ); coenzyme Q- c g which passes the electrons on to cytochrome c; and c IV), which completes the chain, passing the electrons to O2 and causing it to be reduced to H2O g 59896 g M Electron Transport Chain Iggy complexit does not pump anyprotonshoweverit transfers e fo coenzyme Q 2, produced by reactions such as the oxidation of succinate to fumarate, enter the electron transport chain. Complex II will transfer electrons to , without the associated proton pumping across the inner mitochondrial membrane. The production of ATP is coupled to the transfer of electrons through the electron transport chain to O2. The overall process is known as oxidative phosphorylation. FIG. 21.5. Components of the electron-transport chain. need Ubiquinone (coenzyme Q10) gangreieptor small up id solublecompound Named because it is ubiquitous in virtually all living systems. It is a small lipid-soluble compound found in the inner membrane of animal and plant mitochondria and in the plasma membrane of bacteria. The primary form of mammalian ubiquinone contains a side chain of 10 isoprene units and is often called CoQ10. It diffuses within the inner membrane, accepts electrons (from the four major mitochondrial flavoproteins), and transfers them to complex III (QH2 –cytochrome c reductase). Ubiquinone can carry either one or two electrons and is also thought to be a major source of superoxide radicals in the cell No coenzyme Q makes it dif cult to make ATP Case hence causing weakness A rare coenzyme Q10 deficiency A 4-year-old boy presented with seizures, progressive muscle weakness, and encephalopathy. Accumulation of lactate, a product of anaerobic metabolism of glucose, in the cerebrospinal fluid (CSF) suggested a defect in mitochondrial oxidative metabolism. Muscle mitochondria were isolated for study. The activities of the individual complexes I, II, III, and IV were normal, but the combined activities of I + III and II + III were significantly decreased. Treatment with coenzyme Q 10 improved the muscle weakness but not the encephalopathy. Brain The decreased activities of complexes I + III and II + III suggested a deficiency in coenzyme Q10 , which was confirmed by direct measurements. NIH - Primary coenzyme Q10 deficiency alwaysdownhill Electron Transport Chain Electrons are transferred from NADH to O2 through a chain of three large protein complexes called NADH-Q oxidoreductase, Q-cytochrome c oxidoreductase, and cytochrome c oxidase. Electron flow within these transmembrane complexes is highly exergonic and powers the transport of protons across the inner mitochondrial membrane. Electrons flow down an energy gradient Caskjudeneandlaredwhathelaidt Transfer of Electrons from NADH into Mitochondria Electron shuttles are required for mitochondrial oxidation of NADH produced in the cytoplasmic compartment Outside of the TCA cycle, this is another way NADH enters the mitochondrial matrix Cytoplasmic glycerol-3-P Ston Essig dehydrogenase catalyzes reduction of dihydroxyacetone-P (DHAP) with NADH to glycerol-3-P, regenerating NAD+. The cytoplasmic glycerol-3- phosphate is oxidized back to DHAP by another glycerol-3- phosphate dehydrogenase isoform facing the outer surface of the inner mitochondrial membrane; This enzyme is a flavoprotein in which FAD is reduced to FADH2. Transfer of Electrons - Many cells, e.g. in skeletal muscle, use the glycerol-3- P shuttle, but heart and liver rely on the malate–aspartate shuttle. The substrate, malate, can cross the inner mitochondrial membrane, but the membrane is impermeable to the product, oxaloacetate. The exchange is therefore accomplished by interconversion between -keto- and -amino acids. This involves cytoplasmic and mitochondrial glutamate and - ketoglutarate, and isozymes of glutamate-oxaloacetate transaminase. bread oh andmitochondria ATP Generation A molecular assembly in the inner mitochondrial membrane carries out the synthesis of ATP. Protons flow down their electrochemical gradient through the membrane-bound ATP synthase. How is the oxidation of NADH coupled to the phosphorylation of ADP? ATP synthase complex. Fig. 9.11 – Medical Biochemistry ATP Synthase The enzyme complex consists of an F0 subcomplex which is a disk of "C" protein subunits. Attached is a subunit in the form of a "bent axle." Protons passing through the disk of "C" units ADP and Pi are taken up by the subunits of the F1 subcomplex to form ATP. conformation. ATP Generation This is the amount of ATP that can be made from one NADH AND FADH Each mole of NADH oxidized leads to 10 moles of protons being extruded from the matrix. For every mole of NADH that is oxidized, 2 mole of O2 is reduced to H2O. As it takes four protons to flow through the ATPase to synthesize one ATP,. For every mole of FADH2 that is oxidized, approximately are generated. Uncoupling Proteins (UCP) This stops oxidative phosphorylation Uncouplers of oxidative phosphorylation dissipate the proton gradient by transporting protons back into mitochondria, bypassing the ATP synthase. , 2,4- Clinical Correlation: Malignant hyperthermia can result from inhalation anesthetics. The major inhalation anesthetics (halothane, ether, and methoxyflurane) trigger a reaction in susceptible people that results in the uncoupling of oxidative phosphorylation from electron transport. ATP production decreases; heat is generated; and the temperature rises markedly. The TCA cycle is stimulated, and excessive CO2 production leads to respiratory acidosis. Uncoupling proteins stops oxidative phosphorylation Uncoupling Proteins (UCP) However, there is one particular uncoupled By reaction that occurs clearly by design: s y I t. UCP1 is located in the inner mitochondrial transmembrane of brown adipocytes Allows protons in the mitochondrial intermembrane space to re-enter the mitochondrial matrix without generating ATP, that is, uncoupled, and heat is Tseng et al, Nature Review Drug Discovery Vol. 9, 2010 Adipose tissue can help with creating heat Adipose tissue is a dynamic organ which can Thermogenic Fat range from 4% to >40% of total body composition in adult humans. Fat is often noted for its energy-storing function, as in white adipose tissue (WAT); however, mammals also possess brown adipose tissue (BAT) that plays a crucial role in whole- body energy homeostasis through non-shivering thermogenesis. Emerging evidence suggests that mammals possess at least two types of thermogenic adipocytes:. In rodents and infants, classical brown adipocytes (or brown fat) are located in the dedicated BAT depots, such as the interscapular regions and around the kidney (perirenal BAT). Trends in Endocrinology & Metabolism, March 2018, Vol. 29, No. 3 https://doi.org/10.1016/j.tem.2018.01.001 Beige adipocytes (also called brite adipocytes) are an inducible form of thermogenic adipocytes that sporadically reside within WAT depots. Similarly to brown adipocytes, beige adipocytes also possess abundant cristae-dense mitochondria that express UCP1 and multilocular lipid droplets Hormonal control of browning needto you how know Metabolic adaptations to tocovet environmental factors - whitefatto cold, or pourtout metabolic conditions, including exercise, fasting, feeding and obesity, regulated by the release of endocrine and paracrine factors from metabolically active organs. In response to cold, catecholamines released by the sympathetic nervous system (and possibly also tissue-resident M2 macrophages) are of key importance for the activation of energy-sensing pathways in white adipocytes and beige adipocyte precursors. Bartelt, A. & Heeren, J. Nat. Rev. Endocrinol. advance online publication 22 October 2013; exercised cads brown fat prude Uncoupling Proteins (UCP) you cutey Four additional uncoupling proteins are expressed by the human genome: UCP2, UCP3, UCP4, and UCP5. Whereas UCP1 is exclusive to brown adipose tissue, UCP2 is expressed ubiquitously, UCP3 is mainly expressed in skeletal muscle, and UCP4 and UCP5 are expressed in the brain. Except for UCP1, the physiologic functions of these proteins are not well understood, but they could be of profound significance in our understanding of such health issues as , cancer, thyroid disease, and aging. Clinical Correlation: There is strong evidence that obesity induces the synthesis of UCP2 -cells of the pancreas. -cell dysfunction found in type 2 diabetes because it lowers the intracellular concentration of ATP, which is required for secretion of insulin. The common fever that is induced by infectious organisms is probably (partially) also due to uncoupling by UCPs. Inhibitors of electron transport and oxidative phosphorylation If there is a block at any point in the electron transport chain, all carriers before the block will accumulate in their reduced states, whereas those after the block will accumulate in their oxidized states. As a result, O2 will not be consumed; ATP will not be generated; and the TCA cycle will slow down owing to the accumulation of NADH. i P DHT lactatebuildup Rotenone, complexes with complex I, causing NADH to accumulate. It does not block the transfer of electrons to the chain from FADH2. (antibiotics) block the passage of electrons through the 0cytochrome b-c1 complex (complex III). Inhibitors of Electron Transport , combine with cytochrome oxidase (complex IV) and block the transfer of electrons to O2. Cyanide poisoning is due to cyanide binding to Fe3+ in cytochrome aa3. As a result, O2 cannot receive electrons; respiration is inhibited; energy production is halted; and death occurs rapidly. Cyanide and carbon monoxide also bind to hemoglobin, blocking oxygen binding and transport. In these poisonings, both the ability to transport oxygen and the ability to synthesize ATP are impaired.