NURS 1109 - Cells Part 1 PDF
Document Details
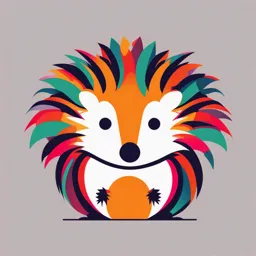
Uploaded by EnhancedMolybdenum
Dr. Pamela Paynter-Armour
Tags
Summary
This document provides an overview of cells, discussing cell theory and the differences between prokaryotic and eukaryotic cells. It covers cell structures, plasma membranes, and membrane transport, including active and passive processes. The text also outlines the importance of osmosis and tonicity in cell function.
Full Transcript
The Cell By: Dr. Pamela Paynter-Armour Objectives 1. Discuss the Cell Theory 2. Describe the general Cell Structure 3. Describe the plasma Membrane 4. Identify the membrane junctions 5. Outline the movements Into and Out of the Cell History of Cell T...
The Cell By: Dr. Pamela Paynter-Armour Objectives 1. Discuss the Cell Theory 2. Describe the general Cell Structure 3. Describe the plasma Membrane 4. Identify the membrane junctions 5. Outline the movements Into and Out of the Cell History of Cell Theory mid 1600s – Anton van Leeuwenhoek Improved microscope, observed many living cells mid 1600s – Robert Hooke Observed many cells 1850 – Rudolf Virchow Proposed that all cells come from existing cells Cell Theory Cells were discovered in 1665 by Robert Hooke. Early studies of cells were conducted by - Mathias Schleiden (1838) - Theodor Schwann (1839) Schleiden and Schwann proposed the Cell Theory. 6 4 The Cell Theory The cell theory (proposed independently in 1838 and 1839) is a cornerstone of biology. All organisms are composed of one or more cells. Schleiden Cells are the smallest living things. Cells arise only by division of previously existing cells. All organisms living today are descendants of an ancestral cell. ³ Schwann Cell Theory The cell is the smallest structural and functional living unit Organismal functions depend on individual and collective cell functions Biochemical activities of cells are dictated by their specific subcellular structures Continuity of life has a cellular basis¹ Two Fundamentally Different Types of Cells A prokaryotic cell A eukaryotic cell Prokaryotic Do not have structures surrounded by membranes Few internal structures One-celled organisms, Bacteria⁴ Eukaryotic Contain organelles surrounded by membranes Most living organisms ⁴ Plant Animal Us vs. Them - Eukaryotes and Prokaryotes³ Definition of Cell A cell is the smallest unit that is capable of performing life functions.6 Examples of Cells Amoeba Proteus Plant Stem Bacteria Red Blood Cell Nerve Cell Cell Diversity Over 200 different types of human cells Types differ in size, shape, subcellular components, and functions¹ Erythrocytes Fibroblasts Epithelial cells (a) Cells that connect body parts, Nerve cell form linings, or transport gases Skeletal Smooth (e) Cell that gathers information Muscle muscle cells and control body functions cell (b) Cells that move organs and Sperm body parts Macrophage (f) Cell of reproduction Fat cell (c) Cell that stores (d) Cell that nutrients fights disease Generalized Cell All cells have some common structures and functions Human cells have three basic parts: Plasma membrane—flexible outer boundary Cytoplasm—intracellular fluid containing organelles Nucleus—control center¹ Chromatin Nuclear envelope Nucleolus Nucleus Smooth endoplasmic reticulum Plasma Mitochondrion membrane Cytosol Lysosome Centrioles Centrosome matrix Rough endoplasmic reticulum Ribosomes Golgi apparatus Secretion being Cytoskeletal released from cell elements by exocytosis Microtubule Intermediate filaments Peroxisome Figure 3.2 Plasma Membrane Bimolecular layer of lipids and proteins in a constantly changing fluid mosaic Plays a dynamic role in cellular activity Separates intracellular fluid (ICF) from extracellular fluid (ECF) Interstitial fluid (IF) = ECF that surrounds cells ¹ Extracellular fluid (watery environment) Polar head of Cholesterol phospholipid Glycolipid molecule Glycoprotein Carbohydrate of glycocalyx Outward- Integral facing proteins layer of Filament of phospholipids cytoskeleton Inward-facing Bimolecular Peripheral layer of lipid layer proteins phospholipids containing Nonpolar proteins tail of phospholipid Cytoplasm molecule (watery environment) Membrane Lipids 75% phospholipids (lipid bilayer) Phosphate heads: polar and hydrophilic Fatty acid tails: nonpolar and hydrophobic 5% glycolipids Lipids with polar sugar groups on outer membrane surface 20% cholesterol Increases membrane stability and fluidity¹ Membrane Proteins Integral proteins Firmly inserted into the membrane (most are transmembrane) Functions: Transport proteins (channels and carriers), enzymes, or receptors¹ Membrane Proteins Peripheral proteins Loosely attached to integral proteins Include filaments on intracellular surface and glycoproteins on extracellular surface Functions: Enzymes, motor proteins, cell-to-cell links, provide support on intracellular surface, and form part of glycocalyx¹ Extracellular fluid (watery environment) Polar head of Cholesterol phospholipid Glycolipid molecule Glycoprotein Carbohydrate of glycocalyx Outward- Integral facing proteins layer of Filament of phospholipids cytoskeleton Inward-facing Bimolecular Peripheral layer of lipid layer proteins phospholipids containing Nonpolar proteins tail of phospholipid Cytoplasm molecule (watery environment) Figure 3.3 Functions of Membrane Proteins 1. Transport 2. Receptors for signal transduction 3. Attachment to cytoskeleton and extracellular matrix¹ (a) Transport A protein (left) that spans the membrane may provide a hydrophilic channel across the membrane that is selective for a particular solute. Some transport proteins (right) hydrolyze ATP as an energy source to actively pump substances across the membrane. ¹ (b) Receptors for signal transduction Signal A membrane protein exposed to the outside of the cell may have a binding site with a specific shape that fits the shape of a chemical messenger, such as a hormone. The external signal may cause a change in shape in the protein that initiates a chain of chemical Receptor reactions in the cell. ¹ (c) Attachment to the cytoskeleton and extracellular matrix (ECM) Elements of the cytoskeleton (cell’s internal supports) and the extracellular matrix (fibers and other substances outside the cell) may be anchored to membrane proteins, which help maintain cell shape and fix the location of certain membrane proteins. Others play a role in cell movement or bind adjacent cells together. Functions of Membrane Proteins 4. Enzymatic activity 5. Intercellular joining 6. Cell-cell recognition¹ (d) Enzymatic activity Enzymes A protein built into the membrane may be an enzyme with its active site exposed to substances in the adjacent solution. In some cases, several enzymes in a membrane act as a team that catalyzes sequential steps of a metabolic pathway as indicated (left to right) here. (e) Intercellular joining Membrane proteins of adjacent cells may be hooked together in various kinds of intercellular junctions. Some membrane proteins (CAMs) of this group provide temporary binding sites that guide cell migration and other cell-to-cell interactions. CAMs (f) Cell-cell recognition Some glycoproteins (proteins bonded to short chains of sugars) serve as identification tags that are specifically recognized by other cells. Glycoprotein Membrane Junctions Three types: Tight junction Desmosome Gap junction¹ Membrane Junctions: Tight Junctions Prevent fluids and most molecules from moving between cells Where might these be useful in the body? ¹ Plasma membranes Microvilli of adjacent cells Intercellular space Basement membrane Interlocking junctional proteins Intercellular space (a) Tight junctions: Impermeable junctions prevent molecules from passing through the intercellular space. Membrane Junctions: Desmosomes “Rivets” or “spot-welds” that anchor cells together Where might these be useful in the body? ¹ Plasma membranes Microvilli of adjacent cells Intercellular space Basement membrane Intercellular space Plaque Intermediate Linker glycoproteins filament (keratin) (cadherins) (b) Desmosomes: Anchoring junctions bind adjacent cells together and help form an internal tension-reducing network of fibers. Membrane Junctions: Gap Junctions Transmembrane proteins form pores that allow small molecules to pass from cell to cell For spread of ions between cardiac or smooth muscle cells ¹ Plasma membranes Microvilli of adjacent cells Intercellular space Basement membrane Intercellular space Channel between cells (connexon) (c) Gap junctions: Communicating junctions allow ions and small mole- cules to pass from one cell to the next for intercellular communication. Why Are Cells So Small? ✓Cells need sufficient surface area to allow adequate transport of nutrients in and wastes out. ✓As cell volume increases, so does the need for the transporting of nutrients and wastes. 6 Why Are Cells So Small? However, as cell volume increases the surface area of the cell does not expand as quickly. If the cell’s volume gets too large it cannot transport enough wastes out or nutrients in. Thus, surface area limits cell volume/size. Why Are Cells So Small? Strategies for increasing surface area, so cell can be larger: “Frilly” edged……. Long and narrow….. Round cells will always be small. Membrane Transport Plasma membranes are selectively permeable Some molecules easily pass through the membrane; others do not¹ Types of Membrane Transport Passive processes No cellular energy (ATP) required Substance moves down its concentration gradient Active processes Energy (ATP) required Occurs only in living cell membranes¹ Passive Processes What determines whether or not a substance can passively permeate a membrane? 1. Lipid solubility of substance 2. Channels of appropriate size 3. Carrier proteins¹ Passive Processes Simple diffusion Carrier-mediated facilitated diffusion Channel-mediated facilitated diffusion Osmosis¹ Passive Transport Simple Diffusion – kinetic energy is everywhere - allows mixing or diffusion – diffusion requires a concentration gradient high concentration in one area, lower concentration in another if areas are continuous (connected), particles move with (down) the concentration gradient eventually it reaches equal concentration everywhere – equilibrium (Pitts, Schiller, & Thompson, 2010). equilibrium Passive Processes: Simple Diffusion Nonpolar lipid-soluble (hydrophobic) substances diffuse directly through the phospholipid bilayer¹ Extracellular fluid Lipid- soluble solutes Cytoplasm (a) Simple diffusion of fat-soluble molecules directly through the phospholipid bilayer Passive Processes: Facilitated Diffusion Certain lipophobic molecules (e.g., glucose, amino acids, and ions) use carrier proteins or channel proteins, both of which: Exhibit specificity (selectivity) Are saturable; rate is determined by number of carriers or channels Can be regulated in terms of activity and quantity ¹ Facilitated Diffusion Using Carrier Proteins Transmembrane integral proteins transport specific polar molecules (e.g., sugars and amino acids) Binding of substrate causes shape change in carrier ¹ Lipid-insoluble solutes (such as sugars or amino acids) (b) Carrier-mediated facilitated diffusion via a protein carrier specific for one chemical; binding of substrate causes shape change in transport protein Facilitated Diffusion Using Channel Proteins Aqueous channels formed by transmembrane proteins selectively transport ions or water Two types: Leakage channels Always open Gated channels Controlled by chemical or electrical signals¹ Small lipid- insoluble solutes (c) Channel-mediated facilitated diffusion through a channel protein; mostly ions selected on basis of size and charge Factors Affection Diffusion 1. Increased temperature increases diffusion rate 2. Greater concentration gradients increase diffusion rate 3. Larger surface area increases diffusion rate 4. Smaller particle sizes increase diffusion rate 5. Time - diffusion decreases as concentrations equalize⁵ Osmosis The diffusion of water from an area of higher [H2O] to lower [H2O] Water concentration = 1/solute concentration Polar water molecules move through aquaporin channels (AQP) Or (perhaps) “wiggle” through phospholipids ⁵ Passive Processes: Osmosis Movement of solvent (water) across a selectively permeable membrane Water diffuses through plasma membranes: Through the lipid bilayer Through water channels called aquaporins (AQPs) ¹ Water molecules Lipid billayer Aquaporin (d) Osmosis, diffusion of a solvent such as water through a specific channel protein (aquaporin) or through the lipid bilayer Figure 3.7d Passive Processes: Osmosis Water concentration is determined by solute concentration because solute particles displace water molecules Osmolarity: The measure of total concentration of solute particles When solutions of different osmolarity are separated by a membrane, osmosis occurs until equilibrium is reached ¹ (a) Membrane permeable to both solutes and water Solute and water molecules move down their concentration gradients in opposite directions. Fluid volume remains the same in both compartments. Left Right Both solutions have the compartment: compartment: same osmolarity: volume Solution with Solution with unchanged lower osmolarity greater osmolarity H2 O Solute Solute molecules Membrane (sugar) (b) Membrane permeable to water, impermeable to solutes Solute molecules are prevented from moving but water moves by osmosis. Volume increases in the compartment with the higher osmolarity. Both solutions have identical osmolarity, but volume of the solution on the right is greater Left Right because only water is compartment compartment free to move H2 O Solute molecules Membrane (sugar) Importance of Osmosis When osmosis occurs, water enters or leaves a cell Change in cell volume disrupts cell function¹ Tonicity Tonicity: The ability of a solution to cause a cell to shrink or swell Isotonic: A solution with the same solute concentration as that of the cytosol Hypertonic: A solution having greater solute concentration than that of the cytosol Hypotonic: A solution having lesser solute concentration than that of the cytosol¹ (b) Hypertonic solutions (c) Hypotonic solutions (a) Isotonic solutions Cells take on water by osmosis until Cells retain their normal size and Cells lose water by osmosis and shrink in a hypertonic solution they become bloated and burst (lyse) shape in isotonic solutions (same (contains a higher concentration in a hypotonic solution (contains a solute/water concentration as inside of solutes than are present inside lower concentration of solutes than cells; water moves in and out). are present in cells). the cells). Summary of Passive Processes Process Energy Example Source Simple Kinetic energy Movement of O2 through diffusion phospholipid bilayer Facilitated Kinetic energy Movement of glucose into cells diffusion Osmosis Kinetic energy Movement of H2O through phospholipid bilayer or AQPs Membrane Transport: Active Processes Two types of active processes: Active transport Vesicular transport Both use ATP to move solutes across a living plasma membrane² Active Transport Requires carrier proteins (solute pumps) Moves solutes against a concentration gradient Types of active transport: Primary active transport Secondary active transport² Primary Active Transport Energy from hydrolysis of ATP causes shape change in transport protein so that bound solutes (ions) are “pumped” across the membrane² Primary Active Transport Sodium-potassium pump (Na+-K+ ATPase) Located in all plasma membranes Involved in primary and secondary active transport of nutrients and ions Maintains electrochemical gradients essential for functions of muscle and nerve tissues² Ext rac ellula r fluid Na+ Na+-K+ pum p K+ Na+ bound ATP-binding s ite Cytoplasm 1 Cytoplasm ic Na+ binds to pum p protein. P ATP K+ released ADP 6 K+ is releas ed from the pump prote in 2 Binding of Na+ promotes and Na+ sites are re ady to bind Na+ again. phosphorylation of the protein by ATP. The cy cle repeats. Na+ released K+ bound P Pi K+ 5 K+ binding t riggers rele ase of the 3 Phosphory lat ion ca use s t he protein t o phosphate. P um p protein returns to its change shape, e xpe lling Na + to the outside. original conformation. P 4 Ext rac ellula r K+ binds to pum p protein. Extracellular fluid Na+ Na+-K+ pump ATP-binding site K+ Cytoplasm 1 Cytoplasmic Na+ binds to pump protein. Na+ bound P ATP ADP 2 Binding of Na+ promotes phosphorylation of the protein by ATP. Na+ released P 3 Phosphorylation causes the protein to change shape, expelling Na + to the outside. K+ P 4 Extracellular K+ binds to pump protein. K+ bound Pi 5 K+ binding triggers release of the phosphate. Pump protein returns to its original conformation. K+ released 6 K+ is released from the pump protein and Na+ sites are ready to bind Na+ again. The cycle repeats. Ext rac ellula r fluid Na+ Na+-K+ pum p K+ Na+ bound ATP-binding s ite Cytoplasm 1 Cytoplasm ic Na+ binds to pum p protein. P ATP K+ released ADP 6 K+ is releas ed from the pump prote in 2 Binding of Na+ promotes and Na+ sites are re ady to bind Na+ again. phosphorylation of the protein by ATP. The cy cle repeats. Na+ released K+ bound P Pi K+ 5 K+ binding t riggers rele ase of the 3 Phosphory lat ion ca use s t he protein t o phosphate. P um p protein returns to its change shape, e xpe lling Na + to the outside. original conformation. P 4 Ext rac ellula r K+ binds to pum p protein. Secondary Active Transport Depends on an ion gradient created by primary active transport Energy stored in ionic gradients is used indirectly to drive transport of other solutes² Secondary Active Transport Cotransport—always transports more than one substance at a time Symport system: Two substances transported in same direction Antiport system: Two substances transported in opposite directions² Extracellular fluid Glucose Na+-glucose Na+-glucose symport symport transporter transporter releasing glucose loading into the cytoplasm Na+-K+ glucose from pump ECF Cytoplasm 1 The ATP-driven Na+-K+ pump 2 As Na+ diffuses back across the stores energy by creating a membrane through a membrane steep concentration gradient for cotransporter protein, it drives glucose Na+ entry into the cell. against its concentration gradient into the cell. (ECF = extracellular fluid) Extracellular fluid Na+-K+ pump Cytoplasm 1 The ATP-driven Na+-K+ pump stores energy by creating a steep concentration gradient for Na+ entry into the cell. Extracellular fluid Glucose Na+-glucose Na+-glucose symport symport transporter transporter releasing glucose loading into the cytoplasm Na+-K+ glucose from pump ECF Cytoplasm 1 The ATP-driven Na+-K+ pump 2 As Na+ diffuses back across the stores energy by creating a membrane through a membrane steep concentration gradient for cotransporter protein, it drives glucose Na+ entry into the cell. against its concentration gradient into the cell. (ECF = extracellular fluid) Resting Membrane Potential Generating/maintaining a resting membrane potential all cells are polarized negatively charged inside positively charged outside Na+/K+ ATPase creates the unequal charge distribution Na+ tends to diffuse inside on its own via leaky channels even more K+ tends to diffuse outside on its own via leaky channels the sodium-potassium pump transports 3 Na+ ions out & 2 K+ ions in with each cycle, using the energy from one ATP hydrolysis more negatively charged proteins (A-) are located within the cell These disequilibria in ions create the charge differential Electrochemical gradient the net effect of all charged ions on either side of the membrane Vesicular Transport Transport of large particles, macromolecules, and fluids across plasma membranes Requires cellular energy (e.g., ATP) ² Vesicular Transport Functions: Exocytosis—transport out of cell Endocytosis—transport into cell Transcytosis—transport into, across, and then out of cell Substance (vesicular) trafficking—transport from one area or organelle in cell to another² Endocytosis and Transcytosis Involve formation of protein-coated vesicles Often receptor mediated, therefore very selective² 1 Coated pit ingests Extracellular fluid substance. Plasma membrane Protein coat (typically Cytoplasm clathrin) 2 Protein- coated 3 Coat proteins detach vesicle and are recycled to detaches. plasma membrane. Transport vesicle Endosome Uncoated endocytic vesicle 4 Uncoated vesicle fuses with a sorting vesicle 5 Transport called an endosome. vesicle containing Lysosome membrane components moves to the plasma membrane for recycling. 6 Fused vesicle may (a) fuse with lysosome for digestion of its contents, or (b) deliver its contents to the plasma (b) (a) membrane on the opposite side of the cell (transcytosis). 1 Coated pit ingests Extracellular fluid substance. Plasma membrane Protein coat (typically Cytoplasm clathrin) 1 Coated pit ingests Extracellular fluid substance. Plasma membrane Protein coat (typically Cytoplasm clathrin) 2 Protein- coated vesicle detaches. 1 Coated pit ingests Extracellular fluid substance. Plasma membrane Protein coat (typically Cytoplasm clathrin) 2 Protein- coated 3 Coat proteins detach vesicle and are recycled to detaches. plasma membrane. 1 Coated pit ingests Extracellular fluid substance. Plasma membrane Protein coat (typically Cytoplasm clathrin) 2 Protein- coated 3 Coat proteins detach vesicle and are recycled to detaches. plasma membrane. Endosome Uncoated endocytic vesicle 4 Uncoated vesicle fuses with a sorting vesicle called an endosome. 1 Coated pit ingests Extracellular fluid substance. Plasma membrane Protein coat (typically Cytoplasm clathrin) 2 Protein- coated 3 Coat proteins detach vesicle and are recycled to detaches. plasma membrane. Transport vesicle Endosome Uncoated endocytic vesicle 4 Uncoated vesicle fuses with a sorting vesicle 5 Transport called an endosome. vesicle containing membrane components moves to the plasma membrane for recycling. 1 Coated pit ingests Extracellular fluid substance. Plasma membrane Protein coat (typically Cytoplasm clathrin) 2 Protein- coated 3 Coat proteins detach vesicle and are recycled to detaches. plasma membrane. Transport vesicle Endosome Uncoated endocytic vesicle 4 Uncoated vesicle fuses with a sorting vesicle 5 Transport called an endosome. vesicle containing Lysosome membrane components moves to the plasma membrane for recycling. 6 Fused vesicle may (a) fuse with lysosome for digestion of its contents, or (b) deliver its contents to the plasma (b) (a) membrane on the opposite side of the cell (transcytosis). Endocytosis Phagocytosis—pseudopods engulf solids and bring them into cell’s interior Macrophages and some white blood cells² (a) Phagocytosis The cell engulfs a large particle by forming projecting pseudopods (“false feet”) around it and enclosing it within a membrane sac called a phagosome. The phagosome is combined with a lysosome. Undigested contents remain in the vesicle (now called a residual body) or are ejected by exocytosis. Vesicle may or may not be protein- coated but has receptors Phagosome capable of binding to microorganisms or solid particles. Endocytosis Fluid-phase endocytosis (pinocytosis)—plasma membrane infolds, bringing extracellular fluid and solutes into interior of the cell Nutrient absorption in the small intestine² (b) Pinocytosis The cell “gulps” drops of extracellular fluid containing solutes into tiny vesicles. No receptors are used, so the process is nonspecific. Most vesicles are protein-coated. Vesicle Endocytosis Receptor-mediated endocytosis—clathrin-coated pits provide main route for endocytosis and transcytosis Uptake of enzymes low-density lipoproteins, iron, and insulin² (c) Receptor-mediated endocytosis Extracellular substances bind to specific receptor proteins in regions of coated pits, enabling the cell to ingest and concentrate specific substances (ligands) in protein-coated vesicles. Ligands may Vesicle simply be released inside the cell, or combined with a lysosome to digest contents. Receptors are recycled to Receptor recycled the plasma membrane in to plasma membrane vesicles. Exocytosis Examples: Hormone secretion Neurotransmitter release Mucus secretion Ejection of wastes² Plasma membrane The process Extracellular SNARE (t-SNARE) of exocytosis fluid Fusion pore formed Secretory 1 The membrane- Vesicle vesicle bound vesicle 3 The vesicle SNARE (v-SNARE) migrates to the and plasma Molecule to plasma membrane. membrane fuse be secreted and a pore Cytoplasm opens up. 2 There, proteins 4 Vesicle at the vesicle contents are Fused surface (v-SNAREs) v- and bind with t-SNAREs released to the t-SNAREs (plasma membrane cell exterior. proteins). Summary of Active Processes Process Energy Source Example Primary active transport ATP Pumping of ions across membranes Secondary active transport Ion gradient Movement of polar or charged solutes across membranes Exocytosis ATP Secretion of hormones and neurotransmitters Phagocytosis ATP White blood cell phagocytosis Pinocytosis ATP Absorption by intestinal cells Receptor-mediated ATP Hormone and cholesterol uptake ² endocytosis References 1. Meeking J. Cells the Living Unit. Part A [PowerPoint Slides]. 2010. 2. Meeking J. Cells the Living Unit. Part B [PowerPoint Slides]. 2010. 3. Azhar A. Bio Cells [PowerPoint Slides]. 2022 Aug 17. Available from:https://www.slideshare.net/ArishaAzhar/biocells1ppt 4. Sam Houston State University. Cell Structure & Function[PowerPoint Slides]. Available from: https://www.shsu.edu/academics/agricultural-sciences-and-engineering- technology/documents/Cell_structure_function.ppt 5. Pitts GR, Schiller JR, and Thompson J. Cells: The Living Units. [PowerPoint Slides]. (2010). Available from https://www.google.tt/?gws_rd=cr&ei=vcUxUtKcBIqK9gSx_YHICA#q=chapter+3+cells+the+living+units+ ppt 6. Boominathan. Human Physiology: Cell Structure and Function. [PowerPoint Slides]. 2012