Fundamentals of the Nervous System PDF
Document Details
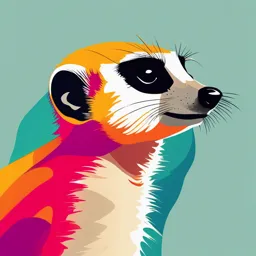
Uploaded by AccomplishedLongBeach
Victoria University
Tags
Summary
This document provides an introduction to the fundamentals of the nervous system, including learning objectives, organization, and divisions like the central and peripheral nervous systems. It covers topics such as neurons, neuroglia and synaptic transmission.
Full Transcript
Fundamentals of the Nervous System Learning Objectives Describe the general functions of the nervous system. Organization of the nervous system Compare and contrast the central nervous system (CNS) and the peripheral nervous system (PNS) with respect to structure and function. Differentiate be...
Fundamentals of the Nervous System Learning Objectives Describe the general functions of the nervous system. Organization of the nervous system Compare and contrast the central nervous system (CNS) and the peripheral nervous system (PNS) with respect to structure and function. Differentiate between the motor (efferent) and sensory (afferent) components of the nervous system. Compare and contrast the somatic motor and autonomic motor divisions of the nervous system. Diagram or describe the components of the nervous system that function as a control system, including sensory receptors, afferent (sensory) pathways, integration center (e.g., central nervous system [CNS]), efferent (motor) pathways, and targets (effectors). Diagram or describe the major structures of a typical neuron (e.g., cell body, dendrites, and axon) and indicate which regions receive input signals and which components transmit output signals. Compare and contrast the three functional types of neurons (i.e., sensory neurons, interneurons [association neurons], and motor neurons) with respect to their structure, location, and function. List and describe the six types of glial cells, their structure, major functions, and locations (i.e., central or peripheral nervous system). 2 An Introduction to the Nervous System The nervous system – Includes various organs Brain and spinal cord Receptors of sense organs (eyes, ears, etc.) Nerves that connect to other systems – Nervous tissue contains two kinds of cells Neurons for intercellular communication Neuroglia (glial cells) – Essential to survival and function of neurons – Preserve structure of nervous tissue 3 © 2018 Pearson Education, Ltd. Functions of Nervous System Nervous system has three overlapping functions 1. Sensory input Information gathered by sensory receptors about internal and external changes 2. Integration Processing and interpretation of sensory input 3. Motor output Activation of effector organs (muscles and glands) produces a response © 2016 Pearson Education, Ltd. Figure 11.1 The nervous system’s functions. Sensory input Integration Motor output © 2013 Pearson Education, Inc. Functions of Nervous System Nervous system is divided into two principal parts: Central nervous system (CNS) Brain and spinal cord of dorsal body cavity Integration and control center Interprets sensory input and dictates motor output Higher functions of brain include intelligence, memory, learning, and emotion Peripheral nervous system (PNS) Includes all nervous tissue outside CNS and ENS (enteric NS) Delivers sensory information to the CNS Carries motor commands to peripheral tissues Consists mainly of nerves that extend from brain and spinal cord Spinal nerves to and from spinal cord Cranial nerves to and from brain © 2016 Pearson Education, Ltd. Functions of Nervous System Peripheral nervous system (PNS) has two functional divisions – Sensory (afferent) division Somatic sensory fibers: convey impulses from receptors in skin, skeletal muscles, and joints to CNS Visceral sensory fibers: convey impulses from receptors in visceral organs to CNS – Motor (efferent) division Transmits impulses from CNS to effector organs – Muscles and glands are target organs that respond to motor commands Two divisions – Somatic nervous system – Autonomic nervous system © 2016 Pearson Education, Ltd. Divisions of the Nervous System Efferent division of PNS – Somatic nervous system (SNS) Controls skeletal muscle contractions Both voluntary and involuntary (reflexes) – Autonomic nervous system (ANS) Controls subconscious actions, contractions of smooth and cardiac muscle, and glandular secretions Sympathetic division has a stimulating effect Parasympathetic division has a relaxing effect 8 © 2018 Pearson Education, Ltd. Figure 11.2 Levels of organization in the nervous system. Central nervous system (CNS) Peripheral nervous system (PNS) Brain and spinal cord Cranial nerves and spinal nerves Integrative and control centers Communication lines between the CNS and the rest of the body Sensory (afferent) division Motor (efferent) division Somatic and visceral sensory Motor nerve fibers nerve fibers Conducts impulses from the CNS Conducts impulses from to effectors (muscles and glands) receptors to the CNS Somatic nervous Autonomic nervous Somatic sensory fiber Skin system system (ANS) Somatic motor Visceral motor (voluntary) (involuntary) Conducts impulses Conducts impulses from the CNS to from the CNS to skeletal muscles cardiac muscles, smooth muscles, Visceral sensory fiber and glands Stomach Skeletal muscle Motor fiber of somatic nervous system Sympathetic division Parasympathetic Mobilizes body systems division during activity Conserves energy Promotes house- keeping functions during rest Sympathetic motor fiber of ANS Heart Structure Function Sensory (afferent) division of PNS Parasympathetic motor fiber of ANS Bladder © 2013 Pearson Education, Motor (efferent) division of PNS Inc. Neuroglia Nervous tissue consists of two principal cell types: Neuroglia (glial cells): small cells that surround and wrap delicate neurons Support and protect neurons Make up half the volume of the nervous system Many types in CNS and PNS Neurons (nerve cells): excitable cells that transmit electrical signals Basic functional units of the nervous system Send and receive signals Function in communication, information processing, and control © 2016 Pearson Education, Ltd. Supporting Cells: Neuroglia The supporting cells (neuroglia or glial cells): Provide a supportive scaffolding for neurons Separate and insulate neurons Guide young neurons to the proper connections Promote neuronal health and growth Six types of glial cells (4 in the CNS and 2 in the PNS). Neuroglia in the CNS: - Astrocytes, - Microglia, - Ependymal cells, - Oligodendrocytes. Neuroglia in the PNS:- Satellite cells,- Schwann cells Cilia Figure 11.4 Neuroglia. Fluid-filled cavity Capillary Ependymal cells Brain or Neuron spinal cord tissue Ependymal cells line cerebrospinal fluid–filled cavities. Astrocyte Myelin sheath Process of Astrocytes are the most oligodendrocyte abundant CNS neuroglia. Nerve fibers Oligodendrocytes have processes that form myelin sheaths around CNS Neuron nerve fibers. Microglial Cell body of neuron cell Satellite cells Schwann cells (forming myelin sheath) Microglial cells are defensive cells in the CNS. Nerve fiber © 2016 Pearson Education, Inc. Satellite cells and Schwann cells (which form myelin) surround neurons in the PNS. Nervous Tissue: Neurons Neurons = nerve cells Cells specialized to transmit messages Major regions of neurons Cell body – nucleus and metabolic center of the cell Processes – fibers that extend from the cell body Extensions outside the cell body Dendrites – conduct impulses toward the cell body Axons – conduct impulses away from the cell body Copyright © 2003 Pearson Education, Inc. publishing as Benjamin Cummings Figure 12–2b The Anatomy of a Typical Neuron. Neuron Nissl bodies (RER Dendritic branches and free ribosomes) Mitochondrion Axon hillock Initial segment Axolemma Axon of axon Telodendria Direction of action potential Golgi apparatus Axon terminals Neurofilament Nucleolus Nucleus Dendrite See Figure 12–15 Presynaptic cell b Details of neuron structure and directional movement of an action potential. Postsynaptic cell 14 © 2018 Pearson Education, Ltd. Support, nutrient supplies, guidance, control the chemical Astrocytes environment, can release neurotransmitters CNS Neuroglial cells Microglia Phagocytise bacteria or neuronal debris Supporting cells Line cavities of the brain and spinal cord, provide a Ependymal cells permeable barrier between cerebro-spinal fluid and nervous tissue Oligodendrocytes Provide myelin sheaths (insulating covering) PNS Satellite cells Functions similar to astrocytes Neuroglial cells Supporting cells Schwann cells Provide myelin sheaths (insulating covering) Cell body Contains the nucleus; produce neurotransmitters (Nissl bodies); The plasma membrane can receive information Neurons from other neurons excitable cells Dendrites Receive information and convey towards the cell body Axons Generate and transmit action potentials; release neurotransmitters from the axonal terminals; provide movement of molecules to and from the cell body Neuron Processes (cont.) Myelin sheath Composed of myelin, a whitish, protein-lipid substance Function of myelin Protect and electrically insulate axon Increase speed of nerve impulse transmission Myelinated fibers: segmented sheath surrounds most long or large-diameter axons Nonmyelinated fibers: do not contain sheath Conduct impulses more slowly © 2016 Pearson Education, Ltd. Structural Classification of Neurons Structural classification Three types grouped by number of processes 1. Multipolar: three or more processes (1 axon, others dendrites) Most common and major neuron type in CNS 2. Bipolar: two processes (one axon, 1one dendrite) Rare (ex: retina and olfactory mucosa) 3. Unipolar: one T-like process (two axons) Also called pseudounipolar Peripheral (distal) process: associated with sensory receptor Proximal (central) process: enters CNS © 2016 Pearson Education, Ltd. Functional Classification of Neurons Functional: Sensory (afferent) — transmit impulses toward the CNS Motor (efferent) — carry impulses away from the CNS to an effector (muscle/gland) Interneurons (association neurons) — integrate information between sensory and motor neurons Learning Objectives Neurophysiology Define resting membrane potential (RMP) of a cell, explain how RMP is measured, and give a typical value for RMP. Describe the physiological basis of a cell’s resting membrane potential (RMP), including membrane permeability to ions, types of ion channels responsible for the RMP, and the electrochemical gradients for key ions. Given a graph of an action potential (membrane potential as a function of time), label or describe each phase, name the ions involved in each phase, and describe the direction of ion flow across the membrane. Define depolarization, hyperpolarization, repolarization Describe the role of the sodium-potassium pump (Na-K-ATPase) in maintenance of the resting membrane potential (RMP). 19 Membrane Potential All plasma (cell) membranes produce electrical signals by ion movements – Membrane potential is particularly important to neurons Resting membrane potential – The membrane potential of a resting cell Graded potential – Temporary, localized change in resting potential – Caused by a stimulus Action potential – Is an electrical impulse – Produced by graded potential – Propagates along surface of axon to synapse 20 © 2018 Pearson Education, Ltd. 12-4 Membrane Potential Resting membrane potential – Three important concepts The extracellular fluid (ECF) and intracellular fluid (cytosol) differ greatly in ionic composition – Extracellular fluid contains high concentrations of Na+ and Cl– – Cytosol contains high concentrations of K+ and negatively charged proteins Cells have selectively permeable membranes Membrane permeability varies by ion 21 © 2018 Pearson Education, Ltd. Generating the Resting Membrane Potential A voltmeter can measure potential (charge) difference across membrane of resting cell Resting membrane potential of a resting neuron is approximately – 70 mV The cytoplasmic side of membrane is negatively charged relative to the outside The actual voltage difference varies from –40 mV to –90 mV The membrane is said to be polarized © 2016 Pearson Education, Ltd. Generating the Resting Membrane Potential (cont.) Potential generated by: Differences in ionic composition of ICF and ECF Differences in plasma membrane permeability Voltmeter Plasma membrane Ground electrode outside cell Microelectrode inside cell Axon Neuron © 2016 Pearson Education, Ltd. Generating the Resting Membrane Potential (cont.) Differences in ionic composition- creates a chemical gradient ECF has higher concentration of Na+ than ICF Na+ ions move into the cell through sodium leak channels. ICF has higher concentration of K+ than ECF Balanced by negatively charged proteins so K+ ions tend to move out of the cell through potassium leak channels. Plasma membrane is highly permeable to K+ -creates electrical gradients Potassium ions leave the cytosol more rapidly than sodium ions enter. As a result, there are more positive charges outside the plasma membrane. Inside the cytosol is negative. Sodium–potassium exchange pump, powered by ATP: Ejects 3 Na+ for every 2 K+ brought in Stabilizes resting membrane potential (–70 mV) © 2016 Pearson Education, Ltd. Focus Figure 11.1-1 Generating a resting membrane potential depends on (1) differences in K+ and Na+ concentrations inside and outside cells, and (2) differences in permeability of the plasma membrane to these ions. The concentrations of Na+ and K+ on each side of the membrane are different. Outside cell The Na+ concentration is higher outside the Na+ cell. K+ Na+ Na+ Na+ (5 mM) (140 mM) The K+ concentration is higher inside the cell. K+ K+ K+ Na+ (140 mM) (15 mM) Na+-K+ pumps maintain Inside cell the concentration gradients of Na+ and K+ across the membrane. © 2016 Pearson Education, Ltd. Membrane Potential Na+ and K+ are the primary determinants of membrane potential – Na+ and K+ channels are either passive or active Passive ion channels (leak channels) – Are always open – Permeability changes with conditions Active ion channels (gated ion channels) – Open and close in response to stimuli – At resting membrane potential, most are closed Types of active channels – Chemically gated ion channels-Open when they bind specific chemicals (e.g., ACh) – Voltage-gated ion channels-Respond to changes in membrane potential – Mechanically gated ion channels-Respond to membrane distortion 26 © 2018 Pearson Education, Ltd. Action Potentials Principal way neurons send signals Means of long-distance neural communication Occur only in muscle cells and axons of neurons Brief reversal of membrane potential with a change in voltage of ~100 mV Action potentials (APs) do not decay over distance as graded potentials do In neurons, also referred to as a nerve impulse Involves opening of specific voltage-gated channels Activation gate opens when stimulated Inactivation gate closes to stop ion movement © 2016 Pearson Education, Ltd. Action Potential Generation of action potentials – Step 1: Resting State – Step 2: Activation of voltage-gated Na+ channels Na+ rushes into cytosol Inner membrane surface changes from negative to positive Results in rapid depolarization – Step 3: Inactivation of Na+ channels and activation of K+ channels At +30 mV, inactivation gates of voltage-gated Na+ channels close Voltage-gated K+ channels open K+ moves out of cytosol Repolarization begins – Step 4: Return to resting membrane potential Voltage-gated K+ channels begin to close – As membrane reaches normal resting potential – K+ continues to leave cell – Membrane is briefly hyperpolarized to –90 mV After all voltage-gated K+ channels finish closing – Resting membrane potential is restored – Action potential is over 28 © 2018 Pearson Education, Ltd. Focus Figure 11.2-1 The action potential (AP) is a brief change in membrane potential in a patch of membrane that is depolarized by local currents. The big picture What does this graph show? During the course of an action potential (below), voltage changes over time at a given point within the axon. 2 Depolarization is 3 Repolarization is caused by Na+ flowing caused by K+ flowing into the cell. out of the cell. Membrane potential (mV) +30 4 Hyperpolarization is caused by K+ continuing to 3 leave the cell. 0 2 −55 Threshold −70 1 1 1 Resting state. No ions 4 move through voltage-gated channels. 0 1 2 3 4 Time (ms) © 2016 Pearson Education, Inc. Action potential: Summary Threshold and the All-or-None Phenomenon Not all depolarization events produce APs For an axon to “fire,” depolarization must reach threshold voltage to trigger AP At threshold: Membrane is depolarized by 15 to 20 mV Na+ permeability increases Na+ influx exceeds K+ efflux The positive feedback cycle begins All-or-None: An AP either happens completely, or does not happen at all © 2016 Pearson Education, Ltd. Conduction Velocity APs occur only in axons, not other cell areas AP conduction velocities in axons vary widely Rate of AP propagation depends on two factors: 1. Axon diameter Larger-diameter fibers have less resistance to local current flow, so have faster impulse conduction 2. Degree of myelination Two types of conduction depending on presence or absence of myelin Continuous conduction Saltatory conduction © 2016 Pearson Education, Ltd. Membrane Is the difference in electrical charge between the inside and outside of a cell generated by different potential concentrations of Na+, K+, Cl−, and protein anions (A−) inside and outside of the cell. Concentrations of K+ and A- higher inside the cell and concentrations of Na+ and Cl− higher outside the cell. The resting membrane potential of the neurons is between -45 to -90 millivolts (in average about -70 mV). Na+ -K+ maintains the concentration gradient , it constantly expels three Na+ ions from the cell, and moves pump two K+ ions back into the cell. Ion The membrane pores made of protein permeable selectively to specific types of ions. Types: I. channels Passive, or leakage, channels – always open; II. Gated channels: a) Chemically gated channels – open with binding of a specific neurotransmitter; b) Voltage-gated channels – open and close in response to change in membrane potential; c) Mechanically gated channels – open and close in response to physical deformation of receptors. Phases of 1 – resting state: all Na+ and K+ gated channels are closed, membrane potential at resting level. the action 2 – depolarization: Na+ channels are open, Na+ flows into the cell; the inside of the membrane potential becomes less negative than the resting potential. 3 – repolarization: Na+ channels inactivating, K+ channels are open, K+ ions move out of the cell; the membrane returns to its resting membrane potential. 4 – hyperpolarization: some K+ channels remain open, some K+ ions move out of the cell; the inside of the membrane becomes more negative than the resting potential. Threshold Depolarization of membrane by 15 to 20 mV to induce the action potential firing. Rate of is determined by: 1) Axon diameter – the larger the diameter, the faster the impulse; 2) Presence of impulse a myelin sheath – myelination dramatically increases impulse speed. Saltatory conduction occurs in propagation myelinated axons; Action potentials are triggered only at the nodes of Ranvier (gaps between Schwann cells) and jump between the nodes. Learning Objectives Neurotransmitters, neuromodulators, and synaptic transmission Define synapse and describe the structure of a chemical synapse (e.g., cleft, pre- and postsynaptic cells). Compare and contrast electrical and chemical synapses Describe the events of synaptic transmission from the action potential arrival at the axon terminal of the presynaptic cell to the effects of neurotransmitter binding on the postsynaptic cell. Describe mechanisms for termination of synaptic neurotransmitter activity (e.g., reuptake, enzymatic breakdown, diffusion) and predict the consequences of a change in the process (e.g., the inhibition of acetylcholinesterase, AChE). Compare and contrast the effects of excitatory and inhibitory neurotransmitters on the postsynaptic cell. 34 Synapses Synapse – Specialized site where a neuron communicates with another cell – Presynaptic neuron Sends the message – Postsynaptic neuron Receives message – 2 Types of synapses- electrical vs chemical Electrical synapses – Direct physical contact between cells – Presynaptic and postsynaptic membranes are locked together at gap junctions – Ions pass between cells through pores – Local current affects both cells – Action potentials are propagated quickly – Found in some areas of brain, the eye, and ciliary ganglia 35 © 2018 Pearson Education, Ltd. Electrical Chemical synapse synapse http://www.ncbi.nlm.nih.gov/bookshelf/br.fcgi?book=neurosci&part=A 318 Chemical Synapses Most common type of synapse Specialized for release and reception of chemical neurotransmitters Typically composed of two parts Axon terminal of presynaptic neuron: contains synaptic vesicles filled with neurotransmitter Receptor region on postsynaptic neuron’s membrane: receives neurotransmitter -Usually on dendrite or cell body Two parts separated by fluid-filled synaptic cleft Electrical impulse changed to chemical across synapse, then back into electrical © 2016 Pearson Education, Ltd. Transmission at chemical synapses 38 Termination of Neurotransmitter Effects Neurotransmitter bound to a postsynaptic neuron: Produces a continuous postsynaptic effect Blocks reception of additional “messages” Must be removed from its receptor Removal of neurotransmitters occurs when they: Are degraded by enzymes; Are reabsorbed by astrocytes or the presynaptic terminals (reuptake); Diffuse away from the synaptic cleft. Synapse Functional junction between 2 neurons or between a neuron and an effector cell that mediates information transfer. Presynaptic neuron – conducts impulses toward the synapse; Postsynaptic neuron – transmits impulses away from the synapse. Electrical synapse The membranes of the two communicating neurons are linked together by a gap junction that contains precisely aligned, paired channels in the membrane of the pre- and postsynaptic neurons, such that each channel pair forms a pore. As a result, an ionic current and some substances (eg. ATP) diffuse through the gap junction pores. Transmission is fast. Chemical synapse Specialized for the release and reception of neurotransmitters. Typically composed of two parts: 1) Axonal terminal of the presynaptic neuron, which contains synaptic vesicles; 2) Receptor region on the postsynaptic neuron. Synaptic cleft Fluid-filled space separating the presynaptic and postsynaptic neurons Prevents nerve impulses from directly passing from one neuron to the next. Transmission across the synaptic cleft: Is a chemical event (as opposed to an electrical one); Ensures unidirectional communication between neurons. Neurotransmitters are endogenous chemicals which relay, amplify, and modulate signals between a neuron and another cell; they are made in the cell body and packaged into synaptic vesicles in the nerve terminals on the presynaptic side of a synapse. Transmission at 1) Nerve impulses reach the axonal terminal of the presynaptic neuron and open Ca2+ the chemical channels; 2) Neurotransmitter is released into the synaptic cleft via exocytosis; 3) synapse Neurotransmitter crosses the synaptic cleft, binds to receptors on the postsynaptic neuron; 4) Postsynaptic membrane permeability changes, causing an excitatory or inhibitory effect. Transmission is slow. Removal of occurs when they: are degraded by enzymes; are reabsorbed by astrocytes or the neurotransmitters presynaptic terminals (reuptake); diffuse away from the synaptic cleft. Neurotransmitters 50 or more neurotransmitters have been identified Classes of neurotransmitters Excitatory neurotransmitters Cause depolarization of postsynaptic membranes Promote action potentials Inhibitory neurotransmitters Cause hyperpolarization of postsynaptic membranes Suppress action potentials The effect of a neurotransmitter on postsynaptic membrane Depends on the properties of the receptor Not on the nature of the neurotransmitter © 2016 Pearson Education, Ltd. Functional Classification of Neurotransmitters Excitatory receptors depolarize membranes (e.g., glutamate) Inhibitory receptors hyperpolarize membranes (e.g., GABA and glycine) Some neurotransmitters have both excitatory and inhibitory effects Determined by the receptor type of the postsynaptic neuron Example: acetylcholine Excitatory at neuromuscular junctions with skeletal muscle Inhibitory in cardiac muscle Functional Classification of Neurotransmitters