British Airways Global Learning Academy – Basic Aerodynamics PDF
Document Details
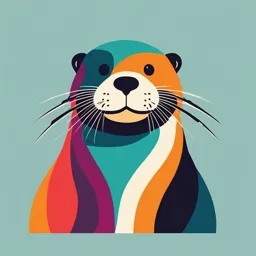
Uploaded by HardierMeadow
2023
null
null
Tags
Related
Summary
This document provides a detailed introduction to basic aerodynamics, exploring the four forces of flight (lift, weight, thrust, and drag) and their relationship. It explains how these forces interact and balance for steady flight, using diagrams and illustrations. It also touches on concepts of turning and maintaining flight stability.
Full Transcript
British Airways Global Learning Academy – Basic Aerodynamics 8.3 – Theory Of Flight Relationship Between Lift Weight, Thrust And Drag Four Forces Of Flight Lift This force is produced mainly by the aircraft’s wings. It acts at right angles to the line of flight and through the centre of pressure (C...
British Airways Global Learning Academy – Basic Aerodynamics 8.3 – Theory Of Flight Relationship Between Lift Weight, Thrust And Drag Four Forces Of Flight Lift This force is produced mainly by the aircraft’s wings. It acts at right angles to the line of flight and through the centre of pressure (CoP) of the wings. Weight The weight acts vertically downwards through the aircraft’s centre of gravity (CoG) no matter what attitude the aircraft assumes. Thrust Thrust is the propelling force delivered by the aircraft’s powerplant which are normally arranged symmetrically about the aircraft’s centre line. The thrust force may be taken to act parallel to the line of flight. Drag Figure 1 – The Four Forces In Flight Forces In Steady State Level Flight For simplicity, thrust and drag forces are considered as acting parallel to the longitudinal axis, and their displacement from this axis depends on the design of the aircraft, high wing or low wing, the position of the engine(s), and so on. Drag opposes the forward motion of the aircraft and can be regarded as a rearward acting force through the centre of pressure. Even when the conditions above have been satisfied the aircraft would still have a tendency to rotate nose up or down if the lines of action of the four forces were not arranged correctly. If an aircraft is flying at a constant height and speed, lift equals weight and thrust equals drag as shown in Figure 1 The arrangement depends on the design of the aircraft, but usually the CoP is designed to be aft of the CoG so that the lift and weight forces, acting together, tend to force the aircraft’s nose down. This tendency is counteracted by arranging the thrust line to be below the line of drag, thus providing a nose up couple as shown in Figure 1a. Under these conditions the lift has been adjusted by altering the angle of attack until it exactly equals the weight which it must support. Similarly, the thrust of the engine(s) has been set to exactly equal the aircraft drag generated at that particular speed. Module 08B ETBN 0492 October 2023 Edition 5 Basic Aerodynamics – Theory Of Flight British Airways Global Learning Academy – Basic Aerodynamics LIFT LIFT DRAG DRAG THRUST THRUST WEIGHT WEIGHT LIFT LIFT / WEIGHT COUPLE THRUST / DRAG COUPLE CoG Nose DOWN moment THRUST WEIGHT DRAG Nose UP moment Fig 1a – Lift/Weight And Thrust/Drag Turning Moments Module 08B ETBN 0492 October 2023 Edition 6 Basic Aerodynamics – Theory Of Flight British Airways Global Learning Academy – Basic Aerodynamics The particular arrangement shown in Figure 1a has the advantage that if the thrust force disappears, through engine failure, the lift weight couple will force the nose down and the aircraft will assume a gliding attitude. It will, therefore, maintain enough speed to prevent it stalling. The couple between the thrust and drag forces may compliment the lift/weight pitching moment (Figure 1b - top), or oppose it (Figure 1b bottom). With the couples arranged as shown at figure 1a – top, it would be necessary for the tailplane to produce negative lift, in order to maintain level flight. In order to maintain steady flight the forces acting on an aeroplane must be in balance, with no turning moment about any axis. In this condition the aircraft is said to be trimmed. The consequence of this downward force produced by the tailplane is effectively an increase in the aircraft weight. Consequently, for a given speed, a greater angle of attack must be used to produce the required lift, and this results in an increase in the amount of drag produced. This is one example of trim drag, we'll come across others later. The condition is achieved by balancing the lift, weight, thrust and drag forces acting at the aircraft's CoG and CoP so that: Lift equals weight, otherwise the aircraft would climb or descend. Thrust equals drag, otherwise the aircraft would accelerate or decelerate. A change in any one of the forces will disturb the trim of the aircraft, causing it to pitch either nose up or nose down. One important consideration in respect of the lift/weight force couple is the way in which the aircraft behaves in the event that the thrust/drag couple is destroyed, in other words during engine failure with a single engine aircraft. Providing that the centre of gravity and the centre of pressure are not coincident a force couple will be set up by the lift and the weight forces, and this will result in a pitching moment, as shown at figure 1. With the centre of gravity forward of the centre of pressure, the natural tendency will be for the nose to drop when the thrust/drag couple is removed as the engine fails, and this is desirable. The magnitude of the pitching moment will depend on the magnitude of lift and weight forces, but also on the distance between the centre of gravity and the centre of pressure. The position of the CoG will depend on the way in which the aircraft is loaded, and on the way in which fuel is transferred/consumed in flight. The position of the centre of pressure depends on the angle of attack, with the CoP moving slowly forward as the angle increases, and then rapidly backwards at the stalling angle. Module 08B ETBN 0492 October 2023 Edition 7 Basic Aerodynamics – Theory Of Flight British Airways Global Learning Academy – Basic Aerodynamics LIFT / WEIGHT COUPLE THRUST / DRAG COUPLE LIFT / WEIGHT COUPLE THRUST / DRAG COUPLE Fig 1b – Different Thrust/Drag Couples Module 08B ETBN 0492 October 2023 Edition 8 Basic Aerodynamics – Theory Of Flight British Airways Global Learning Academy – Basic Aerodynamics Tailplane One reason for fitting a tailplane is to counter any residual out-ofbalance pitching moments arising from inequalities of the two main couples as already stated. The tailplane, although small in area and in lifting capabilities when compared to the main aerofoils, is able to balance large residual pitching moments due to the fact that it is placed some distance behind the Centre Of Gravity (CoG), and can exert considerable leverage because of the moment produced. At high speed the angle of attack of the mainplane will be small, which, in turn, will cause the Centre Of Pressure (CoP) to move rearwards, tending to make the aircraft’s nose drop as seen in Figure 1c. At low speed we see the opposite effect of the nose rising due to the CoP moving forward of the CoG as seen in Figure 1d. Fig 1c – High Speed – Nose Drops To counteract this tendency, the tailplane must carry a download force to re-balance the aircraft at high speed and upload force for low speed. Where a tailplane is likely, as in the above case, to carry loads in either direction, it may well be of symmetrical camber. This will mean that when the angle of attack of the tailplane is 0°, the chord line of the section will also be the neutral lift line. Whilst a tailplane is designed to be set at a definite angle of attack for normal flight, the variables of speed, angle of attack and changing loads, will, at times, require a different tailplane angle of incidence and to provide for this, some tailplanes are adjustable in flight. Module 08B ETBN 0492 October 2023 Edition Fog 1d – Low Speed – Nose Raises 9 Basic Aerodynamics – Theory Of Flight British Airways Global Learning Academy – Basic Aerodynamics Forces In The Climb Strangely enough the lift required is now less than was needed for level flight, since the lift now supports only that component of the weight given by: During a climb the aircraft gains potential energy by virtue of increased altitude, which is achieved by either or both of two means: 𝑊 𝐶𝑜𝑠 𝛳 Where ϴ is the angle of climb. By increasing the thrust above that required for level flight at a given speed. By using the aircraft's kinetic energy (without increasing power, and accepting a gradual loss of airspeed). We obviously haven't been given something for nothing, since the thrust available now has to match not only the drag, but also the component of the weight given by It is normal to combine these two means so aircraft tend to climb at a reduced airspeed and with an increased power setting. 𝑊 𝑆𝑖𝑛 𝛳 During the climb the lift continues to act at right angles to the flight path and the weight vertically downwards, however the two are now no longer directly opposed as in figure 2. The weight must now be resolved into 2 components: One acting in at 90º to the flight path which is supported by lift. One acting parallel to the flight path but in the opposite direction to the direction of travel, and is supplemented by drag. Module 08B ETBN 0492 October 2023 Edition 10 Basic Aerodynamics – Theory Of Flight British Airways Global Learning Academy – Basic Aerodynamics LIFT THRUST DRAG Angle of Climb 𝜽 Vertical component of weight 𝑾𝑪𝒐𝒔𝜽 WEIGHT Horizontal component of weight 𝑾𝑺𝒊𝒏𝜽 VECTOR DIAGRAM True Airspeed Vector (V) Rate of Climb (ROC) Ground Speed (VG) Angle of Climb (𝜽) Figure 2 – Forces Components Acting In A Climb Module 08B ETBN 0492 October 2023 Edition 11 Basic Aerodynamics – Theory Of Flight British Airways Global Learning Academy – Basic Aerodynamics Glide ratio is the ratio of the forward distance travelled to the vertical distance an aircraft descends when operated without power. Forces In A Glide And Glide Ratio The forces acting on an aircraft in a glide descent – that is, when no thrust is present; are as shown at figure 3. In looking at gliding angle and range, we have not yet considered one of the primary factors that will affect the gliding range – the prevailing wind. Gliding with a tail wind increases glide range, whereas gliding into a headwind reduces glide range. From this diagram it can be seen that a part of the aircraft’s weight vector is used to overcome the drag of the aircraft, while the other half of the vector must be overcome by lift in order to keep the aircraft in the air. The rate of descent is not affected by the prevailing wind. In other words, whether gliding into a headwind or with a tailwind, the aircraft will still reach the ground in the same time. What will alter is the distance it covers in the glide as shown in Figure 3a. In other words the aircraft’s potential energy (altitude) is used in the glide descent. The best glide angle depends upon the lift/drag ratio of the aircraft. We know that in order for an aircraft to stay in the air with the least amount of effort the wings must be operating at the optimum Lift/Drag ratio. We already know that to keep the aircraft in the air the lift produced by the wings must be equal to the weight of the aircraft, thus: 𝐿𝑖𝑓𝑡 = 𝑊𝑒𝑖𝑔ℎ𝑡 𝑎𝑐𝑡𝑖𝑛𝑔 𝑎𝑡 90° 𝑡𝑜 𝑡ℎ𝑒 𝑑𝑖𝑟𝑒𝑐𝑡𝑖𝑜𝑛 𝑜𝑓 𝑡𝑟𝑎𝑣𝑒𝑙 𝐿𝑖𝑓𝑡 = 𝑊 Cos 𝛳 Similarly we know that the drag force produced by the aircraft is fighting against the component of weight that is acting parallel to the direction of travel, thus: Figure 3a – Effects of Wind On Gliding Range 𝐷𝑟𝑎𝑔 = 𝑊𝑒𝑖𝑔ℎ𝑡 𝑎𝑐𝑡𝑖𝑛𝑔 𝑝𝑎𝑟𝑎𝑙𝑙𝑒𝑑 𝑡𝑜 𝑑𝑖𝑟𝑒𝑐𝑡𝑖𝑜𝑛 𝑜𝑓 𝑡𝑟𝑎𝑣𝑒𝑙 𝐷𝑟𝑎𝑔 = 𝑊 Sin 𝛳 Module 08B ETBN 0492 October 2023 Edition 12 Basic Aerodynamics – Theory Of Flight British Airways Global Learning Academy – Basic Aerodynamics LIFT DRAG Vertical component of weight 𝑾 𝑪𝒐𝒔 𝜽 WEIGHT Angle of Glide (𝜽) Horizontal component of weight 𝑾 𝑺𝒊𝒏 𝜽 VECTOR DIAGRAM True Airspeed Vector (V) Rate of Descent (ROD) Angle of Glide (𝜽) Ground Speed (VG) Figure 3 - Forces In A Glide Module 08B ETBN 0492 October 2023 Edition 13 Basic Aerodynamics – Theory Of Flight British Airways Global Learning Academy – Basic Aerodynamics Theory Of The Turn A smooth turn entry can be made only by the coordinated use of ailerons and rudder. When you move the wheel to the right, apply a bit of right rudder pressure, just enough to prevent the nose moving to the left. Once the nose has started in the correct direction, the rudder is no longer needed and it can be centred. An aircraft is turned by banking it with the ailerons. When it is banked over, the lift force is tilted, since lift always acts perpendicular to the wings and the horizontal component of the lift vector, Figure 4, will move the aircraft in the direction it is tilted. The wind pushing on the tail surface will align the nose of the aircraft with its relative wind and the aircraft will turn in a smooth circular flight path. Figure 4a – Load Factor The vertical component of the lift vector must be equal to the weight of the aircraft and while the aircraft is turning, the centrifugal force adds an apparent weight, and the total lift must be equal to both the weight of the aircraft and the centrifugal force. In order to prevent the nose of the aircraft dropping in a turn, the pilot must pull the control wheel back to increase the angle of attack enough to produce the additional lift needed. To appreciate the amount of apparent weight the centrifugal force adds to the load on the wings in a turn, look at figure 4a, where we see the load factors that are developed in a coordinated turn. If a 6.000-pound aircraft is in a coordinated 60-degree banked turn, its load factor is 2.0, which means that the wings will have to support 12,000 pounds. Figure 4 – Forces In A Turn A turn is caused by tilting the lift produced by the wing. The vertical tail surfaces keep the aircraft turned into its relative wind. Module 08B ETBN 0492 October 2023 Edition 14 Basic Aerodynamics – Theory Of Flight British Airways Global Learning Academy – Basic Aerodynamics Forces In The Turn During a turn the weight still acts vertically downward, and the lift continues to act parallel to the aircraft’s normal axis. The amount of lift which was sufficient to maintain straight and level flight is therefore insufficient to maintain straight flight with the aircraft tuning, see Figure 5. The horizontal component of the total lift vector is used to provide the centripetal force which causes the aircraft to follow a curved path and stops it side slipping in the direction of the turn. The additional lift which is required is necessarily generated by increasing the angle of attack, and this will result in a decrease in airspeed (and subsequent decrease in lift) unless the power is increased during turns using significant bank angles. The increase in lift which is required in a turn may be considered to compensate for the apparent increase in aircraft weight during the turn since we know that in order to keep an aircraft from descending lift must equal weight. In a turn, it is the lift force acting at 90º to the wings that must increase in order to increase its vertical component that now has to overcome the weight. Module 08B ETBN 0492 October 2023 Edition 15 Basic Aerodynamics – Theory Of Flight British Airways Global Learning Academy – Basic Aerodynamics LIFT LIFT Additional lift required for turn Additional lift required for turn Lift required for level flight Bank Angle (𝜃) Lift required for level flight 30º Bank Angle WEIGHT Horizontal component of Lift 𝑾 𝑺𝒊𝒏 𝜽 WEIGHT 60º Bank Angle Vertical component of Lift (lift required for level flight) 𝑾 𝑪𝒐𝒔 𝜽 Figure 5 – Forces In A Turn Module 08B ETBN 0492 October 2023 Edition 16 Basic Aerodynamics – Theory Of Flight