Metabolism - Energy Homeostasis PDF
Document Details
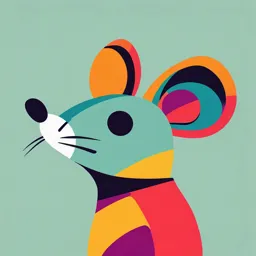
Uploaded by HandierMesa
CCNM - Boucher Campus
Tags
Related
Summary
This document discusses energy homeostasis and the role of the endocrine pancreas in regulating it. It covers the functions of insulin and glucagon, and details the process of glucose transport. The material is geared toward an undergraduate-level understanding of medical physiology.
Full Transcript
Energy Homeostasis – The Endocrine Pancreas Boron & Boulpaep’s Medical Physiology, 3rd ed. BMS 150 Week 12 Chapter 51 An Overview Energy homeostasis is a crucial process in maintaining stable energy levels within the body The endocrine p...
Energy Homeostasis – The Endocrine Pancreas Boron & Boulpaep’s Medical Physiology, 3rd ed. BMS 150 Week 12 Chapter 51 An Overview Energy homeostasis is a crucial process in maintaining stable energy levels within the body The endocrine pancreas plays a pivotal role in regulating it Consists mainly of clusters of cells called the islets of Langerhans, which contain several types of hormone-secreting cells including alpha cells that produce glucagon beta cells that produce insulin delta cells that produce somatostatin Insulin and glucagon are the primary hormones involved in regulating energy homeostasis When blood glucose levels rise, such as after a meal, beta cells in the pancreas release insulin into the bloodstream Insulin facilitates the uptake of glucose by cells, where it can be used for energy production or stored for future use This action helps to lower blood glucose levels back to normal. An Overview Conversely, when blood glucose levels drop, alpha cells in the pancreas release glucagon Glucagon signals the liver to break down stored glycogen into glucose and release it into the bloodstream Raising blood glucose levels to maintain energy balance. The balance between insulin and glucagon secretion is tightly regulated Dysregulation of this balance can lead to metabolic disorders like diabetes mellitus, characterized by abnormal blood glucose levels Other hormones produced by the endocrine pancreas, such as somatostatin, play modulatory roles in energy metabolism by regulating the secretion of insulin and glucagon Pancreatic Histology Junquiera’s Basic Histology – Chapter 20, Fig. 2017 Pancreatic islets About 1 million islets, mostly in the tail, comprising ~ 1% of total pancreatic mass ▪ 100 – 200 um in diameter, ▪ Rich capillary supply ▪ blood flows from the centre of the islet to the periphery Alpha cells – glucagon Beta cells – insulin Delta cells – somatostatin PP cell (sometimes called F cell) – pancreatic polypeptide ▪ Not discussed here Pancreatic Islets – Brief Summary Cell Type % of islet Hormone General Function Alpha 20% Glucagon Acts on several tissue to make energy stored in glycogen and fat available through glycogenolysis and lipolysis – INCREASES blood glucose Beta 70% Insulin Acts on several tissues to cause entry of glucose into cells – DECREASES blood glucose Delta 5 – 10% Somatostatin Inhibits release of other islet cell hormones Overview of Glucose Homeostasis Glucose homeostasis is part of a classic negative feedback loop ▪ If glucose drops under 3 mmol/L (50 mg/dL) then insulin secretion rapidly drops, and epinephrine and glucagon secretion markedly increases Multiple hormones increase blood glucose – only one drops it (insulin) ▪ Consequences of hypoglycemia much more immediately harmful than hyperglycemia Insulin Synthesis Insulin creation is a complex process that occurs in the beta cells of the pancreas within structures called the islets of Langerhans Transcription The process begins with the transcription of the insulin gene (INS) into messenger RNA (mRNA) within the nucleus of the beta cell mRNA processing The mRNA undergoes several processing steps, including capping, splicing, and polyadenylation, to produce a mature mRNA molecule Translation The mature mRNA exits the nucleus and enters the cytoplasm, where it binds to ribosomes Ribosomes read the mRNA sequence and assemble amino acids into a polypeptide chain according to the genetic code. Proinsulin synthesis The polypeptide chain synthesized by ribosomes is the precursor to insulin, known as proinsulin Proinsulin contains three domains: the A chain, the B chain, and the connecting C-peptide. As the polypeptide chain is elongated, it folds into its three-dimensional structure. Insulin Synthesis Proinsulin processing Within specialized cellular compartments called the endoplasmic reticulum (ER) and the Golgi apparatus, proinsulin undergoes post-translational modifications These modifications include cleavage of the C-peptide, which separates proinsulin into its mature insulin and C-peptide components Insulin packaging Once processed, mature insulin molecules are packaged into secretory vesicles, which are small membrane-bound compartments within the beta cell These vesicles contain enzymes and other proteins necessary for the regulated secretion of insulin. Insulin secretion When blood glucose levels rise, signalling pathways within the beta cell trigger the fusion of insulin-containing vesicles with the cell membrane, releasing insulin into the bloodstream Insulin then circulates throughout the body, where it binds to insulin receptors on target cells, such as muscle, fat, and liver cells, initiating various metabolic processes. Insulin Protein hormone ▪ α and β chains connected by disulfide bonds Circulates unbound Degraded by insulinase in liver, kidney, other tissues C-peptide – not active, but released alongside insulin ▪ Can measure C-peptide to determine how much endogenous insulin is secreted by diabetics ▪ Useful in those who are taking exogenous insulin as a medication Insulin secretion: blood glucose concentrations change the intracellular ratio of ADP:ATP this changes the membrane potential by changing the potassium conductance – Low glucose ! Increased ADP:ATP ratio ! open K+ channels ! hyperpolarization depolarization ! VGC opening ! exocytosis of insulin The Insulin Receptor Insulin binds to its receptor ! dimerization ! activating protein tyrosine kinase activity (autophosphorylation) There follows a cascade of phosphorylation events starting with tyrosine phosphorylation of IRS-1 and IRS-2 Insulin Receptor Activation – more detail Key Pathways: PI3K pathway ! Akt and mTOR activation IRS activates Ras ! Raf activation ! MEK & MAPK activation ! protein synthesis More on pathways Insulin Receptor Desensitization - Basics Cells that are chronically exposed to high levels of insulin: – reduce the number of insulin receptors expressed less synthesis and increased endocytosis of receptor – Down-regulate some signaling pathways distal to receptor activation – PI3K, IR phosphorylation – Likely most important mechanism of resistance Regulation of Insulin Secretion Stimulators Nutrients in the bloodstream ▪ Glucose (major) ▪ Amino acids (arg, lys) ▪ Free fatty acids Hormonal signals ▪ CCK, GIP (gastric inhibitory polypeptide), and GLP-1 (glucagon-like peptide) are released from the small intestine in response to food – these are known as incretins ▪ parasympathetic innervation – mostly from the cephalic phase of digestion ▪ GH – needed for normal insulin secretion Regulators of Insulin Secretion Inhibitors Hormones ▪ Somatostatin ▪ Epinephrine ▪ Leptin Sympathetic nervous system ▪ Both epinephrine and norepinephrine can inhibit insulin secretion – mediated by alpha receptors ▪ Stimulation of beta-receptors can stimulate some insulin secretion – however, activation of the SNS results in net inhibition of insulin secretion Importance of Incretins Incretin release from the intestines greatly increases insulin release ▪ IV glucose will not cause incretin release – see the much smaller insulin response to glucose administered IV in the top picture Bottom picture shows insulin and glucose levels in someone with Type 1 diabetes ▪ Very little insulin can be released ▪ Prolonged hyperglycemia Typical Values – Fasting vs. Fed Glucose Transport - An Overview Glucose transport refers to the movement of glucose molecules across cell membranes, allowing glucose to enter or exit cells as needed for energy production and metabolic processes Glucose transport is essential for maintaining proper blood glucose levels and providing energy to cells throughout the body Glucose Concentration Gradient Glucose transport occurs down its concentration gradient, meaning glucose moves from areas of higher concentration to areas of lower concentration. In the context of blood glucose regulation, glucose moves from the bloodstream (where it's often at higher concentrations after a meal) into cells (where it's utilized for energy or stored). Transport Proteins Glucose molecules cannot diffuse freely through the cell membrane due to their hydrophilic nature and the lipid- based structure of cell membranes. Instead, they rely on specialized transport proteins embedded within the cell membrane to facilitate their movement. Glucose Transporter Proteins (GLUTs) The primary facilitators of glucose transport are a family of proteins called glucose transporter proteins, or GLUTs. There are several types of GLUTs, each with different tissue distributions and kinetic properties. GLUT1: Found in various tissues, including the brain, red blood cells, and the placenta. It ensures basal glucose uptake. GLUT2: Found in the liver, pancreas, and kidneys. It participates in glucose sensing and hepatic glucose uptake. GLUT3: Predominantly found in neurons and plays a crucial role in glucose uptake in the brain. GLUT4: Predominantly found in skeletal muscle and adipose tissue. It is insulin-sensitive and plays a major role in postprandial glucose uptake. Glucose Transport - An Overview Regulation of GLUTs: Regulated by various factors, including insulin, intracellular glucose levels, and cellular energy status. For example, insulin promotes the translocation of GLUT4 transporters from intracellular vesicles to the cell membrane, increasing glucose uptake into insulin- sensitive tissues like skeletal muscle and adipose tissue Transport Mechanism Glucose transport via GLUTs typically involves facilitated diffusion, where glucose binds to the transporter on one side of the membrane, inducing a conformational change that allows it to be released on the other side Tissue-Specific Functions Different GLUT isoforms are expressed in various tissues, reflecting their distinct roles in glucose homeostasis For example, GLUT2 in pancreatic beta cells is crucial for glucose sensing and insulin secretion, while GLUT4 in skeletal muscle plays a significant role in glucose uptake during exercise. Glucose Transport Insulin-independent glucose transporters include: – SGLT-1 and SGLT-2: intestine and kidney – GLUT-1 and GLUT-3: wide distribution – GLUT-2: liver and pancreas Major insulin-dependent glucose transporter is GLUT-4 – Mostly in skeletal and cardiac muscle and adipose tissue – Skeletal muscle accounts for roughly 80% of insulin-mediated glucose uptake – Insulin mediates movement of intracellular transporters to membrane and synthesis of new transporters Insulin: Liver effects 1. Increased glucose uptake from bloodstream ▪ Glucokinase activation ! conversion of glucose into G-6-P (increased concentration gradient) 2. Increased glucose use ▪ Storage: increased glycogenesis, decreased glycogenolysis (increased glucokinase and glycogen synthase activity, decreased G-6-Pase activity) ▪ Energy: increased glycolysis (increased glucokinase, PFK, and pyruvate kinase activity) 3. Increased fatty acid synthesis and VLDL formation ▪ (increased fatty acid synthase and acetyl CoA carboxylase activity) ▪ Increased apoprotein and lipid synthesis to make VLDL Items in (brackets) – not tested for this class Insulin: Liver effects 4. Decreased ketogenesis ▪ Gluconeogenesis is inhibited (inhibited PEPCK, FBPase, G6Pase) ▪ Beta oxidation is inhibited (increased malonyl CoA) ▪ Therefore ketogenesis is decreased 5. Increased protein synthesis and decreased urea cycle activity ▪ Don’t need the urea cycle if limited protein catabolism Items in (brackets) – not tested for this class Insulin and the Liver See notes below for description of this FYI diagram Insulin: Muscle effects Increased glucose uptake - by increasing GLUT-4 availability Increased glucose use ▪ Storage: increased glycogenesis, decreased glycogenolysis (increased activity of hexokinase and glycogen synthase) ▪ Energy: increased glycolysis, energy usage (increased activity of hexokinase, PFK, pyruvate dehydrogenase) Increased amino acid uptake, increased protein synthesis, decreased proteolysis Increase in Na-K ATPase activity Items in (brackets) – not tested for this class Insulin and Skeletal Muscle See notes below for description of this FYI diagram Insulin: Adipose Effects Increased glucose uptake - by increasing GLUT-4 availability Increased glucose use ▪ Energy: Increased glycolysis Also increases glycerol-3-phosphate that can shunt into TG production Increased TG production - esterification of fats Decreased lipolysis – inhibits hormone-sensitive lipase Increases the removal of lipids from VLDL and chylomicrons ! into the adipocyte Insulin and Fat Cells See notes below for description of this FYI diagram Glucagon Synthesis Proglucagon and its metabolites The proglucagon molecule includes amino acid sequences that, depending on how the peptide chain is cleaved, can yield glucagon-related polypeptide (GRPP), glucagon, IP-1, GLP-1, IP-2, and GLP-2 Proteases in the pancreatic α cells cleave proglucagon at points that yield GRPP, glucagon, and a C-terminal fragment – glucagon is the most physiologically-relevant Proteases in neuroendocrine cells in the intestine cleave proglucagon to yield GLP-1 and GLP-2 ! important incretins Glucagon Secretion Stimulators ▪ Drop in blood glucose ▪ Rise in serum amino acids (arg, ala) ▪ Cortisol ▪ sympathetic nervous system stimulation ▪ Exercise, stress Due to cortisol, SNS Inhibitors ▪ Rise in blood glucose ▪ Somatostatin Glucagon: Liver effects 1. Increased glucose “output” to other tissues ▪ Increased glycogenolysis and decreased glycogenesis (reduced glucokinase and glycogen synthase activity, activates glycogen phosphorylase) ▪ Increased gluconeogenesis Decreased glycolysis (reduced glucokinase, PFK, pyruvate kinase) (increased PEPCK, fructose 1-6 bisphosphatase, G6Pase) ▪ Increased uptake of gluconeogenic precursors Ala, glu, pyruvate, lactate Items in (brackets) – not tested for this class Glucagon: Liver effects 2. Increased beta oxidation (decreased malonyl CoA) 3. Increased ketogenesis ▪ As beta oxidation increases, increased acetyl CoA and use of NAD ▪ Oxaloacetate is used for GNG, instead of being used in the citric acid cycle - therefore less CAC activity 4. Increased urea cycle activity Items in (brackets) – not tested for this class Glucagon and the Liver See notes below for description of this FYI diagram Glucagon: Adipose and Muscle Effects Adipose: stimulation of hormone-sensitive lipase (HSL) ▪ Promotes lipolysis and release of free fatty acids Muscle: no direct effects ▪ Glucose uptake and use decreased by increase in serum levels of free fatty acids from adipose Insulin/Glucagon Ratio High ratio ▪ Anabolic state Nutrient incorporation into peripheral tissues Low ratio ▪ Catabolic state Nutrient mobilization Insulin and glucagon inversely regulated ▪ Exception: low carb, high protein diet Insulin fluctuates much more than glucagon – the relative balance of the two is what changes in the fasting vs. fed state Somatostatin Polypeptide hormone produced in pancreatic delta cells and GI tract Predominantly acts in paracrine fashion in the pancreas ▪ tends to decrease the secretion of both insulin and glucagon Stimuli for release in pancreas and GIT include: ▪ Stimulators of insulin release Increase in serum glucose Increase in serum amino acids Increase in serum fatty acids ▪ Gastrointestinal hormones Secretin CCK Type I Diabetes Mellitus Robbins and Cotran’s Pathologic Basis of Disease Chapter 25 BMS 150 Week 12 Diabetes - Intro 1 million islets of Langerhans – mainly interested in the beta cells ▪ key players are the targets of insulin action, and their sensitivity to the hormone Multiple disorders, with the common theme of hyperglycemia ▪ diabetes = “urinates excessively”, mellitus = “sweet” Diabetes – laboratory definition Diabetes = hyperglycemia ▪ Results from defects in insulin secretion, insulin action, or (usually) both Diagnosis (basic): 1.random blood glucose (RBG) concentration > 11.1 mmol, with classical signs and symptoms (only has to be observed once) 2.FBG concentration > 7.0 mmol on more than one occasion 3.Abnormal oral glucose tolerance test (OGTT): > 11.1 mmol 1 hour after ingestion on more than one occasion 4.Hemoglobin A1c > 6.5% Fasting glucose should be < 5.6 mmol – it’s tightly regulated Diabetes - classification Type 1 diabetes - ~ 5 – 10% ▪ autoimmune disease ! pancreatic beta cell destruction, usually onset is in childhood but sometimes in early adulthood ▪ Discussed next class Type 2 diabetes - ~ 90 – 95% ▪ combination of peripheral resistance to insulin action and an inadequate secretory response by beta cells Monogenic and secondary causes – quite uncommon ▪ single-gene disorders or secondary to infection or pancreatic destruction by other means (tumours, inflammation, ischemia) FYI – Diabetes is not one disease Insulin Actions (Review) Regulation of Blood Glucose Levels When blood glucose levels rise, such as after a meal, insulin is released into the bloodstream. Insulin promotes the uptake of glucose by various tissues, including skeletal muscle, liver, and adipose tissue, where it can be used for energy or stored for future use. Promotion of Glycogen Synthesis In the liver and muscles, insulin stimulates the conversion of excess glucose into glycogen, a storage form of glucose This helps to regulate blood glucose levels by storing glucose when levels are high and releasing it when levels are low, maintaining a steady supply of glucose for energy. Inhibition of Gluconeogenesis Insulin inhibits the process of gluconeogenesis, which is the production of glucose from non-carbohydrate sources, such as amino acids and glycerol By suppressing gluconeogenesis, insulin helps to prevent excess glucose production and further contributes to the regulation of blood glucose levels. Cellular Growth and Differentiation Insulin plays a role in cellular growth, proliferation, and differentiation in various tissues, including the liver, muscles, and adipose tissue It helps to regulate cell growth and tissue remodelling processes. Insulin Actions Stimulation of Protein Synthesis Protein synthesis and inhibiting protein breakdown It enhances the uptake of amino acids by cells and stimulates protein synthesis in tissues such as skeletal muscle Regulation of Lipid Metabolism Promotes the storage of fatty acids in adipose tissue by stimulating lipogenesis, the synthesis of fatty acids and triglycerides It also inhibits lipolysis, the breakdown of triglycerides into free fatty acids and glycerol By promoting lipid storage and inhibiting lipid breakdown, insulin helps to regulate lipid levels in the bloodstream. Modulation of Appetite and Satiety Insulin can also act in the brain to regulate appetite and satiety It interacts with neural circuits involved in hunger and feeding behaviour, helping to signal when the body has consumed enough food and promoting feelings of satiety. Insulin physiology - review Diabetes – pancreatic pathology Type I: Reduction in the number and size of islets and leukocytic infiltrates in the islets (T- cells, mostly) ▪ inflammation and reduction in the size of islets ▪ More later – the immune system kills the pancreatic beta cells Type II: Reduction in islet cell mass as well as amyloid deposition around beta cells ▪ no inflammatory infiltrate ▪ insulin resistance is the primary trigger Type 1 Diabetes: Pathogenesis Healthy Type 1 Diabetes Type 2 Diabetes: Pathogenesis Healthy Type 2 Diabetes Insulin Resistance Type 1 Diabetes – Catch-Up Diabetes Mellitus Type 1 is a chronic metabolic disorder characterized by the pancreas not producing enough insulin. In this condition, glucose needs insulin to help it enter the cells to be used for energy. Since cells cannot use glucose, the blood glucose levels become extremely high. Typically occurs in adolescents and young adults. Type 1 Diabetes ▪ Usually diagnosed in those < 20 years can develop at any age ▪ Autoimmune destruction of beta cells Absolute deficit in insulin, insulin resistance is not found ▪ Twin concordance rate ~ 30% to 50% Genes implicated – major histocompatibility genes, other genes that are important in teaching the immune system to “tolerate” self-tissues Pathogenesis – T1DM Genetic etiologic factors: ▪ HLA DR3 or DR4 – the histocompatibility genes Account for 50% of genetic risk ▪ People with one copy of both DR3 and DR4 show greatly increased risk DQ8 also presents increased risk Polymorphisms are within antigen-presenting portion ▪ CTLA-4 and PTPN-22 – the “tolerance” genes CTLA-4 is a major “downregulator” of antigen presentation by APCs, interacts with CD-28 (Treg) PTPN-22 is important in the development of central Tregs in the thymus Pathogenesis – T1DM Environmental theories - Viral infections: Associations with mumps, rubella, coxsackie B, or cytomegalovirus ▪ Three different mechanisms proposed: “Bystander” damage - viral infections induce islet injury and inflammation, leading to release of β-cell antigens and activation of autoreactive T cells Viruses produce proteins that mimic β-cell antigens, and immune response to viral protein cross-reacts with self-tissue Viral infections early in life persist in pancreas, and re-infection with a related virus (“precipitating virus”) that shares antigenic characteristics leads to immune response against infected islet cells ▪ All these mechanisms need further confirmation before they can be definitively identified as causing the disease Pathogenesis – T1DM Gradual loss of β-cell mass likely due to destruction of β -cells by cytotoxic T-cells ▪ Disease manifestations appear after 90% of β cells destroyed ▪ T-cells seem to be the main problem: Self-reactive T-cells not destroyed in thymus Defects in regulatory T-cell function (the tolerance cells) Antigens likely attacked: insulin, glutamic acid decarboxylase, others (islet cell autoantigen 512) Autoantibodies may cause damage or just be a sign of immune dysregulation Pathogenesis – T1DM Viruses? Development of T1 DM Honeymooning: remaining β- cells become hyper-productive and compensate for failing insulin response Disease manifestations appear after 90% of β cells destroyed 18 Honeymooning and Islet cell loss As you kill β cells - remaining cells become hyperproductive ▪ When glucose goes up, remaining cells make insulin and glucose goes down again ▪ Some more die off and remaining ones become even more hyperactive Honeymooning is ability of remaining β-cells to become hyper-productive and compensate for failing insulin response ▪ once the “honeymooning” cells fail, deterioration is rapid Type I DM – Clinical Features Progresses quickly once significant loss of beta-cell mass occurs Typical initial presentation – acute, known as diabetic ketoacidosis: ▪ Subacute history of polyphagia, polydipsia, polyuria Why do these occur? ▪ Patients are often emaciated and quite dehydrated ▪ As patients lose the ability to use serum glucose to generate ATP and adipose tissue releases increasing amounts of fatty acids, liver generates ketones ketones are acidic, resulting in a drop in blood pH as blood pH drops, steady deterioration in level of consciousness ▪ For unclear reasons, patients who experience DKA often experience fairly severe generalized abdominal pain Figure 24-33 Sequence of metabolic derangements underlying the clinical manifestations of diabetes. An absolute insulin deficiency leads to a catabolic state, culminating in ketoacidosis and severe volume depletion. These cause sufficient central nervous system compromise to lead to coma and eventual death if left untreated. Volume depletion in DKA Elevated blood glucose ! an osmotic diuresis in the kidney ▪ Excess sugar in the urine ! drawing water from the blood into the urine ! loss of high volumes of water and dehydration ▪ The increased osmolarity of the blood contributes to the increased sensation of thirst As more and more fluid is lost and the sodium- potassium pump activity is reduced, K+ loss occurs ▪ Insulin stimulates activity of Na+/K+ ATPase ▪ More K+ outside the cells ! more K+ lost in the urine Complications of long- term hyperglycemia Remember: DM is associated with - Small vessels disease - Large vessels disease Hyperglycemia – who cares? Persistent hyperglycemia leads to: ▪ Advanced glycation end products (AGEs) – metabolic products of glucose non-enzymatically linked to amino groups of intra-and extracellular proteins ▪ AGE binds to R-AGE on macrophages, T-cells, smooth muscle cells and endothelial cells Hyperglycemia – who cares? Advanced glycation end products cont… ▪ Leads to: Release of pro-inflammatory cytokines and growth factors from intimal macrophages Generation of ROS in endothelial cells Increased procoagulant activity on endothelial cells Enhanced proliferation of vascular smooth muscle cells and synthesis of extracellular matrix ▪ AGEs cause cross-linking of matrix proteins and render them resistant to proteolysis Hyperglycemia – who cares? In the end, AGEs seem to contribute to: ▪ Decreased large artery elasticity ▪ Narrowing of smaller arteries ▪ Deposition of atherosclerotic plaques (trapping of LDL in AGE-linked matrix, smooth muscle proliferation) ▪ Increased susceptibility to coagulation Activation of protein kinase C pathway by hyperglycemia contributes to deposition of excess matrix, secretion of pro-inflammatory cytokines, and increased susceptibility to coagulation Diabetes – small vessel disease Hyaline arteriolosclerosis ▪ Vascular lesion associated with HTN more prevalent and more severe in diabetics than in nondiabetics ▪ Amorphous, hyaline thickening of wall of arterioles - causes narrowing of lumen Diabetic Microangiopathy ▪ Diffuse thickening of basement membranes ▪ Prominent in capillaries of skin, skeletal muscle, retina, renal glomeruli, and renal medulla ▪ Diabetic capillaries more leaky than normal to plasma proteins - underlies development of diabetic nephropathy, retinopathy, and some forms of neuropathy Diabetes - Neuropathy Loss of small and large axons, myelinated and unmyelinated ▪ Loss of pain sensation quite early - somewhat unique among peripheral neuropathies ▪ Can also lose vibration, proprioception, fine touch, much like many of the other peripheral neuropathies ▪ Autonomic neuropathy can feature postural hypotension, incomplete emptying of bladder resulting in recurrent infections, and sexual dysfuction