Heat Transfer PDF
Document Details
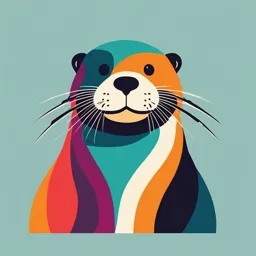
Uploaded by DynamicChrysoberyl6678
Tags
Summary
This document provides a comprehensive overview of heat transfer, covering concepts, modes (conduction, convection, radiation), and relevant applications like heat exchangers.
Full Transcript
HEAT TRANSFER DESCRIBE THE CONCEPT OF HEAT TRANSFER HEAT TRANSFER describes the flow of heat (thermal energy) due to temperature differences and the subsequent temperature distribution and changes. HEAT FLOW PRESSURE VELOCITY DRIVING FORCE OF HEAT TRA...
HEAT TRANSFER DESCRIBE THE CONCEPT OF HEAT TRANSFER HEAT TRANSFER describes the flow of heat (thermal energy) due to temperature differences and the subsequent temperature distribution and changes. HEAT FLOW PRESSURE VELOCITY DRIVING FORCE OF HEAT TRANSFER: DIFFERENCE IN TEMPERATURE DIFFERENT MODE OF HEAT TRANSFER CONDUCTION CONDUCTION HEAT ENERGY IS TRANSFERRED BY THE COLLISION OF MOLECULES OR OTHER PARTICLES WHEN TWO THINGS TOUCH, THE FASTER MOVING MOLECULES OF THE WARMER OBJECT CRASH INTO THE SLOWER MOVING MOLECULES OF THE COLDER OBJECT, TRANSFERRING ENERGY HEATS THEM UP. FOURIER’S LAW CONDUCTION HAPPEN WHEN THE MOLECULES OF MATTER VIBRATE. THE LAW STATE’S THAT THE CONDUCTIVE HEAT FLOW IS A PRODUCT OF THE THERMAL CONDUCTIVITY OF THE MATERIAL, THE AREA NORMAL TO THE HEAT FLOW AND THE TEMPERATURE GRADIENT ACROSS THE AREA. FOURIER’S LAW Q=- k A ∆T x FOURIER’S LAW R= x kA ∆T R= 𝑸ሶ CONVECTION CONVECTION TRANSFER OF ENERGY BY THE PHYSICAL MOVEMENT OF FLUID WHICH CAN BE LIQUID OR GAS CONVECTION N F A O T R U C R E A D L FACTOR AFFECTING HEAT TRANSFER THROUGH CONVECTION BOUNDARY LAYER Convective Heat Transfer Q =h A ∆T Convective Heat Transfer k h= x RADIATION RADIATION TRANSFER OF ENERGY IN THE FORM OF ELECTROMAGNETIC WAVES. REFLECTIVE COATING HELPS REDUCE THE HEAT TRANSFER FROM RADIATION A LITTLE, SINCE THIS ABSORBS FEWER ELECTROMANETIC WAVES. Radiation Heat Transfer Q =e σ A ∆T 4 HOW DO WE ACHIEVE THE DESIRED TEMPERATURE? HEAT EXCHANGERS EXAMPLES OF HEAT EXCHANGER CONCENTRIC TUBE CONCENTRIC TUBE TWO FLUIDS ARE SEALED OFF FROM EACH OTHER AND NEVER MIXED. THERE IS A TUBE INSIDE A TUBE. 1 2 CONCENTRIC TUBE FLUID FLOW PARALLEL FLOW COUNTER FLOW MAIN EQUATION OF HEAT TRANSFER Q =m Cp ∆T 1 2 HEAT TRANSFER WITH OVERALL HEAT COEFFICIENT Q =U A ∆T HEAT TRANSFER WITH OVERALL HEAT COEFFICIENT U= 1 R IF THE TEMPERATURE DIFFERENCE BETWEEN THE FLUIDS IS THE DRIVING FORCE, THEN THE HEAT EXCHANGER WILL NEED TO HAVE AN APPRORIATE AREA AND U VALUE TO ACHIEVE A REASONABLE AMOUNT OF HEAT TRANSFER. FINNED TUBES FINNED TUBES FINS ARE ADDED TO THE TUBE TO INCREASE ITS SURFACE AREA,WHICH ENHANCE ITS RATE OF HEAT TRANSFER AT THE SAME TIME. AXIAL FIN FINS RUN ALONG THE TUBES FLUID FLOWS MORE SLOWER OR VISCOUS RADIAL FINS DISCS ARE ADDED TO THE TUBE AND SPACED OUT FROM EACH OTHER, USUALLY IN REGULAR INTERVALS. FLUID FLOWS FASTER LIKE AIR TO FLOW AROUND THE TUBES PLATE HEAT EXCHANGER USES METAL PLATES TO TRANSFER HEAT BETWEEN FLUIDS. SURFACE AREA COLD WARM SHELL AND TUBE DESIGN SHELL AND TUBE MADE OF A LARGER SHELL WITH A BUNDLE OF SMALLER TUBES INSIDE SHELL BAFFLES TUBES SEATWORK IDENTIFY THE HEAT EXCHANGER CLASSIFICATION OF THE FOLLOWING: Steam Condenser Steam Boiler Cooler Heater ACTIVITY 1 IDENTIFY EXISTING MATERIALS THAT GOVERNS THE DIFFERENT MODE OF HEAT TRANSFER. MODE OF HEAT TRANSFER SURROUNDING TEMPERATURE INSIDE TEMPERATURE COMPOSITION OF MATERIALS TO BE SUBMITTED NEXT MEETING RECAP COMBINED MODES OF HEAT TRANSFER (Convection and Conduction) Fluid Flow Fluid Flow Th TL qCh qCl qR COMBINED MODES OF HEAT TRANSFER (Convection and Conduction) Fluid Flow Fluid Flow TH TL qCh qCl Twh qR Twl CONDUCTION THROUGH COMPOSITE WALL SAMPLE PROBLEM: One side of refrigeration cold chamber is 6m long by 3.7m high and consists of 168 mm thickness of cork between outer and inner walls of wood. The outer wood wall is 30mm thick and its outside face temperature is 20ºC, the inner wood wall is 35mm thick and its inside face temperature is -3ºC. Taking the coefficient of thermal conductivity of cork and wood as 0.042 and 0.20 W/m-K respectively, calculate the heat transfer per second per sq. m of surface area. SAMPLE PROBLEM: A composite furnace wall is made up of a 12 in. lining of magnesite refractory brick, a 5 in. thickness of 85% magnesia, and a steel casing 0.10 in. thick. Flue gas temperature is 2200ºF and the boiler room is at 80ºF. Gas side film coefficient is 15 BTU/hr-ft2-ºF and air side is 4 BTU/hr- ft2-ºF. Determine the thermal current Q/A. k1 = 20.5 BTU-ft/hr-ft2-ºF k2 = 0.04 BTU-ft/hr-ft2-ºF k3 = 25 BTU-ft/hr-ft2-ºF HEAT LOSSES IN BARE AND LAGGED PIPES TYPE OF FLUID IN HEAT EXCHANGER PARALLEL FLOW COUNTER FLOW PARALLEL FLOW COUNTER FLOW In a heat exchanger, hot fluid entering at the top with a temperature of 100 °C and exiting at 90 °C. Cold fluid entering at 30 °C and exiting at 50 °C. Find the LMTD for Parallel and Counter flow. EVAPORATOR HEAT LOSSES THE INHERENT TEMPERATURE DIFFERENCE BETWEEN HOT PIPES AND THE SURROUNDINGS HOW CAN WE MANAGE HEAT LOSSES? WE CAN MANAGE HEAT LOSSES BY INTRODUCING: Insulation Increase in Surface Area HEAT LOSSES OF PIPES is due to the temperature gradient between the pipe and the surroundings. THE RATE OF HEAT LOSS 𝒒𝒍𝒐𝒔𝒔 = 𝒎 [λ𝒔 + 𝑪𝒑 (∆𝑻)] 𝒒𝒍𝒐𝒔𝒕 = 𝑻𝒐𝒕𝒂𝒍 𝑯𝒆𝒂𝒕 𝒍𝒐𝒔𝒕 λ𝒔 = 𝑳𝒂𝒕𝒆𝒏𝒕 𝑯𝒆𝒂𝒕 𝒐𝒇 𝑽𝒂𝒑𝒐𝒓𝒊𝒛𝒂𝒕𝒊𝒐𝒏 𝑻𝒔 = 𝑺𝒂𝒕𝒖𝒓𝒂𝒕𝒆𝒅 𝑻𝒆𝒎𝒑𝒆𝒓𝒂𝒕𝒖𝒓𝒆 𝒐𝒇 𝑺𝒕𝒆𝒂𝒎 𝑻𝒄 = 𝑻𝒆𝒎𝒑𝒆𝒓𝒂𝒕𝒖𝒓𝒆 𝒐𝒇 𝑪𝒐𝒏𝒅𝒆𝒏𝒔𝒂𝒕𝒆 BARE PIPES are uninsulated kinds of pipes. LAGGED PIPES are insulated pipe BARE PIPE Uninsulated pipes BARE PIPE SURFACE CONDENSER ADVANTAGE OF SURFACE CONDENSER Condensate direct pumping to Boiler Can develop high vacuum suitable for high capacity plants. Require less power for water pumping System is more efficient DISADVANTAGES OF SURFACE CONDENSER It requires a large quantity of cooling water The system is complicated, costly, and require high maintenance cost It requires large floor space since it is bulky LAGGED PIPE Insulated Pipes HOW TO CALCULATE THE HEAT LOSS IN A LAGGED PIPE? A thick-walled tube is a stainless steel (A) having a k = 21.63 W/m-K with dimensions of 0.0254 m ID and 0.0508 m OD is covered with a 0.0254 m layer of asbestos (B) insulation, k = 0.2423 W/m-K. The inside wall temperature of the pipe is 811 K and the outside surface of the insulation is at 310.8 K. For a 0.305 m length of pipe, calculate the heat loss and also the temperature at the interface between the metal and the insulation. ACTIVITY 2 Identify the flow of the refrigeration system’s evaporator and condenser. Identify the type of pipes used for each component. Identify the material of the pipes and provide justification (which includes properties of the material) PUMPS PARTS OF CENTRIFUGAL PUMP (PART 2) PUMP MOTOR Converts the electrical energy to mechanical work. Provide rotational movement to the shaft. Source: The Engineering Mindset. (2020, March 1). Centrifugal Pump Basics. https://theengineeringmindset.com/centrifugal-pump-basics-2/ Motor Cover Bearing housing Fan Copper Coils Stator Protective Case Coupling Electrical Motor Rotor Motor Shaft Pump Shaft Source: The Engineering Mindset. (2020, March 1). Centrifugal Pump Basics. https://theengineeringmindset.com/centrifugal-pump-basics-2/ Pump Cover Shaft Source: The Engineering Mindset. (2020, March 1). Centrifugal Pump Basics. https://theengineeringmindset.com/centrifugal-pump-basics-2/ The fan blows ambient air over the casing to remove unwanted heat FAN FINS Source: The Engineering Mindset. (2020, March 1). Centrifugal Pump Basics. https://theengineeringmindset.com/centrifugal-pump-basics-2/ MOTOR CAN BE: - Single Phase - Three Phase Source: The Engineering Mindset. (2020, March 1). Centrifugal Pump Basics. https://theengineeringmindset.com/centrifugal-pump-basics-2/ COIL 1 STATOR COIL 3 COIL 2 Source: The Engineering Mindset. (2020, March 1). Centrifugal Pump Basics. https://theengineeringmindset.com/centrifugal-pump-basics-2/ Source: The Engineering Mindset. (2020, March 1). Centrifugal Pump Basics. https://theengineeringmindset.com/centrifugal-pump-basics-2/ Source: The Engineering Mindset. (2020, March 1). Centrifugal Pump Basics. https://theengineeringmindset.com/centrifugal-pump-basics-2/ Source: The Engineering Mindset. (2020, March 1). Centrifugal Pump Basics. https://theengineeringmindset.com/centrifugal-pump-basics-2/ DIFFERENT PHASES CAUSE A ROTATING MAGNETIC FIELD THAT DRIVES THE ROTOR AND SHAFT TO SPIN Source: The Engineering Mindset. (2020, March 1). Centrifugal Pump Basics. https://theengineeringmindset.com/centrifugal-pump-basics-2/ IMPELLER SHAFT ROTOR Source: The Engineering Mindset. (2020, March 1). Centrifugal Pump Basics. https://theengineeringmindset.com/centrifugal-pump-basics-2/ ADVANTAGES OF CENTRIFUGAL PUMP Simple and compact Easy to maintain Adaptability to a motor with High rpm Little Vibrations Flow can be controlled from full to non-discharge without shutting the pump DISADVANTAGES OF CENTRIFUGAL PUMP Poor Suction Power Usually needs priming Cavitation may develop during the operation Needs multistage to increase discharge pressure Cannot handle very viscous fluid Check valve is required to avoid backflow FUNDAMENTAL PARAMETERS FOR PUMP CALCULATION PUMP FUNDAMENTALS Hydraulic Power Total Dynamic Head Total Static Head Static Suction Lift Static Suction Head Velocity Head Pressure Head Friction Head TOTAL DYNAMIC HEAD Also known as “ PUMP HEAD” is the total amount of work needed by the pump, per specific weight of water flowing through the pump. It is the sum of the elevation head, velocity head, pressure head, and head losses. TDH = Total Static Head + Velocity Head + Pressure Head + Friction Loss NET POSITIVE SUCTION HEAD Is the term used to describe the pump cavitation characteristics. Specifically, it is the pressure in excess of the saturation pressure of the liquid being pumped. Refers to the pressure at the pump suction flange, corrected to the pump centerline, that prevents vaporization of the fluid. CAVITATION It is the phenomenon which occurs in a centrifugal pump when the pressure at any point inside the pump drops below the vapor pressure corresponding to the temperature of the liquid. This results in the formation of vapor bubbles. It is the formation of cavities of water vapor in the suction side of the pump due to low pressure. NET POSITIVE SUCTION HEAD REQUIRED (NPSHR) Is the performance characteristics of a pump and is established through close loop or valve suppression tests conducted by the pump manufacturer. Source: The Engineering Mindset. (2020, Mar 1). Centrifugal Pump Basics. https://theengineeringmindset.com/centrifug basics-2/ NET POSITIVE SUCTION HEAD AVAILABLE (NPSHA) Net positive suction head that is available or existing at the pump installation. It is equal to the pressure head of the source of fluid or atmospheric pressure. The NPSHA depends on: 1. Installation type 2. Elevation 3. Liquid Temperature 4. Liquid Boiling Point Source: The Engineering Mindset. (2020, March 1). Centrifugal Pump Basics. https://theengineeringmindset.com/centrifugal-pump- NPSHA ≥ NPSHR SAMPLE PROBLEM 1 What pump brake power is required to pump 40 lps of water through a 100mm pipe from a pump to a point 10 m above? Assume an overall efficiency of 65%. Determine the pressure intensities at points 1 and 2. Neglect losses. SAMPLE PROBLEM 2 A pump discharges 126 lps of brine (SG = 1.2). The pump inlet, 304.8 mm in diameter, is at the same level as the outlet, 203.2 mm in diameter. At the inlet, the vacuum is 152.4 mm of Mercury. The center of the pressure gauge connected to the pump discharge flange is 122 cm. This gauge reads 138 kPag. For a pump efficiency 83%, what is the power output of the motor? PUMPS WHAT ARE THE HEAD LOSSES IN THE PIPE LINE? CLASSIFICATION OF HEAD LOSSES MAJOR HEAD LOSSES - losses due to fluid friction because of viscosity and pipe roughness MINOR LOSSES - Turbulence losses due to pipe fittings and valve HEAD LOSSES Darcy-Weisbach Equation 𝟐 𝑳𝑽 𝒉𝒇 = 𝒇 𝟐𝑫𝒈 HEAD LOSSES Non-circular 𝟐 𝑳 𝑽 𝒉𝒇 = 𝒇 𝟒𝑹𝒎 𝟐𝒈 FRICTON FACTOR Hagen-Poiseuille Equation 𝟔𝟒 f= 𝑹𝒆 Re < 2000 FRICTON FACTOR Blasius Equation 𝟎.𝟑𝟏𝟔 f= 𝑹𝒆 𝟎.𝟑𝟓 Re < 105 FRICTON FACTOR Colebrook Equation 𝟏 f= 𝐷 9.3 𝟏.𝟏𝟒+𝟐 log −2 log 1+ 𝜀 𝑓 𝜀 𝑅𝑒 𝐷 FRICTON FACTOR Moody’s Equation 𝜺 𝟏𝟎𝟓 𝟏/𝟑 f = 𝟎. 𝟎𝟎𝟓𝟒 𝟏 + (𝟐𝟎 𝟎𝟎𝟎 + ) 𝑫 𝑹𝒆 SPECIFIC SPEED OF CENTRIFUGAL PUMP THE SPEED IN RPM AT WHICH GEOMETRICALLY SIMILAR PUMP IMPELLERS OPERATE TO DEVELOP ONE FOOT OF HEAD WHEN DISCHARGING ONE GALLON PER MINUTE. 𝑵 𝑸 𝑵𝒔 = 𝟎.𝟕𝟓 𝑯 Ns = Specific Speed N = Pump Speed Q = Pump Capacity H = Pump Head per stage DETERMINE THE SPECIFIC SPEED OF THE FOLLOWING SINGLE-STAGE PUMPS TOTAL HEAD, PUMP RPM CAPACITY, GPM FT A 1150 3500 100 B 885 12000 15 C 675 20000 50 D 625 9000 18 DETERMINE THE SPECIFIC SPEED OF THE FOLLOWING SINGLE-STAGE PUMPS PUMP RPM FRANCIS TYPE 1500 TO 4500 MIXED-FLOW TYPE 4500 TO 8000 PROPELLER > 8000 𝑷𝑨 𝑽 𝟐 NPSHR = + 𝒛 – Hvpg + 𝜸 𝟐𝒈 THINGS TO CONSIDER IN DETERMINING THE VALUE OF NPSHR 1. The pump manufacturer should be consulted to determine the basis of the stated values of NPSHR. 2. The pumping system designer should provide some margin above the stated NPSHR when designing for pump suction conditions. TYPICAL MARGINS OVER THE PUBLISHED NPSHR 10% TO 50% - For a simple cold water pumping system 50% TO 100% - for a complex boiler feed pumping system with transient suction operation CAVITATION PARAMETER (Thoma - Moody Coefficient) A dimensionless parameter used to predict the occurrence of cavitation 𝑵𝑷𝑺𝑯𝑹 𝒆= 𝑻𝑫𝑯 Critical values of e: Ns = 1000 rpm (e = 0.05) Ns = 2000 rpm (e = 0.10) Ns = 4000 rpm (e = 0.30) For different pump operating speed (e = 0.75) – the beginning of cavitation and the minimum safe operating value of e SAMPLE PROBLEM Assume a horizontal shaft pump handling water at 65 ºC. The suction nozzle is 4.72 cm below the pump centerline, the pressure at this point being 221 kPag and the velocity is 2.74 m/s. What is the pump’s NPSHR? NPSHA = HP ± HZ – Hvp – HL Hp = head corresponding to the absolute pressure on the surface of the liquid from which the pump draws Hz = height of the fluid surface above or below the impeller centerline Hvp = head corresponding to the vapor pressure of the liquid at the existing temperature HL = head loss because of friction and turbulence between the surface of the liquid and the pump suction flange SAMPLE PROBLEM A plant located at an altitude of 1000 m above sea level has an open feed-water heater located 3 m above the pump intake. The water in the heater has a temperature of 80 °C. If the head loss in the suction line is 0.30 m, determine the available suction head at the pump. PUMP PERFORMANCE CURVE PUMPS COMBINATIONS AND ARRANGEMENTS PUMPS IN SERIES Arrangement of two or more identical pumps used to increase the head with the same discharge PUMPS IN SERIES PUMPS IN SERIES Pump capacity: 𝑸𝑻 = 𝑸𝟏 = 𝑸𝟐 Pump Head: 𝑯𝑻 = 𝑯𝟏 + 𝑯𝟐 Pump Specific Speed: 𝑵 𝑸 𝑵𝒔 = 𝑯𝑻 𝟎.𝟕𝟓 ( ) 𝒏𝒔 PUMPS IN SERIES PUMPS IN PARALLEL Arrangement of two or more identical pumps used to increase the discharge with the same head PUMPS IN PARALLEL PUMPS IN PARALLEL Pump capacity: 𝑸𝑻 = 𝑸𝟏 + 𝑸𝟐 Pump Head: 𝑯𝑻 = 𝑯𝟏 = 𝑯𝟐 Pump Specific Speed: 𝑸𝑻 𝑵 𝒏𝒔 𝑵𝒔 = (𝑯𝑻 )𝟎.𝟕𝟓 PUMPS IN PARALLEL A pump is to discharge 10 m3/s at a head of 5 m when receiving at 300 rpm. Consider using a centrifugal pump. What will happen if the condition is be series and parallel pump arrangement? Use 5 pumps connected in series (Additional head of 3m) Use 5 pumps connected in parallel MULTISTAGE PUMP DOUBLE SUCTION PUMP PUMPS PUMPS Machines that are used to transfer liquid from a location of low elevation to a higher elevation MAJOR CATEGORIES OF PUMPS POSITIVE DISPLACEMENT NON-POSITIVE DISPLACEMENT CLASSIFICATIONS OF PUMP TYPE OF FLUID FLOW IN THE IMPELLER RADIAL SEMI-AXIAL AXIAL CLASSIFICATIONS OF PUMP TYPE OF CASING VOLUTE DIFFUSER CLASSIFICATIONS OF PUMP TYPE OF DESIGN SINGLE STAGE HORIZONTAL PUMP MULTISTAGE VERTICAL PUMP SINGLE SUCTION SUBMERSIBLE DUAL SUCTION CLASSIFICATIONS OF PUMP TYPE OF APPLICATIONS FIRE DRAINAGE DREDGE CIRCULATING SLURRY BOILER FEED DEEP WELL CONDENSATE IRRIGATION CLOGLESS FLUID PROPERTIES MASS DENSITY WEIGHT SPECIFIC WEIGHT SPECIFIC VOLUME SPECIFIC GRAVITY PRESSURE REYNOLD’S NUMBER Dimensionless parameters are used to determine the type of fluid flow 𝝆𝑽𝑫 Re = 𝝁 FLUID FLOW LAMINAR FLOW - Fluid particles move along straight, parallel paths in layer Re < 2000 FLUID FLOW TURBULENT FLOW - Fluid particles move in a haphazard fashion in all direction Re > 4000 Calculate the Reynolds number and identify the type of flow for water at 80 ºF flowing through a tube 5 in. in diameter, If the average water velocity and specific weight are 1500 cm/s and 62.4 lb/ft3 respectively. Use a value of the viscosity as 0.648 slug/ft-hr. VISCOSITY The measure of resistance to the flow of a fluid also defined as the property of a fluid that determines the amount of resistance to a shearing stress. CONTINUITY EQUATION The equation is derived from the first law of thermodynamics for a steady, open-flow system. It is used to determine the mass flow rate and the volume flow rate A1* V1 = A2 * V2 Q=𝑨∗𝑽 BERNOULLI’S PRINCIPLE The higher a fluid’s velocity is through a pipe, the lower the pressure on the pipe’s walls, and vice versa. 𝟏 𝟏 P1+𝟐ρv1 +ρgh1=P2+𝟐ρv2 +ρgh2 2 2 NON-POSITIVE DISPLACEMENT PUMP Pumps in which energy is continuously added to the fluid to increase its velocity CENTRIFUGAL JET TURBINE PUMPS CENTRIFUGAL PUMP Consists of a stationary casing and an impeller connected to the rotation shaft. Liquid enters the center of the rotating impeller leaves at a high velocity and passes to a stationary volute casing which transforms kinetic energy into pressure. PARTS OF CENTRIFUGAL PUMP PUMP SHAFT The shaft is a piece of metal where the internal parts of the pump are mounted. Use to transmit torque from the motor to the rotating parts of the pump. IMPELLERS These are the most critical parts of the pump because the capacity of the pump depends on its diameter and the speed of which it turns. OPEN SEMI-OPEN CLOSED PUMP CASING The casing is the stationary part of the centrifugal pump and it can either be volute or circular. The main function of the volute is to convert the kinetic energy acquired by the liquid into pressure. VOLUTE CASING WEAR RINGS Are fitted to the impellers and/or to the pump casing to protect the actual impeller and casing from wear. Excessive wear in the pump will reduce its efficiency, particularly in small pumps used in high- speed applications. NOTE: Wear rings should be replaced periodically to avoid damage in the impeller or casing PACKING or MECHANICAL SEAL The main function of packing or mechanical seals is to protect the pump from leakage in the area where the shaft passes through the casing BEARINGS Are used to keep the correct alignment of the shaft with other stationary parts of the pump. LABYRINTH END SEALS AND DEFLECTORS The main purpose of the labyrinth end seals and deflectors is to retain oil in the pump housing and to prevent foreign materials from entering the pump housing.