MBG 3.2 Transcript: DNA Replication PDF
Document Details
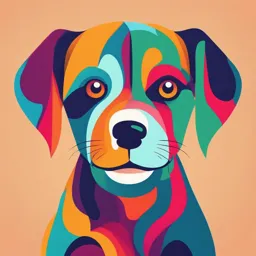
Uploaded by .keeks.
Marian University
Tags
Related
- DNA Replication PDF
- Human Genetics and Molecular Biology Notes PDF
- Molecular Biology and Genetics - Explorations: An Open Invitation to Biological Anthropology (2nd Edition) PDF
- Lesson 2: Central Dogma of Molecular Biology: Replication PDF
- Lecture 5 Biology: DNA Replication PDF
- Molecular Biology Final Exam 2021-2022 KING SALMAN Univ PDF
Summary
This document is a lecture transcript covering the basics of DNA replication. It explains directional synthesis, bidirectional replication, and the roles of enzymes like polymerases and helicases. The document also touches upon the concepts behind replication forks and the importance of specific nucleotide sequences.
Full Transcript
Okay, it is one o'clock. Let's go ahead and get started. I went ahead and put in a new blank slide so that we could just really quickly do the basics of something. And I think the first thing I want to do is kind of go back through concepts in directionality. Because I think this is one of the piece...
Okay, it is one o'clock. Let's go ahead and get started. I went ahead and put in a new blank slide so that we could just really quickly do the basics of something. And I think the first thing I want to do is kind of go back through concepts in directionality. Because I think this is one of the pieces that's the hardest thing with synthesis versus movement. If we're sort of looking at this in three- dimensional space, and I do apologize, I should have said this first, there's no video today. So, sorry. Whatever is going on with these cameras, they look like they're on, but they're not picking me up. So if there's any difficulties in the hand motions or things that I use and you're just hearing this after the fact, it's not for you guys, it's for the recording. Please let me know because I want to make sure everybody's getting the same experience regardless of whether they're in person. Anyway, when we look at this from the traditional drawing perspective, we tend to have, oh, please work. We tend to have this classic perspective, right? What if we were to instead take this and look down the ends of it, right? Where we, instead of having this flat perspective, we kind of turn it toward ourselves. What are we gonna see? We're looking right there, we have flipped it on its side and we're staring down it, so we see these two things. And we see the connections across it and really all we can see are these two ends. What are we gonna see? It's a strand of DNA, we're at the telomere. Let's say we don't have a cap to make this even more visual. We're just looking at the ends of these strands. We're gonna see the sugars, right? But we're gonna see specific ends of the sugar. We are either going to see the phosphate or the hydroxyl. It matters which one we see. Because when we are the polymerase, that's kind of the view we're getting. We're not getting it from this end right here, we're getting it as we build in here. So this, why are there places it won't let you write? Anyway, sorry, this would be the five prime end. This would be the three prime end. That is literally what it means to have directionality. When we are looking down that molecule, that's what we see. So if we're the polymerase, remember that if this is five prime, here is the opposite. But there's this happening as well. And we can draw that imaginary line and separate that. If you Google this, some of the images are misleading. And I made sure to do this after the fact, because I'm like, hmm, when that drawing didn't go so well on Wednesday, I was for sure thinking people were gonna Google this particular thing. This imaginary line is not imaginary. You do have polymerases that land and move opposite each other, because we have bidirectional synthesis. If we had an independent origin with only one direction of synthesis, we would have purely one leading strand and one lagging strand. But because we are bidirectional, we end up with two of each. And many of the drawings online will have the top different from the bottom. That is misleading. And it is based off of a unidirectional synthesis model. So when it comes to looking at this bidirectional fork, make sure you are thinking about both directions and that you're thinking of it from this perspective. What is the polymerase seeing? So if this is the five prime end, and I am landing on this molecule, I'm creating a landing pad, and I am landing on it as a polymerase, what am I gonna see? I'm seeing this base across from me that I need to put a new base in. But what I'm seeing here to catalyze as I move with the direction of the replication fork has to be that three prime hydroxyl. If it's not, if I see the five prime on my strand I'm making, if I see that five prime end, I can't move this way. I have to move the other way. Because I can only ever bring in to that three prime hydroxyl. Synthesis is five prime to three prime because I'm constantly bringing in the phosphate to the hydroxyl. So what that means is me as a polymerase, I move opposite. I hop on to a three prime end and can go the same direction. Leading strand synthesis. But down here, I can't see that. I can't see that three prime hydroxyl if I try to go with the replication fork, with the helicase movement. And so I have to constantly play catch up. And the best way to think about this is if you take, you treat both polymerases as though they're tied to the helicase. Helicase is sitting where? Right here, right? The helicase is going this way. So if I'm tied to the helicase, imaginary rope. Shh, shh, shh, shh, shh, shh. Imaginary rope, shh, shh, shh, shh, shh. And I am constantly having to run away from the helicase. That rope is gonna pull me forward and I'm gonna try to run away again and it's gonna pull me back and I'm gonna try to run away again. That's lagging strand synthesis. Because I have to see that three prime hydroxyl. Does that make sense? And while that's happening over here, we have the opposite over here. Because we have this helicase moving that way. Does that make a little bit more sense with directionality? Remember that the perspective of the polymerase is what matters. What can I clamp onto and ride along versus what I'm actually doing enzymatically? Makes sense? At least, hopefully. All right, so now we have to kind of go backwards for a sec. We're not. I didn't have any questions in here that I didn't answer for you yet. Oh, there they are, okay. Yes, here. Review from the previous lecture. How does DNA influence protein? Go for it. Yep. Gives us the instructions to make proteins. Do proteins magically appear in your system? Nope. If your cell is tasked with a job and it had to rely on all of the supplies to do that job from an external source, would it be efficient at its job? Probably not. Picture a work environment. You have to, I don't know, add a stamp to this. We still do stamps. Something we still can relate to. You have to stamp this item. Can you do that if you are never given a supply of stamps? Can you stamp something even though you have a supply of stamps but you never get the item? Stamp. Empty air like I'm doing, right? So a lot of what we think about in terms of efficiency, we gotta tie back to your cell does what it does because it can't. The DNA told it to do this job. And doing its job is literally making the supplies to carry out the action. We do need things from outside, right? You gotta eat. Because you don't have a limitless supply of things. You can't synthesize everything. But things that are absolutely essential to your basic function are often, often, things that you can make. Your supply of stamps is readily available. Does that make sense? So protein influencing DNA? DNA's organization is literally dependent on protein. Histones. Fully formed nucleosomes. Association of replication machinery. Those are proteins. Transcription machinery, proteins. For the most part, there's other pieces in there too, but still. Make sense? It's Friday and I feel like I've lost you already. How we doing? We doing okay? Okay. So that was the answer for protein. What about this next one? Can you contain information? Be transmissible, be capable of being copied, be capable of replication, and be capable of some change. The best genetic material is capable of moderate change based on environmental needs. Bacterial genomes work for bacteria because they have a much more mutatable, can take a new information system that lets it survive its conditions. We need our system to be what? Pretty consistent. For individual organism, we don't want a lot of change. We want that variation to occur where? The next generation, right? Our gametes get to have variation. Mitotically, our cells should all be identical, right? Okay. What is the form for DNA? I had a great question about Z form. And I want us to, what I didn't talk about is the fact that Z form is often the consequence of things we are doing actively. Our DNA is never structurally limited. But I didn't want you confused. B form is necessary for these processes to take place. Z form is often a form that kind of gets picked up as a process occurs. So that I hope, I just didn't want us to get confused with that. B form is our main form. But we do see these other forms happening as a result of things we are doing. I just didn't want us to get confused. But I had some great questions about that. So I wanted to add that clarifying point. Makes sense? And what about this last one? Specifically linked to changes in melting temperature, right? Where we have that un annealing, that separation of strands. The more hydrogen bonds we have, the less likely we are to separate easily at a low temperature. DNA likes to be double-stranded. RNA likes to do complimentary bonding. Hydrogen doesn't like to hang out by itself. It wants partners and friends. It's what makes water so amazing. The difference in electronegativity, all that jazz. Makes sense? Okay. Moving on. All right, so this is our, if you were looking for the bare minimum for replication fork, being able to do topoisomerase, helicase, and our polymerases. That's your bare minimum. When it comes to places we're going, we really do need to be able to pay attention to DNA ligase and stability in the form of single-strand binding proteins. But this is a basic bacterial replication fork. It is going to have very simple polymerase differences where we have leading versus lagging-strand polymerases. We are still gonna use single-strand binding proteins and our upstream topoisomerase to relieve that strain. That make sense? For another way of looking at this, part of the reason we have these different polymerases is because we have that RNA segment. Primase lays an RNA primer. DNA polymerase cannot land on single-strand DNA. It has to be double-stranded. And so when it comes to viruses stealing our machinery, oftentimes they will fold their genetic material or have to be already double-stranded for this to work. Or they have to encode some of their own little bits of machinery depending on what we're talking about. Polymerases have restricted functions. DNA polymerase needs to see a hybrid double-stranded molecule. But do we want all those bits of RNA in our DNA? No, so we have to have a means to get rid of it. And so some of our polymerases can not only lay those primers, but also can chop them off. And we call that exonuclease activity. And so what you will see for this molecule, Paul I, our friend over here, is removing that primer and helping to get us set up for a complete strand. We have human counterparts to this. We have human counterparts to Paul III. Paul III is the main strand synthesis molecule for prokaryotes. But we still have this origin of replication, the bidirectionality where we have leading and lagging strands going both directions because this is this direction, that is that direction, heel cases are moving that way. The basics are the same. So what does that tell us about this process? If every organism from bacteria through humans, who I always put at the top of things, what does that tell us? Highly conserved process means that it's the most efficient way to do it. It's the best. It's what works. It's a highly conserved, highly essential process. I can't get rid of it without having disastrous consequences. You can't copy your genetic material. You're not making a new generation from the cellular level to the organismal level. If you can't copy it, that's it. Does that make sense? So how does this work? Well, we have a lot more polymerases in humans, but these essential functions are the same. Make the new piece of DNA, get rid of or lay and then get rid of the primer. What do I have to do? Faithfully copy the entire molecule. So I've got lots of ways to do that, but I'm still gonna have helicases, topoisomerases. I have a lot more with people, but we're only gonna talk about one and two. This may look more complicated, but the pieces are the same. Primase, lay a primer, helicase, topoisomerase, polymerase, ligase. I don't know what that was, but that's okay. All right, so let's talk about primers. Primers are bits of RNA that we use to create a signal for lay and hear polymerase. They are typically laid by primase. What this does is give that polymerase that three prime hydroxyl that we need to see. It tells me lay and hear and this is the direction you need to go. Here's how you're gonna do that synthesis. When it comes to the final product, we gotta get rid of it. And so in the lagging strand synthesis, what we will typically do is kind of move along to the previous strand and eat up that primer, laying a new set of bases. We're gonna literally cleave off that primer, pushing it like a snow shovel and filling in the space. So origins of replication are those bubbles you've seen, right? Now we're gonna talk about how they differ. Prokaryotes, really simple. What kind of genome do they have? Bi-directional synthesis of a circle would involve opening it and copying it, right? So you kind of get two overlapping circles that then come apart. We can also have rolling circle models, which we'll get into in a little bit, but copying a circle is pretty easy. They can do that, it's a small genome. I mean, it's a tiny circle. So they can only need really one origin. We need many. We need many, many, many origins. And the linear nature of our genetic material means we have other considerations as well. But the basic process is always gonna be the same. I've gotta open the DNA. I've gotta build the actual pieces. I gotta get the polymerase in there. I've gotta be able to unwind the DNA with little strain and I gotta make the copy. Opening the DNA is consistent for all origins. We're gonna talk about that in a little bit. All this is showing you is that doing this job from a single origin isn't possible for humans. It takes multiple replication forks to get all this making a copy. I'm kinda glad that wasn't recorded. Because most of you weren't looking and that's for me. I might have done a little. Trying to keep you awake, it's Friday. So, multiple origins. Why do we know that? Because we've literally radio labeled the DNA. We have literally radio labeled bases and looked at their incorporation. We have actually been able to visualize the opening of multiple replication forks. We call them replication units. And when it comes to how this is incorporated in the cell cycle, we have within S phase what we call late and early replicating units. Regions of replication are specific to the needs of the cell. A liver cell is going to have different genes on than a skin cell, yes or no. Otherwise, they would function the same, right? That's how we give them differential functionality. So, if there is a particular gene that I need to define skinness, do I want that to be accessible for as much as possible if I'm a skin cell? Do I care if I'm a liver cell? So, when it comes to these replicating units, we have a differential preference. We need the stuff that we're using to remain accessible and the stuff that we're not using, we don't really care about. And it turns out this actually ties to origins of replication. When you are a key gene actively being transcribed, your origins are carefully organized. You will open outside the reading frame of that gene. You will be carefully positioned to not disrupt that gene and you're gonna replicate early because this will actually give us more copies to work with. It will give us more accessibility. If you're especially important to the process of replication, like the actual cell division process, you're also gonna do this early. But if you are late in your replicating, your origin could be anywhere, completely randomly organized. We are not yeast, we don't have set sequences. We have random dispersal. But if we tie this back to the skinness argument, I've really gotta come up with a better word for that, we tie it back to that, it makes sense. Are all your cells the same? In terms of genetic information, they should be. But how we're using that information, no. And so if we had strict set origins, the replication timing could never be proportionate to the needs of the cell. So it makes sense that we don't have a sequence limitation in the way that a yeast does. Does that make, we follow in? So instead, our origin of replication, our replication is tied to transcriptional activity. Remember, at no time is your cell transcriptionally inactive. It is always part of the process. So nice distinct between versus random distribution. All origins are seen at the same time. But the process of initiating replication is twofold. I have to label all of the origins, then I have to bring in all the machinery and fire. So we have two steps, licensing and firing. But licensing really has two parts. All origins are found at the same time. Origin, recognition, complex, ORC. The orcs hunt down the hobbits. And they're all found at the same time. Frodo doesn't get away in this version. But how they're impacted depends on when they're going to fire. And so we see them all, we call in helicase. So, ORC, we call in helicase, CDT1 and CDC6, and that is our licensed origin. I see you, happens to all of them at the same time. I see you, you can't get away. I know you're there. The IRS are on is all knowing. Lord throwing a show of friends, okay. But we know Frodo gets away, right? He doesn't get caught by orcs until we're what, in Mordor? Because, wow, that's some bad observation there, right? So he's going to fire differently than Pippin and Merry who get caught, like what, right away? We're not lovingly, we feel like it's connecting, we'll see. That firing is different. And how is it different? Because you call in new things. Saruman caught Pippin and Merry, right? I'm trying. So, that is the second part of licensing. That's the pre- initiation complex. And that is going to involve here and here, all of those components we've been talking about. I am now in the position where as soon as I'm ready, I can go. So, pre-recognition complex is all about finding all the origins, and they all get recognized at the same time. Pre-initiation complex is right before firing. And that doesn't form until we're ready to go. It's part of being ready to go. So, licensing and firing, pre-replication involves that orc finding our hobbits, bringing in some help in the form of our HeLa case, our CDT1 and CDC6, right? That's our pre-replication complex. But only when we've actually nabbed our hobbits do we run. Does that make sense? And that involves bringing in some orichi, if you will. Did that actually help? I was trying something new with that. So, okay. If I am transcriptionally active, I can't have an origin in the middle. Why? Just look right there. What did that origin do? So, this is the ORC, the orc finding the origin and calling in some friends. What is that? If I am another molecule coming into this mess, what would that look like to me? Do I see a nice, pretty piece of DNA there? No, I see a bunch of stuff piled in that spot, right? If this is a parking lot, am I gonna park there? So, this can't be within the transcriptional reading frame if I need that gene to still be active because I'm literally gonna prevent RNA polymerase from landing. So, that can be another way to sort of tie that in. This happens no matter what gene you are. They're all gonna be found at the same time. So, if I need to be active, I can't have this in the middle of my gene because the next step makes it even more complicated and that's our machinery. So, I know there's a lot of words there, but MCM is the helicase. This is the pull that's polymerase. These are associated proteins that help the polymerase, but we're not getting into any of those. CDT1 and CDC6 are part of the pre-recognition complex. Our big pieces are still there. And once we fire, we properly replicate. But this pre-initiation complex correlates with firing. It's all part of licensing, but once we have this, we can go. We've nabbed our hobbits and we're on the run. And much like in the movie, we could run two different directions and not doing it well. Anyway, message I'm gonna hit home for this. Synthesis is always five prime to three prime because of that three prime hydroxyl. How we move and how we synthesize are two different parts of what we are. Some of our polymerases can move backwards. And when they move backwards, they're gonna be cutting things out from where they were. It's called exonuclease activity. So rolling circle, circular genomes, super easy. I can cut one strand and I can just move along, get that going and make two perfect copies. Where is the replication fork? It's actually right here. There's your replication fork. If we think about it like this, it might make a little bit more sense. So where's our movement? Well, it's this way. Does that make sense? When you have single-stranded DNA, the rolling circle model creates situations where you have to resolve two connecting circles. And the resolution of those two connecting circles can be random, increasing the hypermutability. What's the most stable form of DNA? Which is more immutable? This is part of that. If I have two circles stuck together and I need to resolve that, I could have that cut point very slightly. Even though I have molecules that are gonna help me do that, there could be variation there. Single- stranded material can also do what is called rolling hairpin replication. This is going to be for our linear because single-stranded DNA, does it like to be single- stranded? So it's gonna complimentary base, but the answer is no, sorry, people were shaking their heads, forgot recording. They're going to fold in three- dimensional space in complimentary base pair. And so this rolling hairpin model is the formation and resolution of complimentary pieces, allowing you to more faithfully copy the whole molecule for those linear pieces, but still have that same problem of, where do I resolve it at? Am I copying the right component? And if you look at that bottom picture, you can see the little merger of old and new. How do I resolve that? And so single-stranded material, whether circular or linear, does have the potential for further mutation because our number one source of mutation is replication. All right, polymerases, the enzymes of replication. Can I do anything without an enzyme? Not efficiently, not well. And in terms of replication, no. Because the job of the enzyme in replication is to say, oh, hey, I know what friend you should have and bring it in and synthesize a backbone. Right? Replication is literally creating a strand of connected nucleotides. A nucleotide is the base, the sugar, and the phosphate. Okay, so, Paul won for our prokaryotic friends. They have very few polymerases. They only have five. We're only gonna talk about two. Please don't memorize the pieces of this. We're not, I'm not gonna ask you what theta does. Okay? I'm showing you its structure so you can get a better sense of that. I grab the genetic material and I slide. It's a sliding clamp polymerase. See, isn't it pretty? Component's done. What I do want you to know is one versus three. One has different exonuclease activity than three because three is all about elongation. Remember that replication has three major stages. Initiation, elongation, and termination. All of these molecular processes have this. Initiation was all about the origin. Elongation is all about the action of calling in new nucleotides and synthesizing the new strand. Paul one has the job of pulling off some of that RNA. It is not functioning in the same way as Paul three. Paul three we could designate as the main polymerase. Big air quotes on that. So that's why I'm showing you the very unique structure of Paul three because alterations in any of those components is going to impact function. These are highly conserved genes. That being said, we have 15 that we know of and they have highly specific functions. We are only gonna talk about a main four with really emphasis of trying to make connections to our prokaryotic friends. We have Paul alpha, which is really going to resemble our Paul one. And then we have Paul delta and epsilon. These are our main polymerases. And you'll see that it says lagging and leading strands, they work together. And then we have Paul betas and a few others that are all about repair. But our delta epsilon is really gonna be like Paul three. Paul three delta epsilon main polymerases. Great, polymerase is doing its job. We've spent some time on how it lands with that primer needing to be there. We've talked about its actual process. It is so wonderful that I'm just hopping along and making new bases and I'm just doing that new strand. If I didn't have this, I'm gonna get stuck. Topoisomerases are critical to enabling the molecule to withstand the force of being opened. HeliCase opens us. Topoisomerase makes it so possible for HeliCase to keep moving. But to do its job, it's either going to nick one strand and unwrap or both strands and unwrap. This creates potential for error. The backbone of DNA is supposed to be solid or all chopped up, solid. Double strand breaks are bad. Single strand breaks are bad. Here you have a molecule that is part of replication that is literally breaking the backbone. If it falls off, you have error. That is literal endogenous DNA damage, which we will summarize later. Topoisomerases, one, one nick. Topoisomerases, two, two nicks. So I see a single strand break upstream of a replication fork, but no topoisomerase. Which topoisomerase likely fell off? Single strand break. Topois, one nick, one strand broken, topois. I see a double strand break upstream of my replication fork. What fell off? Topois, two. Okay, we've talked about telomeres. Why do they matter? Gotta make the cap. Why do we need them long enough? Because they protect the ends of genetic material. And the reason they're there is the end replication problem. How'd my polymerase get on the genetic material? There was a primer that said, here, land here, and then it landed. It's gonna move along differently when it can move the same direction as the replication fork than when it has to move against, right? That I'm tied like a string and I keep pulling one of them off. This action, this need for a primer and this inability to consistently synthesize leads to part of the chromosome not being capable of proper replication. I literally lose material from the ends of the chromosome. After about 30 generations for this, I am critically short in humans. And what does it mean to be critically short? No cap. I can't twist back in and create that little shoe. So I have to have this buffer because of replication. So there's our chromosome level tie-in. I need the sequences at the end of my linear strands to be highly repetitive because I'm gonna do a bad job copying it because my polymerase can only do so much. Does that make sense? So the literal end replication problem is I cannot copy the end. Now, we also have this presence of material. And I said, Paul one for prokaryotes could kind of, you know, slide in like a snow shovel and sort of plow off that RNA primer. You have something similar in humans for our polymerases. And what it will do is it will come along and it will actually help displace that RNA primer, but then we have to process it. If it's too long, we're gonna have to call in additional machinery to cleave that backbone and get it out of there because our exonucleus activity is really more about fixing our own mistakes, not fixing something somebody else did. Our polymerases are really good at proofreading themselves. Our main polymerases, Delta and Epsilon, are really good at saying, I did a good job, or ooh, let's rewind that. They are not so great at fixing something else that's in their way. Does that make sense? So to do this, we often have to have additional processing. And so one of the things that we call in is called flap endonuclease. Endo or exonucleases are all about cutting backbones. And in this case, we are going to remove within the plane of the system, so endonuclease, we're gonna remove this piece. If we have way too much material, we may need additional nuclease activity to help us kind of deal with that, and that's where DNA2 comes into play. Does that make sense? So ultimately, all of this is about getting rid of that RNA primer so that we're DNA on DNA. But then what does every backbone need at the end, whether we're lagging strain synthesis, or getting two parts where the replication fork went down our imaginary line and went that way? What do we have to call in? Ligase. We've gotta get DNA ligase to fix that backbone. Much of the repair machinery we're gonna talk about next lecture is also gonna end with ligase. So what would a mutation in ligase mean? Not fixing some stuff, that's a problem. Breaks in the backbone are damage. Single-strand breaks, damage. Double-strand breaks, damage. Critically short tail mirrors appear as double-strand breaks. Does that make sense? Okay, questions. Do we wanna wrap up here with questions or bring those back to the beginning next time like we just did? Next time? All right, close your eyes. Ah, there's more. Sorry. All right, take a breather and move on to the next section in the last five minutes, because I've kind of set the stage for it. Damage and repair. Why do we fix DNA? Existentially, why do we fix it? Let's put on our philosophical hats. Because we want some variation, right? Doesn't damage sound like the perfect way to induce variation? No, it doesn't at all. Please do not link those two things. Damage is bad because, why do we say damage is bad? Because it prevents function. DNA has one purpose, and that is to indirectly control everything you do. If you hurt it, if you break it, if you prevent it from functioning, you are going to impact everything you try to do from a cellular and organismal perspective. So, since we expect damage, we have to have a means of fixing it. Tealameres that get short look like what? Double-strand breaks. I need to be able to fix double-strand breaks because I literally have mechanisms that make them. Topoy summaries two does what? Cuts both strands of the DNA. Literally breaks the backbone in a double-strand break methodology. Anytime we use the term mutation, and I know that there are times where we talk about a mutated protein, that's not technically accurate description. It creates a visceral image, which is why we use it, but mutation literally refers to changes in the bases. Either the base itself, the base sequence, is a change in DNA. That is mutation. Single nucleotide polymorphisms that are the result of replication error are mutations. X- Men, sure. We'll pretend that's possible. We're not liking my science fiction references today. So, when it comes to mutation and its impacts, we can have structural or functional impacts. When we affect the ability of a DNA to build a chromosome, that's gonna be very much structural. I am literally affecting the way this genetic material is behaving. When it comes to within reading frames or within regulatory elements of key genes, we are now talking about functional products being affected. I am supposed to put ATCG together so that I build this domain to this protein. It needs to be ATCG for this domain to work. If I change that sequence, I affect this domain. That is within the reading frame. I even can alter the reading frame for that, but that is going to be within coding regions. But outside of coding regions, I can still impact function by preventing me from ever making that domain. If I affect the ability to read ATCG and build the protein, I have also impacted function. I've also lost that domain. Make sense? We will spend a lot of time with regulatory elements. When it comes to how we talk about mutation, we really have three categories. Pathogenic, unclear, and benign. A benign mutation is considered normal human variants. Brown hair versus blonde hair. Blue eyes versus green eyes. Normal human variation. deleterious pathogenic mutations. They cause disease. They cause phenotypes. And in this category of the word phenotype, it doesn't just mean what you see, but a physical appearance that equates to disorder. And then we have levels of that. But if you say lethal, that has a connotation, that's going to fit under pathogenic. If you say beneficial, that's going to be benign. Does this make sense? When you have mutation, you have three things that that mutation can do. It can increase function, it can leave the function the same, or it can decrease function. And what you do as a gene will confer whether those changes have a disorder impact or a disease impact. If turning me on is good, turning me on as a gene is good, then having a gain of function mutation will be beneficial. I am P53, I'm controlling things. I want to be on to prevent damage. That's a beneficial change. But have a loss of function to that same gene, now I have a deleterious change. So the same gene can have different change impacts. And so we must think, what is the variation? How is it impacting my function? And what do I do so that that change of function has an actual consequence? Turn on genes that are doing bad things, that's going to be a problem. But turn them off, that's going to be beneficial. And vice versa for the opposite. So we will talk about this from a perspective of cancer, but the last piece that I'll say in my last 10 seconds, if I have the change in my somatic cells, it's only going to impact me. If I have the change in my germline, gametes, any of my reproductive cells, I have the potential to affect the next generation. Spontaneous versus induced. Replication is our number one source. That causes spontaneous error. Induced applies to changes that are due to factors we can't control. Exogenous sources. All right, when we come back, we will have more fun with breaking DNA only to fix it. Thank you so much. Happy Friday.