Advanced Production Technologies II PDF
Document Details
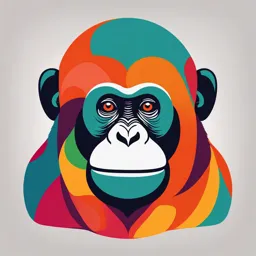
Uploaded by ProtectiveFlute
Universidade do Porto
Abílio de Jesus
Tags
Summary
This document covers advanced production technologies II (Tecnologias Avançadas de Produção II), specifically focusing on the machinability of metals. The document delves into definitions, criteria for assessing machinability, and potential challenges in evaluating this property. It also encompasses a laboratory assessment of metals and examines orthogonal cutting operations and cylindrical turning operations.
Full Transcript
Outlook Machinability o Definition Advanced Production Technologies...
Outlook Machinability o Definition Advanced Production Technologies II o Criteria to assess machinability (Tecnologias Avançadas de Produção II – TAP II) o How difficult could be the machinability evaluation? o Machinability rating o Machinability rating calculation example Machinability of Metals o Machinability of common materials o Proposed machinability problem Master in Mechanical Engineering – M.EM Laboratory on Machinability Assessment of Metals 2024-2025 o Orthogonal cutting operations o Cylindrical turning operations Abílio de Jesus Advanced Production Technologies II AMPJ 1 Advanced Production Technologies II AMPJ 2 1. Machinability 1. Machinability Definition(s) Criteria to assess machinability Denotes the relative easy a material can be machined using appropriate tooling and cutting Tool life/ tool wear conditions (M.P. Groover) Forces and power The easy a material can be machined to an acceptable surface finish. Surface finish The ability of a material to be machined under given set of cutting conditions. Easy of chips disposal www.dajinprecision.com Materials with good machinability… - Require little power to cut; - Can be cut quickly (high MRRs); - Easily obtain a good surface finish; - Do not wear the tooling too much. Advanced Production Technologies II AMPJ 3 Advanced Production Technologies II AMPJ 4 1. Machinability 1. Machinability How difficult could be the machinability evaluation? How difficult could be the machinability evaluation? Machinability generally refers to the work material, but it depends on more that just the work material (many variables), making its evaluation complex. Work material is the main factor affecting machinability Work material Strength o Hardness: Impacts on tool life – higher hardness results in lower tool life and lower Hardness hardness results in higher tool life; Chemical Composition Microstructure o Strength: higher tensile strengths result in higher shear strength -> higher cutting forces, Heat treatment specific energy and temperatures -> material more difficult to machine; Cutting tool Machinability Machine-tool o Ductility: high ductility may cause in poor finish and problems with chip control /disposal. Tool type Tool life Stiffness Cutting edge geometry Surface finish Accuracy/Precision Tool holder (fixation) Cutting forces Lubricant/cooling Tool material Chip control Cutting parameters Workpiece Geometry (shape and dimensions) Stiffness of holding Operation type Advanced Production Technologies II AMPJ 5 Advanced Production Technologies II AMPJ 6 1. Machinability 1. Machinability How difficult could be the machinability evaluation? Machinability rating Work material is the main factor affecting machinability Machinability testing involves usually a comparison of work materials. o Chemical composition Machining performance of a test material is compared with that of reference (base/standard) ✔ Addition of some elements to increase strength and hardness will have negative material. impact on machinability; Possible measures of performance in machinability testing: ✔ Some elements (e.g. lead, sulphur, tin, bismuth, selenium, tellurium and/or phosphorus) are added to increase machinability (so-called free cutting steels). o Tool life ❖ lubrication: reducing friction, forces, temperature and BUE and better tool life o Tool wear and surface finish; o Cutting force ❖ improved chip control for easier chip disposal; o Power in the operation ❖ Lead is less-frequently used today due to environmental and health concerns; o Cutting temperature ❖ Bismuth and tin elements are becoming more popular as additives for environmental reasons; o Material removal rate under standard cutting conditions ❖ free cutting steels are more expensive but extra costs pay for themselves due to higher production rates and longer tool lives. Advanced Production Technologies II AMPJ 7 Advanced Production Technologies II AMPJ 8 1. Machinability 1. Machinability Advanced Production Technologies II AMPJ 9 Advanced Production Technologies II AMPJ 10 1. Machinability 1. Machinability Machinability rating calculation example Machinability rating calculation example A machinability rating is to be determined for a new work material using the cutting speed for a 60 A machinability rating is to be determined for a new work material using the cutting speed for a 60 min tool life as the basis of comparison. min tool life as the basis of comparison. For the base material (B1112 steel), test data resulted in Taylor equation parameter values of n=0.29 and C=500, where speed is in m/min and tool life is min. For the base material (B1112 steel), test data resulted in Taylor equation parameter values of n=0.29 and For the new material, the parameter values were n=0.21 and C=400. These results were obtained using C=500, where speed is in m/min and tool life is min. cemented carbide tooling. (a) Compute a machinability rating for the new material. (b) Suppose the machinability criterion were the cutting speed for a 10 min tool life rather than the present criterion. For the new material, the parameter values were n=0.21 and C=400. These results were obtained using Compute the machinability rating for this case. (c) Compute the machinability rating for the new cemented carbide tooling. material considering the tool life criterion assuming a cutting speed of 200 m/min. (d) What do the results of the calculations show about the difficulties in machinability measurement? (a) Compute a machinability rating for the new material. (a) (b) Suppose the machinability criterion were the cutting speed for a 10 min tool life rather than the present criterion. Compute the machinability rating for this case. (c) Compute the machinability rating for the new material considering the tool life criterion assuming a cutting speed of 200 m/min. (d) What do the results of the calculations show about the difficulties in machinability measurement? Advanced Production Technologies II AMPJ 11 Advanced Production Technologies II AMPJ 12 1. Machinability 1. Machinability Machinability rating calculation example Machinability rating calculation example A machinability rating is to be determined for a new work material using the cutting speed for a 60 A machinability rating is to be determined for a new work material using the cutting speed for a 60 min tool life as the basis of comparison. For the base material (B1112 steel), test data resulted in min tool life as the basis of comparison. For the base material (B1112 steel), test data resulted in Taylor equation parameter values of n=0.29 and C=500, where speed is in m/min and tool life is min. Taylor equation parameter values of n=0.29 and C=500, where speed is in m/min and tool life is min. For the new material, the parameter values were n=0.21 and C=400. These results were obtained using For the new material, the parameter values were n=0.21 and C=400. These results were obtained using cemented carbide tooling. (a) Compute a machinability rating for the new material. (b) Suppose the cemented carbide tooling. (a) Compute a machinability rating for the new material. (b) Suppose the machinability criterion were the cutting speed for a 10 min tool life rather than the present criterion. machinability criterion were the cutting speed for a 10 min tool life rather than the present criterion. Compute the machinability rating for this case. (c) Compute the machinability rating for the new Compute the machinability rating for this case. (c) Compute the machinability rating for the new material considering the tool life criterion assuming a cutting speed of 200 m/min. (d) What do the material considering the tool life criterion assuming a cutting speed of 200 m/min. (d) What do the results of the calculations show about the difficulties in machinability measurement? results of the calculations show about the difficulties in machinability measurement? (b) (c) Advanced Production Technologies II AMPJ 13 Advanced Production Technologies II AMPJ 14 1. Machinability 1. Machinability Machinability rating calculation example Machinability of common materials A machinability rating is to be determined for a new work material using the cutting speed for a 60 Approximate values of machinability ratings (M.P. Groover) min tool life as the basis of comparison. For the base material (B1112 steel), test data resulted in Taylor equation parameter values of n=0.29 and C=500, where speed is in m/min and tool life is min. For the new material, the parameter values were n=0.21 and C=400. These results were obtained using cemented carbide tooling. (a) Compute a machinability rating for the new material. (b) Suppose the machinability criterion were the cutting speed for a 10 min tool life rather than the present criterion. Compute the machinability rating for this case. (c) Compute the machinability rating for the new material considering the tool life criterion assuming a cutting speed of 200 m/min. (d) What do the results of the calculations show about the difficulties in machinability measurement? (d) Material is the same but the MRs show different values, even reversed trends, due some changes in the machinability criteria. This is a clear example on how machinability ratio could be difficult to evaluate. MR always defined for a particular set of conditions! Advanced Production Technologies II AMPJ 15 Advanced Production Technologies II AMPJ 16 1. Machinability 1. Machinability Machinability of common materials Machinability of common materials Approximate values of machinability ratings (M.P. Groover) Steels – carbon content of steel significantly affects its machinability o High carbon steels -> difficult to machine since they are harder and contain carbides; o Low carbon steels –> may be troublesome because they may be too soft (BUE); o Medium carbon steels (~0.2% C) -> best choice regarding machinability. Stainless steels – show poor machinability compared to regular carbon steel Easy formation of BUE; Work harden very significantly; Lower heat conductivity; Tend to form serrated chips; AISI 303 and AISI 416 – easier to cut due to the addition of sulphur and phosphorus. Advanced Production Technologies II AMPJ 17 Advanced Production Technologies II AMPJ 18 1. Machinability 1. Machinability Machinability of common materials Proposed machinability problem Aluminium alloys – generally very easy to machine Tool life turning tests have been conducted on B1112 steel with high-speed steel tooling, and the resulting parameters of the Taylor equation are: n=0.13 and C=225. B1112 is the base metal and has a o Softer grades tend to form BUE -> poor surface finish; machinability rating = 1.00 (100%). During the tests, feed = 0.254 mm/rev, and depth of cut = 0.254 o High cutting speeds, high rake angles and high relief angles are recommended for proper mm. Based on this information, and machinability data given in slide 16, determine the cutting speed machinability; you would recommend for the following work materials, if the tool life desired in operation is 30 min o AA2007, AA2011 and AA6020 have specially good machinability. (the same feed and depth of cut are to be used): (a) C1008 low carbon steel with 150 Brinell hardness, Non-metallic materials - Thermoplastics (b) 4130 alloy steel with 190 Brinell hardness, and (c) B1113 steel with 170 Brinell hardness. o Difficult to machine due to poor thermal conductivity; o Heat built up in the cutting zone will degrades tool life and locally melts the plastic Non-metallic materials - Composites o Often have the worst machinability o Combines the poor thermal conductivity of plastic resin matrix with an abrasive matrix Advanced Production Technologies II AMPJ 19 Advanced Production Technologies II AMPJ 20 2. Laboratory on Machinability Assessment of Metals 2. Laboratory on Machinability Assessment of Metals Objectives Orthogonal cutting operations To compare the machinability of different metallic alloys under two different cutting operations; o Steel, AL alloy, Brass alloy tool o Orthogonal cutting (2D cutting) chip o Cylindrical turning (3D cutting) workpiece o Instrumented tests aiming at measuring the cutting forces Cutting direction Orthogonal cutting: cutting speed Shaping machine equipped with load-cell and DAQ system orthogonal to cutting edge Load measurement: Three-component piezoelectric dynamometer (Kistler 9257B) + charge amplifier (Kistler 5070A) + Data acquisition system (Advantech 4711A) + PC Advanced Production Technologies II AMPJ 21 Advanced Production Technologies II AMPJ 22 2. Laboratory on Machinability Assessment of Metals 2. Laboratory on Machinability Assessment of Metals Orthogonal cutting operations Orthogonal cutting operations Orthogonal cutting experimental setup (a); tool slide lever (b); specimen fixation vise (c); Mitutoyo toolmaker’s microscope (d). Insert geometries (WC-Co) used in the orthogonal Uncut chip thickness (t0) measurement procedure cutting tests with 5 and 15º rake angle. Advanced Production Technologies II AMPJ 23 Advanced Production Technologies II AMPJ 24 2. Laboratory on Machinability Assessment of Metals 2. Laboratory on Machinability Assessment of Metals Orthogonal cutting operations Orthogonal cutting operations Specific cutting pressure Values of specific cutting pressure for selected work materials using sharp cutting tools and chip thickness before the cut to = 0.25 mm (0.010 in) [M.P. Groover]. tool chip workpiece Fc: Cutting force; Ft: Thrust force; Advanced Production Technologies II AMPJ 25 Advanced Production Technologies II AMPJ 26 2. Laboratory on Machinability Assessment of Metals 2. Laboratory on Machinability Assessment of Metals Orthogonal cutting operations Cylindrical turning operations Tentative testing plan - 2 tools (carbide inserts); - 3 uncut chip thicknesses (t0); 9 tests - 3 materials (steel, Al alloy, brass alloy) - 1 cutting speed Output data Machinability - 3 forces components (cutting and thrust) vs. t0; comparison Scheme of the turning setup: (a) front view and (b) top view of tool-workpiece relative position Advanced Production Technologies II AMPJ 27 Advanced Production Technologies II AMPJ 28 2. Laboratory on Machinability Assessment of Metals 2. Laboratory on Machinability Assessment of Metals Cylindrical turning operations Cylindrical turning operations Tentative testing plan - 1 tool (carbide insert); workpiece - 1 depth of cut (ap=0.5 mm); 18 tests - 3 materials (steel, Al alloy, brass alloy); - 3 feed values (f) (0.05 mm/rev; 0.1 mm/rev; 0.2 mm/rev); - 2 spindle frequencies (n) (2500 rev./min; 1250 rev/min). Three-component piezoelectric dynamometer (Kistler 9257B) Output data Machinability chip - 3 forces components (cutting, feed and penetration); comparison Fc: Cutting force; - surface roughness. tool Ft: Feed force; v= cutting speed; f= feed; p=ap=penetration; Portable Hommel Tester T500 Advanced Production Technologies II AMPJ 29 Advanced Production Technologies II AMPJ 30 2. Laboratory on Machinability Assessment of Metals 2. Laboratory on Machinability Assessment of Metals Cylindrical turning operations Chip analysis Continuous chips tool tool BUE workpiece workpiece Good surface finish Traces of BUE in the new surface rε=tool tip radius fn=feed Advanced Production Technologies II AMPJ 31 Advanced Production Technologies II AMPJ 32 2. Laboratory on Machinability Assessment of Metals 2. Laboratory on Machinability Assessment of Metals Chip analysis Chip analysis (ISO 3685) Serrated chips Fragmented chips High shear deformation tool localization tool Low shear deformation localization workpiece workpiece Rough surface Advanced Production Technologies II AMPJ 33 Advanced Production Technologies II AMPJ 34 2. Laboratory on Machinability Assessment of Metals 2. Laboratory on Machinability Assessment of Metals Orthogonal cutting vs. turning How to use orthogonal cutting tests to estimate friction and material strength? Turning Orthogonal cutting Feed, f Chip thickness, t0 tool tool chip Penetration, p Cut width, w chip workpiece Feed force, Ff Thrust force, Ft Same nomenclature for remaining parameters chip workpiece workpiece workpiece tool chip Groover, M. P., 2010, “Fundamentals of Modern Manufacturing. Materials, Processes, and Systems, 4th edition, John Wiley & Sons, Inc. tool Advanced Production Technologies II AMPJ 35 Advanced Production Technologies II AMPJ 36 2. Laboratory on Machinability Assessment of Metals How to use orthogonal cutting tests to estimate friction and material strength? Advanced Production Technologies II tool (Tecnologias Avançadas de Produção II – TAP II) tool chip chip Machinability of Composites workpiece Fibre Reinforced Plastics (FRP) Drilling workpiece Master in Mechanical Engineering – M.EM (Experimental analysis) (Merchant equation) Shear 2024-2025 angle Abílio de Jesus Groover, M. P., 2010, “Fundamentals of Modern Manufacturing. Materials, Processes, and Systems, 4th edition, John Wiley & Sons, Inc. Advanced Production Technologies II AMPJ 37 Advanced Production Technologies II AMPJ 1 Outlook Introduction Introduction Fiber Reinforced Plastics (FRPs) are finding increasing applicability in high-tech industries which is the case of Challenging of FRPs drilling the aeronautics/aerospace and automotive industries due to material unique properties: Conventional drilling of FRPs – High specific strength and stiffness; High performance drilling methods – Low density; – High damping properties; Cutting fluids – High stability; Boeing 787 – High corrosion resistance. Quality assessment Safety Issues on FRPs Machining Most of FRPs parts are produced in ready-to-shape manner using different manufacturing processes: References – Molding; – Vacuum bagging; – Compression molding; – Filament winding; – Hand-lay-up laminating techniques; BMW – … Advanced Production Technologies II AMPJ 2 Advanced Production Technologies II AMPJ 3 Introduction Hole drilling of FRPs Introduction Machining of FRPs is still required: Most important machining processes for FRPs: – Meet dimensional tolerances; – Hole making (e.g. drilling); – Produce features hard to mould such as Commercial aircraft requires about 1.5~3 million holes Trimming with PCD grooving insert pockets or special surfaces; – Trimming; – Finishing edges; – Edging; – Hole making (for assemblies). – Surface machining; Hole drilling of multimaterial stack Edging with PCD endmill cutter Pictures: Courtesy of Palbit S.A. Pictures: Courtesy of Palbit S.A. Edging with PCD arbor cutter Surface machining Advanced Production Technologies II AMPJ 4 Advanced Production Technologies II AMPJ 5 Challenging of FRPs drilling Challenging of FRPs drilling Low machinability materials Factors that influence drilling performance of composites – High abrasive wear of cutting tools due to the hard and stiff fibres; – Non-homogenous material; – Anisotropic material; Drilling induced damages (high machining costs & parts rejection) – High tool wear; – Delamination; – Fibre-matrix debonding; – Pull-out or uncut fibres; – Spalling; – Chipping; – Fraying; – Fuzzing; – Fibres breaking; – Microcracking; – Matrix burning or cracking; – Resin loss – Inappropriate surface roughness; Source: – … Source: Advanced Production Technologies II AMPJ 6 Advanced Production Technologies II AMPJ 7 Conventional drilling of FRPs Conventional drilling of FRPs Delamination issues common on direct drilling – Peel-up delamination at hole entry; – Push-down delamination at hole exit; – Matrix debonding. Impact of process parameters on delamination – High feed rate -> increase delamination; – Cutting speed -> not consensual impact on delamination; Advanced Production Technologies II AMPJ 8 Advanced Production Technologies II AMPJ 9 Conventional drilling of FRPs Conventional drilling of FRPs Cutting parameters and process optimization: – Usage of back-up plates facilitates the delamination reduction; – Feed variation along the hole depth Source: Youtube, Bad vs. Good quality drilled holes. to achieve delamination free holes. Adapted from. Common optimization techniques: – Taguchi method; – Taguchi method & ANOVA; – Taguchi method, response surface methodology and ANOVA; – Genetic algorithms; – Artificial Neural Networks; – Various combinations of previous. Adapted from. Adapted from. Advanced Production Technologies II AMPJ 10 Advanced Production Technologies II AMPJ 11 Conventional drilling of FRPs Conventional drilling of FRPs Critical thrust force model for conventional delamination: – Based on Linear Elastic Fracture Mechanics Theory (LEFM); – Different analytical models assume different load distribution types: Point load Uniformly distributed loading Point load combined with uniform distributed loading Point load and triangular loading – Material hypothesis; Isotropic Orthotropic – Cracking mode; Mode I Mixed-mode I/II – Delamination shape; Circular Adapted from Adapted from Elliptical Advanced Production Technologies II AMPJ 12 Advanced Production Technologies II AMPJ 13 Conventional drilling of FRPs Conventional drilling of FRPs Tools for FRP drilling Tools for FRP drilling – Minimize delamination reducing axial cutting force component – Conventional twist drill Decreasing point angle of drilling tools; Usually do not meet the requirements for FRPs drilling; Sharp cutting edges (uncoated or thin coatings preferred); Possible to use with proper selection of cutting parameters and cutting Conventional twist drill environments (low feeds rates, adjusted cutting speeds, cooling, back-up plate Picture: Courtesy of Palbit S.A. Multiple cutting edges; support); – Capacity to cut both fibres and matrix Pictures: Courtesy of Palbit S.A. Long operation times. Sharp cutting edges: positive rake and high clearance angles and small – Double point angle twist drill cutting edge radius; Produce lower thrust force and delamination due to their small second point – Increase cutting edge wear resistance angle; Fibres could be very abrasive (e.g. Carbon Fibres) Better wear resistance; Proper choice of materials including coatings: – Brad and Spur or candle stick drill – HSS not recommended; Excellent performance as regards the delamination; – Solid carbides with high Co content and smaller grain sizes; Number of uncut fibres and delamination can be minimised; Double point – Thin diamond coating; angle twist drill Brad & spur – Dagger drill (one-shot drill) drill – Helix angle should be as small as possible to minimize uncut Special double point angle drill with four flutes; fibres at entry and exit edges of holes Small point angle of the secondary edges reams the final surface of the hole; – Minimize mechanical contact during cutting to avoid excessive Low thrust forces leading to low delamination and uncut fibres at hole exit; heating of matrix Burr formation at entry hole; Sharp edges, high clearance angles, low friction coefficient→ diamond Drilling operation longer due to longer tool; coatings Hand tool operations. Adapted from Dagger drill Advanced Production Technologies II AMPJ 14 Advanced Production Technologies II AMPJ 15 Conventional drilling of FRPs Conventional drilling of FRPs Conventional tools for FRP drilling Cutting tools for FRP drilling are continuously being developed; – Step drill New solutions could be found elsewhere: Pilot hole is drilled with the small diameter drill; Final surface is reamed with the wider diameter tool; Step-control scheme; Effect of chisel minimized on delamination; Core drill Step drill Ratio of primary to secondary diameters need to be optimized; – Core or trepanning drill Drills the hole directly to final dimensions by abrasive action; Usage of diamond grains; Thrust force is reduced resulting minimal delamination risks; Difficulty to remove chips. – Special core drill To overcome the limitations of chips removal various types of core drills may be used with inner part similar to previous solutions. Source: Youtube, UNICUT (4:08 min) – Generally cutting parameters and cutting environment must be Special core optimized to each FPR materials specifications. drill Adapted from Advanced Production Technologies II AMPJ 16 Advanced Production Technologies II AMPJ 17 High performance drilling methods High performance drilling methods Vibration assisted drilling: Orbital drilling or helical milling: – Low frequency (20kHz) portable orbital drilling units; Ultrasonic assisted drilling; – May eliminate delamination and thermal induced damage; – Intermittent engagement of the tool; – Stationary tool centre from conventional drilling processes (chisel force) is eliminated; – Reduction of thrust force; – More lateral free space to facilitate the chip removal; – Improvement chips evacuation; Adapted from – High number of cutting parameters that allows higher possibilities for process optimization; – Longer machining times but lower than other – Low productivity; concurrent delamination free approaches. – Challenging the evacuation of the chips generated by the frontal cutting edges. Advanced Production Technologies II AMPJ 18 Advanced Production Technologies II AMPJ 19 High performance drilling methods Cutting fluids Five axis wobble milling: Cutting fluids are common in machining of conventional materials with main – Machining strategy applied into three stages: functions of cooling and lubrication. Drilling of a pilot hole by orbital drilling; When machining FRPs (e.g. CFRPs) cutting fluids are generally avoided. Rotation of the tilted tool about the centre of the hole; – To avoid contamination and deterioration of the machine guiding systems; – Vacuum cleaner used to remove chips from the working area; Machining of the uncut material by helical – Detrimental effects of moisture absorption of FRPs on mechanical properties. milling. – Generates cutting forces directed toward Special cooling processes have been adopted in the literature showing some workpiece centre when machining the outer Source: benefits with respect to dry cutting (e.g. reduce delamination): layers to avoid delamination at entry and exit hole. – Cryogenic machining; – Chilled air. Advanced Production Technologies II AMPJ 20 Advanced Production Technologies II AMPJ 21 Hole quality Criteria Hole quality Criteria Comparison of major inspection techniques for CFRPs Adapted from Advanced Production Technologies II AMPJ 22 Advanced Production Technologies II AMPJ 23 Hole quality Criteria Hole quality Criteria Most used delamination inspection techniques for FRPs Surface roughness/texture measurement techniques Microscopy Digital scanning X-RAY Ultrasonic C-scan Moiré Interferometry Advanced Production Technologies II AMPJ 24 Advanced Production Technologies II AMPJ 25 Safety issues on FRPs machining References Machining of FRPs release small fibers in the form Composite Structures 216, 168–186, 2019. of dust to surrounding environment → harmful to operators health → necessary safety measures: Composites Part A: Applied Science and Manufacturing 125, Art. No. 105552, 2019. – Vacuum aspiration of the fibers dust with adequate Int J Adv Manuf Technol. 105, 2289–2308, 2019. filters; – Clothe protection of operators (e.g. usage of gloves, Composites Part A: Applied Science and Manufacturing 66, 142–154, 2014. dust mask, full goggles); – Control cutting parameters to reduce short fibers dust Sandvik Coromant, Sandvik Coromant User’s Guide. Machining Carbon Fibers formation; Materials, Sweeden, 2020. Low cutting speeds and high feed rates lower the dust formation; Materials 7, 4258-4271, 2014. Compromise between quality and particles emission would be preferable. Journal of Engineering for Industry, Trans ASME 112, 236–239, 1990. Machining of CFRPs release small carbon fibers which are conductive and highly abrasive → negative impact on machine tool. Adapted from Advanced Production Technologies II AMPJ 26 Advanced Production Technologies II AMPJ 27 Outlook Introduction Advanced Production Technologies II Challenges (Tecnologias Avançadas de Produção II – TAP II) Common damages Process parameters Machinability of Multimaterials Cutting tools Multimaterials Drilling Hole drilling strategies Drilling example @ INEGI - CFRP/AA stack Master in Mechanical Engineering – M.EM References 2024-2025 Abílio de Jesus Advanced Production Technologies II AMPJ 1 Advanced Production Technologies II AMPJ 2 Introduction Challenges Hybrid stacks or multi-layered material stacks have been used for the design and production of Drilling of hybrid stacks is a challenging task: high-added-value components: – Differential machinability of the different Adapted from materials; Adhesive film (3M AF163) Holes with tight tolerances are difficult to achieve due – Aeronautics industry, among others; Ti 6-4 to different mechanical properties of materials; CFRP Dimensions variations along the hole depth. – Different materials may be joined such as metals Al 7050 and FRPs; Adapted from – FRPs shows two phases: brittle fibers and ductile matrix -> machining results in intermittent Aluminium fracture of fibers and possible damages; – Common stacks CFRP/AA, CFRP/Ti, AA/CFRP/Ti, …; ARALL (ARamid fibre/ AA metal Laminate) Prepreg – Metals may show high machinability with long GLARE (GLass fibre/AA LaminatE) chips (AA or mild steels) or low machinability (Ti alloys): Metal reinforcements – Drilling operations have significant impact as regards manufacturing costs. Composite Metal chips interference with FRPs; Possible burr formation; Metallic local reinforcement for heavy loaded joints of Build-up edges affect hole quality. composite components (Patented solutions) Advanced Production Technologies II AMPJ 3 Advanced Production Technologies II AMPJ 4 Common damages Process parameters FRP phase (common in FRPs drilling) – Matrix cratering; Effect of Stack – Delamination; Sequence – Fibres pull-out; – Fibres breaking; – Resin loss; Cutting Tool Geometry – Surface cavities; parameters – Thermal modifications; Multi-material stacks drilling FRP/Metal interface – Damage ring; Adapted from – Delamination. Economy of Tool material Metallic material Drilling /coating – Hole size error; – Roundness error; – Burr; Adapted from – Feed marks; Courtesy from. Advanced Production Technologies II AMPJ 5 Advanced Production Technologies II AMPJ 6 Cutting tools Hole drilling strategies Orbital drilling advantages Hole center Tool spin – Theoretically tool should be adapted to FRPs and Metallic stacks; – Reduced thrust force: Burr free in metal; Orbital cutter FRPs are extremely abrasive -> PCD tools are good choice; Delamination free in FRPs. Orbital PCD may react with some metallic alloys -> Carbide drills becomes common – Intermittent cutting: Helical path of Hole revolution the tool center choice with diamond coatings (CVD); Lower cutting temperature; Reduced risk of matrix melting; – Sharp and high hot hardness tools are required; Efficient cooling of tool and workpiece. – Tool diameter smaller than hole diameter: Orbital cutter One tool for different diameter holes; – Tool materials should not react with the multi-materials stack; Reduce tool inventory; Workpiece Tool center Easy chip evacuation; Adapted from Reduced chip damage. – Others: Adjustable cutting parameters in each material; Possibility to repair misaligned holes. Advanced Production Technologies II AMPJ 7 Advanced Production Technologies II AMPJ 8 Hole drilling strategies Hole drilling strategies Direct drilling vs. Orbital drilling Orbital drilling – 2 tools tested (one endmill for steel and one endmill for CFRPs) – Miguel Rodrigues, MSc Thesis, FEUP, 2018 – Miguel Rodrigues, MSc Thesis, FEUP, 2018 – CFRP/DP600 steel – CFRP/DP600 steel CFRP: Entry side CFRP: Entry side Steel: Exit side Steel: Exit side Direct drilling with drill recommended for Orbital drilling with endmill. composites: Appropriate quality holes after Total inability of the tool to perform the work. parameters tuning. Advanced Production Technologies II AMPJ 9 Advanced Production Technologies II AMPJ 10 Hole drilling strategies Hole drilling strategies New tools design and machining strategy New tools design and machining strategy – Marco Freitas, MSc Thesis, FEUP, 2019 – Marco Freitas, MSc Thesis, FEUP, 2019 – CFRP/DP600 steel – CFRP/DP600 steel – Combined tool – Strategy Drill: 5,5mm Stage I - Direct drilling: 5 Drill tip angle:118º Stage II - Orbital drilling: 8 Mill diameter: 6 Stage III - Orbital drilling: 10 Helix angle: 20º Rake angle: 18º 1st stage Cutting speeed 2nd stage 3rd stage Material: WC-Co/TiALN Cutting Four flutes mill Feed rate Orbital speed Orbital Cutting speed feed feed CFRP CFRP CFRP Feed Feed rate rate Courtesy from PALBIT S.A. Steel Steel Steel Advanced Production Technologies II AMPJ 11 Advanced Production Technologies II AMPJ 12 Hole drilling strategies Hole drilling strategies New tools design and machining strategy New tools design and machining strategy – Marco Freitas, MSc Thesis, FEUP, 2019 – Marco Freitas, MSc Thesis, FEUP, 2019 – CFRP/DP600 steel – CFRP/DP600 steel – Results – Results: hole surface and tool damage Hole surface inspection Entrance (CFRP) After 10 holes Tool damage after 5 holes Exit (Steel) After 10 holes Advanced Production Technologies II AMPJ 13 Advanced Production Technologies II AMPJ 14 Hole drilling strategies Hole drilling strategies New tools design and machining strategy Direct drilling and orbital drilling strategy – Marco Freitas, MSc Thesis, FEUP, 2019 – CFRP/DP600 steel – Objective: drill a 12 hole in the CFRP/Ti stack – Results: thurst force evolution – (1) First hole drilled with 8 – entrance in CFRP – Ti works as a back support plate – (2) Enlarge hole to 10 using orbital drilling – entrance in Ti – (3) Enlarge hole to 12 using orbital drilling – entrance in CFRP – Ti works as a back support plate (1) (2) (3) CFRP Ti CFRP Ti CFRP Feed rate Ti Feed rate Feed rate Adpated from Advanced Production Technologies II AMPJ 15 Advanced Production Technologies II AMPJ 16 Hole drilling strategies Hole drilling strategies i) Variable cutting parameters Peck cycle for FRP-metal stack ii) – Cutting speed on a stacked metallic Entry layer i) Start point 10 material, such as titanium, usually needs Mid layer Feed: 0.15 10 to be lower than the FRP; Exit layer ii) Direct drill of the FRP till small distance above 10 interface iii) 2 Feed 0.05 – Variable cutting data can be favourable: Entry layer : CFRP Drilling CFRP composite at high cutting (mm) iii) Begin peck cycle (feed amount adjusted to speed and low feed rate is preferable. Mid layer : Al or Ti metallic material) Drilling Ti alloys requires lower cutting Exit layer : CFRP Adapted from iv) speeds than CFRPs; iv) Return to start point in each peck cycle v) Reduce feed rate on exit v) Adpated from Advanced Production Technologies II AMPJ 17 Advanced Production Technologies II AMPJ 18 Drilling example @ INEGI - CFRP/AA stack References Composite Structures 157, 461-482, 2016. Key Engineering Materials 447-448, 624-633, 2010. Journal of Machining and Forming Technologies 2 (3/4), 1–32, 2010. Composite Structures 135,316-338, 2016. Kennametal, Composites Machining Guide. Composite Structures 200, 679-688, 2018. Sandvik Coromant, Sandvik Coromant User’s Guide. Machining Carbon Fibers Materials, Sweeden, 2020. Miguel Rodrigues, MSc Thesis, FEUP, 2018. Marco Freitas, MSc Thesis, FEUP, 2019. Advanced Production Technologies II AMPJ 19 Advanced Production Technologies II AMPJ 20