Ultrasound Imaging Technology PDF
Document Details
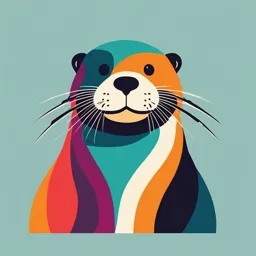
Uploaded by PurposefulNirvana
Tags
Summary
This document provides an overview of ultrasound imaging technology, explaining how it works, the properties of mechanical waves, and the processes involved in making measurements. The document is suitable for understanding the fundamentals of ultrasonic wave propagation.
Full Transcript
Module 2-2 : X-Ray Biomedical Imaging Technology 1 Overview of Technology In this module, we seek to understand the technology generally labeled as Ultrasound Imaging to look inside a patient. To make the GBIF specific to ultrasound imaging in the Figure 1, we need to specify the sensor and processi...
Module 2-2 : X-Ray Biomedical Imaging Technology 1 Overview of Technology In this module, we seek to understand the technology generally labeled as Ultrasound Imaging to look inside a patient. To make the GBIF specific to ultrasound imaging in the Figure 1, we need to specify the sensor and processing elements. An important difference from standard photography, is that the image is not captured directly but rather is constructed from physical measurements; that is, there is no actual image that we are trying to capture. For ultrasound imaging, the frequencies, time-of- flight, reflection, and attenuation of sound waves are used to construct the image. 8 Figure 1: Ultrasonic imaging makes unique uses of sound waves that are reflected and absorbed in the body. 2 Technology Elements 2.1 What is a mechanical wave? Ultrasonic waves are a form of mechanical waves that include audible sound as shown in Figure 2. The bottom row of the figure illustrates the way that different wave frequencies appear in our daily lives. We hear high and low frequency sounds, but there are sounds with frequencies above and below what we can hear. These mechanical waves are different than the electromagnetic waves of light and x-rays, however, the waves share many of the same wave properties. Figure 2: Ultrasonic waves are high-frequency, high-energy sound waves that have special usefulness in helping reveal the internal structure of animals and people, and many objects. 2.2 How are mechanical waves created for ultrasound? Figure 3 below extends the idea of creating a traveling disturbance to include a source that continuously excites the medium. The start of a series of high-then low pressures is shown. The sketch below the illustration shows that the process can be modeled as a wave traveling through the medium. Figure 3: Traveling wave created by an ultrasonic source. 2.2.1 Wave Properties Waves traveling in a medium exhibit a number of properties that make ultrasound imaging possible and some that limit this application. Wave properties of interest: • • • • • Constant velocity in a specific medium Absorption Reflection Doppler Shift Scattering • • • Refraction Diffraction Destructive Interference 2.2.1.1 Constant Velocity in a Medium Waves travel with constant velocity in a medium. The actual velocity depends on the medium as shown in Figure 4. If we could label and follow any specific point on the wave, for example one of the peaks, we would see it move forward at a constant speed. Note that the more compressible (soft) materials like air have low velocities while not compressible materials like water and bone have much higher conduction velocities. If a wave is traveling in air, the speed would be low. If we then we move into a medium like water, the wave would travel much faster. Note however the transition between mediums is complicated and will require further attention. Figure 4: Wave equation and material properties 2.2.1.2 Wave Energy is Absorbed by the Medium As illustrated in Figure 5 absorption refers to the fact that as the wave compresses and decompresses in the material, energy is dissipated as heat. Different materials will have different absorption characteristics. Figure 5: Wave amplitude is reduced as the wave moves through medium. 2.2.1.3 Wave are Reflected and Transmitted at Interface of Two Medium Reflection refers to the condition where a wave reaches the boundary of two materials and the direction of the wave is changed. Think of light reflecting from a mirror as in Figure 6. Figure 6: Reflection by change in medium properties. As illustrated in Figure 8, when a pressure wave arrives at the surface between two materials, part of the wave will be Transmitted (continue in the same direction) and part Reflected. The amount reflected and transmitted is generally characterized by a quantity called the acoustic impedance Z = ρv where ρ is the density of the material and v is the velocity of sound in the material. Figure 7: An incoming (incident) wave is partially reflected and partially transmitted at the interface of two materials, e.g. tissues such as bone and fat. The acoustic impedances of some important biological materials is shown on the right. If the three pressure waves are described as sinusoids, that would have the form: Don’t worry about the complexity of the sinusoids here, just pay attention the amplitudes (the strengths) of the waves represented by AI, AR, and AT. We want to see how the strengths of the sinusoids change at the boundary of the two materials. The relations ship relative to the incoming wave is found to be which shows that the relationships between the acoustic impedances, Z1 and Z2, specifies how much gets reflected and transmitted. Example First assume that a ultrasonic wand is help away from the skin to create the situation where the wave must travel through the air before encountering the skin as shown in Figure 9. The calculations for the reflected wave and the transmitted wave are shown. What we see is that when there is a large difference between the incoming and outgoing sides, most of the wave is reflected and almost none is transmitted. This is not good for ultrasound imaging where we are trying to send waves into the body and measure reflections from organs and tissues. Figure 8: Ultrasound transmitter/sensor held away from skin. Fortunately, the solution is simple: replace the air with a material that has an acoustic impedance closer to the skin as shown in Figure 10. In this case, a gel with the acoustic impedance of Z1= 1.6 was used. Since it exactly matches the acoustic impedance of the skin, all of the incoming wave is transmitted and none is reflected! Figure 9: Ultrasound transmitter/sensor held away from skin by a layer of gel with and acoustic impedance of Z1= 1.6. Note that the transmitted wave may be redirected at the interface. Refraction can also occur at the interface of two materials. It is illustrated in Figure 7 that the wave that continues through the second materials has a path that bends from the incoming wave direction. Figure 10: Wave refraction at the interface of materials. 2.2.1.4 Doppler Shift Doppler Shift relates to sound waves around moving bodies. As illustrated in Figure 8 a source of a sound wave is moving toward an observer, the person hearing the wave, the observer will hear the wave as increased in frequency - it makes a higher pitch than the original source as it approaches. The opposite is true if the source moves away from the observer, the frequency is lowered and sounds lower than the original sound. This is true for all the frequencies in the sound spectrum. Figure 11: Doppler shift from material moving to the left. A special case that combines Reflection and Doppler shift is shown in Figure 9. Here an observer sends out a wave, the frequency at the moving object is higher than the original frequency from the source because of a Doppler shift. If the wave then reflects, it is considered as a new source and a second Doppler shift means the frequency reflected to the observer is even higher. The first calculation shown below in Figure 9 encapsulates these two frequency shifts to show that the received, reflected wave has a higher frequency than the sent wave. The second calculation, obtained by rearranging the first, reveals that if we can measure the two frequencies, then an estimate of the velocity of the object can be made! Figure 12: Calculating Doppler Shift 2.2.1.5 Scattering Scattering illustrated in Figure 10 occurs when waves deviate from their trajectory because of small particles or uneven surfaces. Figure 13: Scattering 2.2.1.6 Diffraction Diffraction occurs when a wave meets a surface with a small whole as shown in Figure 11. The wave is re-radiated from what looks like a point source. Figure 14: Diffraction 2.2.1.7 Interference Destructive interference occurs when two waves reach a point and the magnitudes add to zero. Figure 12 shows how his phenomenon is utilized in noise canceling headphones where a local sound is created to cancel the ambient sound. There can also be constructive interference where the waves sum to create a new wave with increased magnitude. Figure 15: Destructive interference of waves. 2.2.2 Creating and measuring ultrasonic pressure waves The ultrasonic measurements are made in a two-step process using a piezoelectric crystal: 1. Create outbound wave. 2. Measure the return wave. The piezoelectric crystal acts like an actuator to create pressure waves (first row in Figure 13) and then as a sensor to measure incoming pressure waves (second row in Figure 13). The voltage is first applied to create the pressure wave and then removed to listen for the return wave, which then creates a voltage as the device is compressed. The frequency shifts and time of flight are found from the voltage measurements. Figure 16: Piezoelectric crystal is used as both a sensor and an actuator. The piezoelectric actuators/sensors are arrayed in a wand for application to tissue as shown in the left image in Figure 14. Figure 17: Ultrasound wand comprises an array of piezoelectric actuators/sensors 3 Making measurements with ultrasound The basic timing of an ultrasound scan is shown in Figure 15. Two tissues are shown embedded in a media (labeled Fluid). Sequence of measurement events: 1. During the transmission phase, a pulse, a short piece of a wave, is sent out from the sensor. The pulse travels forward until it encounters the boundary of Tissue 1 (labeled B1) a. During the listening phase, reflections from the tissues are measured: b. At B1: i. part of the wave is reflected (R1) and travels back to the sensor. The start of the pulse arrives back at the sensor at twice t1, ie 2 t1. ii. part of the pulse travels forward toward the far boundary of Tissue 1 (labeled B2) c. At B2: i. part of the wave is reflected (R2) and travels back to the sensor. The start of the pulse arrives back at the sensor at 2 t1 +2 t2 . ii. part of the pulse travels forward toward the boundary of Tissue 2 (labeled B3) d. At B3 i. part of the wave is reflected (R3) and travels back to the sensor. The start of the pulse arrives back at the sensor at 2 t1 +2 t2 +2 t3 ii. part of the pulse travels forward toward the boundary of Tissue 2 (labeled B3) e. At B4: i. part of the wave is reflected (R4) and travels back to the sensor. The start of the pulse arrives back at the sensor at 2 t1 +2 t2 .+2 t3 +2 t4 ii. part of the pulse travels forward 2. The remining pulse is not reflected (in this example). Note that the pulses lose amplitude as they travel. The farther a pulse travels the more the amplitude is reduced. Figure 18: Sequence of events during a scan. The array of piezoelectric actuators/sensors creates a beam as shown in the figure. The area around the sensor is called the near field. There is too much noise in this area to make useful measurements. The area at the end of the beam is called the far field. The waves are attenuated too much by this point to provide reliable measurements. In the area in between, four modes of ultrasound are used: • • • • A-Mode – Amplitude mode B-Mode – Brightness mode M-Mode – Motion mode Doppler Mode B-Mode is the view of the relative tissue structure in the observation cone. It is called brightness mode because the reflection at each tissue interface is observed, and the amplitude of the reflection is colored as a shade of gray. If a reflection is strong, then it will be recorded as a value close to “white” and if there is a weak reflection then it will be recorded as a color closer to “black”. Figure 15 illustrates that the B-Mode is a plot of distances from the sensor. Figure 19: Each pixel in the B-Mode image represents the distance of the reflection from the actuator/sensor array, recorded as the time-of-flight measurement and the intensity of the reflection. Lighter, brighter areas represent areas of high density and hence more reflection. A slice of the B-Mode plot, can be recorded as the A-Mode plot as seen in Figure 16. The A-Mode shows the amplitude of each pixel along a line moving away from the actuator/source. It provides a means of making accurate distance measurements. The A-Mode plot may also be obtained directly using a single actuator/sensor. Figure 20: The A-Mode plot shows a plot of the intensity of the reflections along a line moving away from the actuator/source. The M-Mode plot represents the changes in a slice of the B-Mode image as time evolves. It is like a movie of the changes in a slice of the B-Mode plot. Figure 17 illustrates how the frames of the movie are assembled. Figure 21: The M-Mode plot represents sequential stripes of the B-Mode plot recorded as a function of time. The M-Mode plot shows motion in the stripe of the B-Mode plots as the B-Mode plot is more slowly updated. The M-Mode or A-Mode plots are typically plotted with the B-Mode plots as seen in Figure 18. Figure 22: Figure: Sample ultrasound with B-Mode and M-Mode plots. The repetition of features in the M-Mode plots shows how some features are changing live during the live ultrasound. Doppler Mode uses the change in frequencies from moving tissues to record the velocities of these moving tissues. Doppler can be used to show velocities of fluids such as blood flow in Figure 19. Figure 23: Doppler Mode ultrasound. Note that different tissue areas have different velocities and are colored accordingly. 4 Summary We can now put it all together. For each sensor, compare the outgoing and incoming waves to the to measure the change that a small beam (of waves) sees traveling through the subject. Each pixel in the image contains two pieces of information: 1. Brightness shows the intensity of the reflection. A brighter pixel means there was a stronger reflection, and hence the boundary of a denser tissue. 2. Location relative to the sensor array shows the distance from the array determined from timeof- flight measurements. By convention, we typically create images where the dark areas mean less reflection and the bright areas mean more. In Doppler mode the pixel may contain a third piece of information, color coding to show the velocity in that area of the tissue. The image can be modified to convey even more information, for example a specific bone could be colored. Figure 24: Final view of ultrasound measurements of a subject.