Ultrasound Imaging Lecture Notes PDF
Document Details
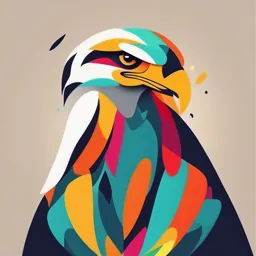
Uploaded by AppreciableDouglasFir
University of Nicosia Medical School
Dr Anastasia Hadjiconstanti,Prof Vered Aharonson
Tags
Summary
These lecture notes cover various aspects of ultrasound imaging, including the production of ultrasound beams and the detection of echoes. The detailed explanations cover different types of receivers, ultrasound fields, and focused transducers that can be used within the medical field. Several factors affecting beamsteering and resolution are also discussed.
Full Transcript
IMAGING WITH ULTRASOUNDS II Dr Anastasia Hadjiconstanti Prof Vered Aharonson Acknowledgements: Dr. Constantinos Zervides LECTURE LOB’S 28. DESCRIBE THE PRODUCTION OF AN ULTRASOUND BEAM. 29. EXPLAIN THE DETECTION OF ECHOES WITH A TRANSDUCER. RECEIVER I The receiver detects and amplifies weak signals...
IMAGING WITH ULTRASOUNDS II Dr Anastasia Hadjiconstanti Prof Vered Aharonson Acknowledgements: Dr. Constantinos Zervides LECTURE LOB’S 28. DESCRIBE THE PRODUCTION OF AN ULTRASOUND BEAM. 29. EXPLAIN THE DETECTION OF ECHOES WITH A TRANSDUCER. RECEIVER I The receiver detects and amplifies weak signals coming from different depths. Different tissue thicknesses attenuate the ultrasound variably. Thus, the difference in echo strength is compensated by time gain compensation (TGC). RECEIVER II The attenuation of sound is proportional to the frequency and is constant for certain tissues. Weaker echoes returning from deeper tissues must be amplified more to produce a uniform appearance of the organ in question. The operator can achieve this using TCG controls which allow: selective amplification from deeper structures and suppression of signals from more superficial structures. TGC IS ONE OF THE MOST IMPORTANT USER CONTROLS AND HAS AN ENORMOUS IMPACT ON THE QUALITY OF THE IMAGE. RECEIVER III Also, the receiver compresses and remaps returning data into allowable dynamic range that can be seen on a gray-scale display. The actual range of reflected signals can vary widely. The receiver can selectively amplify returning signals so that they match the dynamic range of the display. ULTRASOUND FIELDS I The transducer produces ultrasound pulses, making wavefronts that form a 3D beam of ultrasound. The characteristic shape of the beam depends on: the curvature of the transducer, the pressure waves and the acoustic lens which focuses the beam. ULTRASOUND FIELDS II Five terms describe the shape and regions of an ultrasound beam: 1. Focus. 2. Near Zone. 3. Far Zone. 4. Focal Zone. 5. Focal Length or Near Zone Length. ULTRASOUND FIELDS III The sound beam exits the probe and immediately enters the near or Fresnel zone. The beam diameter, or width, is the same as that of a transducer. The sound beam gets progressively narrower in the near zone. The beam continuous to narrow until it reaches its narrowest point, the focus. The focus marks the end of the near zone. The distance from the transducer to the focus is called the focal length, focal depth, or near zone length. The focus also marks the beginning of the far or Fraunhofer zone. ULTRASOUND FIELDS IV At this depth, the beam starts to diverge or widen. At a depth that is twice the focal length, the sound beam is again as wide as the transducer. LOCATION At the transducer At the focus At 2 near zone lengths Deeper than 2 near zone lengths BEAM DIAMETER Beam diameter equals transducer diameter. Beam diameter is half the transducer diameter. Beam diameter equals transducer diameter. Beam diameter is wider than the transducer diameter. FOCUSED TRANSDUCERS I All diagnostic transducers are focused, either by using a curved piezo electric crystal or an acoustic lens. Transducers commonly use an array of piezoelectric elements to transmit and receive sound and echoes respectively. Transmitting and receiving signals, can be individually delayed in time and are termed as phased array. FOCUSED TRANSDUCERS II This arrangement electronically steers and focuses each of a sequence of acoustic pulses through the plane or volume that is imaged, in a process called beamforming. This produces a 2-D or 3-D map of the scattered echoes on the display. The frequency of the ultrasound beam affects the quality of the resulting image to a great degree. Higher frequency ultrasound waves have a longer near field and less divergence in the far field. They permit better resolution of small structures. FACTORS AFFECTING BEAMSTEERING I Changes in transducer diameter The near field is much shorter and diverges considerably greater when the transducer has a small diameter. FACTORS AFFECTING BEAMSTEERING II Changes in transducer frequency Higher frequency transducers have much longer near fields. How come high frequency transducers are used to image superficial structures if they have deeper focus? FACTORS AFFECTING BEAMSTEERING III Changes in transducer frequency The rule of physics is higher frequency sound creates a deeper focus. Transducer manufacturers are aware of this and overcome it by making very small diameter, high frequency crystals. Even with high frequency sound, an extremely small diameter, displaces the focus shallower. This combination creates a clinically useful transducer. The mathematical relationship between diameter, frequency and focal depth is: 𝒅𝒊𝒂𝒎𝒆𝒕𝒆𝒓 (𝒎𝒎)𝟐 ×𝒇𝒓𝒆𝒒𝒖𝒆𝒏𝒄𝒚 (𝑴𝑯𝒛) 𝒇𝒐𝒄𝒂𝒍 𝒅𝒆𝒑𝒕𝒉 𝒎𝒎 = 𝟔 FACTORS AFFECTING BEAMSTEERING IV Mechanical focus Ultrasonic beam emitted by an unfocused and a focused transducer. FACTORS AFFECTING BEAMSTEERING V Electronic focus Diagram demonstrating how a phased array transducer can focus the ultrasonic beam. By appropriate timing of the individual elements, the leading edges of the wavelets can produce a concentrically curved wave front. The resultant ultrasonic beam focuses on a given point from the transducer. FACTORS AFFECTING BEAMSTEERING VI RESOLUTION I Spatial resolution of ultrasound devices determines the ability to separate two adjacent objects. Spatial resolution must be considered in three planes: 1. Depth (Axial) Resolution 2. Lateral Resolution and 3. Elevation Resolution. RESOLUTION II Test Images of phantom: contains circular echo-free regions, shown in (a) and (c), which mimic the presence of cysts of varying sizes. (From left to right, their diameters are 10, 8, 6, 4, 3, and 2 mm.) 3.5 MHz with focal length equal to 8 cm 7.5 MHz with focal length equal to 4 cm RESOLUTION III Two concerns must be balanced in selecting a frequency for ultrasound imaging: ü the depth to which the image will be formed ü the spatial resolution of the image. A lower frequency (longer wavelength) is used to see deep within the abdomen A higher frequencies (shorter wavelengths) finer details can be resolved, but only close to the surface. Consequently, a frequency higher than that used for abdominal imaging (roughly 3 MHz) can be used for breast ultrasound (7.5–10 MHz) because much shallower regions of the body must be imaged in the latter procedure. Introducing the transducer into the body near the tissues to be imaged is another way to take advantage of the higher spatial resolution made possible with higher frequencies. AXIAL RESOLUTION I Axial resolution determines the ability to separate two objects lying along the axis of the beam. Axial resolution tells us what the minimum distance that two structures, positioned front-toback can be apart and still produce two distinct echoes on an US image. Axial resolution is determined by the pulse length and is measured in mm. The pulse length is the product of the wavelength and the number of cycles in the pulse. Since the pulse length determines axial resolution, higher transducer frequencies give better image resolution. AXIAL RESOLUTION II Lower numerical values of axial resolution indicate a shorter pulse. Shorter pulses create more accurate images; therefore, the image quality is better when axial resolution has a lower numerical value. The mathematical description of axial resolution is: 𝒑𝒖𝒍𝒔𝒆 𝒍𝒆𝒏𝒈𝒉𝒕 (𝒎𝒎) 𝒂𝒙𝒊𝒂𝒍 𝒓𝒆𝒔𝒐𝒍𝒖𝒕𝒊𝒐𝒏 𝒎𝒎 = 𝟐 𝒘𝒂𝒗𝒆𝒍𝒆𝒏𝒈𝒉𝒕 𝒎𝒎 ×#𝒄𝒚𝒄𝒍𝒆𝒔 𝒊𝒏 𝒑𝒖𝒍𝒔𝒆 = 𝟐 AXIAL RESOLUTION III AXIAL RESOLUTION IV LATERAL RESOLUTION I Lateral resolution is the ability to distinctly identify two structures that are very close together when they are side by side, or perpendicular, to the sound beam’s main axis. Lateral resolution tells us what the minimum distance that two structures, positioned side by side, can be apart and still produce two distinct echoes on an US image. It is determined by the width of the ultrasound beam and it is measured in mm. Lateral resolution is controlled by focusing the beam, either by an acoustic lens, or by a curved piezoelectric crystal. Lateral resolution is best at the focus where the beam is narrowest and good within the focal zone. LATERAL RESOLUTION II LATERAL RESOLUTION III LATERAL RESOLUTION IV In clinical situations axial resolution is better than lateral because ultrasound pulses are shorter than they are wide. Thus, the numerical value for axial resolution is less than the value for lateral resolution. With regards to resolution, higher frequencies improve both axial and lateral resolution. Axial resolution is improved in the entire image because sorter pulses are associated with higher frequency sound. Lateral resolution is improved in the far field only because higher frequency pulses diverge less in the far field and are narrower than lower frequency beams. COMPARISON OF AXIAL AND LATERAL RESOLUTION AXIAL LATERAL Side by side Perpendicular to beam Beam width IN NEAR FIELD BEST WITH Front to back Parallel to beam Pulse length Shorter pulse Higher frequency and fewest cycles Some at all depths, does not change Shortest pulse IN FAR FIELD BEST WITH Shortest pulse ORIENTATION DETERMINED BY BEST WITH DOES IT CHANGE Narrowest beam Changes with depth, Best at focus Smallest diameter crystal Largest diameter & highest frequency IMAGE ARTEFACTS I IMAGE ARTEFACTS II Artefacts are ultrasound images that do not represent an anatomical structure. They are generated by ultrasound interacting with structures within the body. Shadowing artefacts When ultrasound encounters high-density structures, all of the ultrasound is either absorbed by or reflected away from the surface of the structure. The surface of the structure appears hyperechoic (white). The area deep to bone or gallstones appears anechoic (black) since there is no ultrasound available deep to these structures. This black area is called a shadow. IMAGE ARTEFACTS III Shadowing artefacts can be used for identifying a structure. For example, both gallbladder polyps and gallstones can appear as similar protrusions from the gallbladder wall. However, only gallstones produce a shadow deep to their image because of their high-density. IMAGE ARTEFACTS IV Shadowing artefacts can be a disadvantage when they obstruct the view of a deeper structure. IMAGE ARTEFACTS V Enhancement artefacts Ultrasound passes through low-density fluid filled structures, such as gallbladder or a fluid filled cyst, with little attenuation. It then reaches tissues deep to the fluid filled structures with more energy, making them look “enhanced” and more echogenic (white) than surrounding tissues. An enhancement artefact can be used to differentiate between a fluid filled cyst and a tumour. Both can appear as circular structures within a solid organ. The fluid filled cyst, will produce an enhancement artefact. The tumour will produce less, if any, enhancement artefact. IMAGE ARTEFACTS VI IMAGE ARTEFACTS VII The enhancement artefacts can introduce errors. When measuring the thickness of the gallbladder wall, the posterior wall is “enhanced” and appears thicker than it is. IMAGE ARTEFACTS VIII Mirror image artefact When ultrasound encounters a high reflective curved surface like the diaphragm, part of the reflected ultrasound travels through the liver and returns directly to the probe. However, part of the ultrasound follows a longer indirect trajectory in returning to the probe. The ultrasound system can interpret this longer return time as representing a deeper structure. This can result in a “mirror” image of the liver appearing deeper to the diaphragm. IMAGE ARTEFACTS IX A mirror image of the liver will not usually form in the presence of a pleural effusion. The presence of the mirror image of the liver cephalad to the diaphragm generally excludes a pleural effusion at that point. The disadvantage of this artefact is that the mirror image of the liver can be misinterpreted as representing consolidated lung. IMAGE ARTEFACTS X Reverberation artefact When ultrasound encounters two closely apposed reflective surfaces, like the parietal and visceral pleura, the ultrasound reverberates between the two surfaces. This creates parallel linear artefacts at equidistant intervals on the image. Reverberation artefacts that occur while imaging the lung are called “A lines”. The presence of A lines on an ultrasound image of the chest can be used in the assessment of a patient with dyspnoea. IMAGE ARTEFACTS XI IMAGE ARTEFACTS XII Refraction (edge) artefact When ultrasound encounters the edge of a fluid filled curved structure, the ultrasound waves are deflected from their path. This results in an anechoic (black) artefact that projects from the edge of the fluid filled structure and extends into the far-field. SBA 1 SBA 1-Solution C) small diameter, low frequency. Divergence is pronounced with small diameter, low frequency probes. SBA 2 SBA 2-Solution Longer focal lengths are associated with large diameter, high frequency probes. The probe identified as choice B has the largest diameter and highest frequency and, therefore, the deepest focus 𝒅𝒊𝒂𝒎𝒆𝒕𝒆𝒓 (𝒎𝒎)𝟐 ×𝒇𝒓𝒆𝒒𝒖𝒆𝒏𝒄𝒚 (𝑴𝑯𝒛) 𝒇𝒐𝒄𝒂𝒍 𝒅𝒆𝒑𝒕𝒉 𝒎𝒎 = 𝟔 SBA 3 The pulse length? SBA 3-Solution 𝑃𝑢𝑙𝑠𝑒 𝑙𝑒𝑛𝑔𝑡ℎ = 𝑤𝑎𝑣𝑒𝑙𝑒𝑛𝑔𝑡ℎ 𝑥 # 𝑜𝑓 𝑐𝑦𝑐𝑙𝑒𝑠 𝑖𝑛 𝑝𝑢𝑙𝑠𝑒 = 0.31 𝑚𝑚 𝑥 3 = 𝟎. 𝟗𝟑 𝒎𝒎 SBA 4 SBA 4-Solution 𝑩: Low frequency with more pulses EXERCISE FOR HOME – SBA 1 Which of the following locations is the deepest? A) end of the fresnel Zone B) end of the focal Zone C) end of Fraunhofer Zone D) end of the near Zone EXERCISE FOR HOME – SBA 2 Which of the following probes creates a beam with the less Divergence? A) 4 mm diameter, 4MHz B) 6 mm diameter, 8 MHz C) 6 mm diameter, 2 MHz D) 5 mm diameter, 8 MH EXERCISE FOR HOME –SBA 3 In soft tissue, a 3 cycle, 1 MHz pulse has a pulse length equal to 4.5 mm. What is the axial resolution? SUMMARY I Time Gain Compensation is an amplification technique to increase ultrasound echoes from tissue interfaces that are deeper within the body. Factors affecting beamsteering: 1. Changes in transducer size. 2. Changes in transducer frequency. 3. Mechanical focus. 4. Electronic focus. SUMMARY II Spatial resolution of ultrasound devices determines the ability to separate two adjacent objects. Spatial resolution must be considered in three planes: 1. Depth (Axial) Resolution, 2. Lateral Resolution, and 3. Elevation Resolution. Artefacts are ultrasound images that do not represent an anatomical structure. They are generated by ultrasound interacting with structures within the body. REFERENCES Authors Title Edition Publisher Year ISBN R.K.Hobbie and B.J.Roth Intermediate Physics for Medicine and Biology 5th Edition Springer 2015 9783319126814 M.A. Haidekker Medical Imaging Technology 1st Edition Springer 2013 9781461470724 A.B. Wolbarst, P. Capasso and A.R. Wyant Medical Imaging: Essentials for Physicians 1st Edition Wiley-Blackwell 2013 9780470505700 Sidney K. Edekman Understanding Ultrasound Physics 4th Edition E.S.P. Ultrasound 2012 9780962644450 Suzanne Amador Kane Introduction to Physics in Modern Medicine 2nd Edition CRC Press 2009 9781584889434 V. Gibbs, D. Cole, A. Sassano Ultrasound Physics and Technology. How, Why and When 1st Edition Churchill Livingstone 2009 9780702030413