X-Ray Production Lecture PDF
Document Details
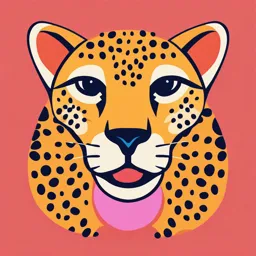
Uploaded by ClearModernism
Prince Sattam Bin Abdulaziz University
Dr. Nahla Atallah
Tags
Summary
This lecture covers X-ray production, highlighting the interactions between projectile electrons and the X-ray tube target, as well as the concepts of characteristic and bremsstrahlung X-rays. It discusses anode heat and efficiency aspects.
Full Transcript
X-ray production Dr. Nahla Atallah TABLE OF CONTENTS After the completion of this chapter, the student should be able to do the following: 1.Discuss the interactions between projectile electrons and the x-ray tube target. 2. Identify characteristic and bremsstrahlung...
X-ray production Dr. Nahla Atallah TABLE OF CONTENTS After the completion of this chapter, the student should be able to do the following: 1.Discuss the interactions between projectile electrons and the x-ray tube target. 2. Identify characteristic and bremsstrahlung x- rays. ELECTRON TARGET INTERACTIONS The x-ray imaging system description emphasizes that its primary function is to accelerate electrons from the cathode to the anode in the x-ray tube. The three principal parts of an x-ray imaging system— the operating console, the x-ray tube, and the high- voltage generator—are designed to provide a large number of electrons with high kinetic energy focused onto a small spot on the anode Stationary objects have no kinetic energy; objects in motion have kinetic energy proportional to their mass and to the square of their velocity. The kinetic energy equation follows. For example, a 1000-kg automobile has four times follows: the kinetic energy of a 250-kg motorcycle travelling at the same speed (Figure 7-1). If the motorcycle were to double its velocity, however, it would have the same kinetic energy as the automobile. Review of Radiation Oncology Physics: A Handbook for Teachers and Students - 1. In determining the magnitude of the kinetic energy of a projectile, velocity is more important than mass. In an x-ray tube the projectile is the electron. All electrons have the same mass; therefore, electron kinetic energy is increased by raising the kVp. As electron kinetic energy is increased, both the intensity (quantity) and the energy (quality) of the x- ray beam are increased. The modern x-ray imaging system is remarkable. It conveys to the x-ray tube target an enormous number of electrons at a precisely controlled kinetic energy. At 100 mA, for example, 6 × 1017 electrons travel from the cathode to the anode of the x-ray tube every second. In an x-ray imaging system operating at 70 kVp, each electron arrives at the target with a maximum kinetic energy of 70 keV. Because there are 1.6 × 10−16 J per keV, this energy is equivalent to the following: O0-0-== When this energy is inserted into the expression for kinetic energy and calculations are performed to determine the velocity of the electrons, the result is a : The distance between the filament and the x- ray tube target is only approximately 1 cm. It is not difficult to imagine the intensity of the accelerating force required to raise the velocity of electrons from zero to half the speed of light in so short a distance. Electrons travelling from cathode to anode constitute the x-ray tube current. When these electrons hit the heavy metal atoms of the x-ray tube target, they transfer their kinetic energy to the target atoms. These interactions occur within a very small depth of penetration into the target. the projectile electrons slow down and finally come nearly to rest, The electron from the cathode interacts with the orbital electrons or the nuclear field of target atoms. These interactions result in the conversion of electron kinetic energy into thermal energy (heat) and electromagnetic energy in the form of infrared radiation (also heat) and x-rays. Anode Heat Most of the kinetic energy of electrons is converted into heat (Figure 7-2). The electrons interact with the outer shell electrons of the target atoms but do not transfer sufficient energy to these outer-shell electrons to ionize them. Rather, the outer-shell electrons are simply raised to an excited, or higher, energy level. Review of Radiation Oncology Physics: A Handbook for Teachers and Students - 1. The outer-shell electrons immediately drop back to their normal energy level with the emission of infrared radiation. The constant excitation and return of outer shell electrons are responsible for most of the heat generated in the anodes of x-ray tubes. Only approximately 1% of electron kinetic energy is used for the production of x-radiation Therefore, sophisticated as it is, the x-ray imaging system is not very efficient. The production of heat in the anode increases directly with increasing x-ray tube current. Doubling the x-ray tube current doubles the heat produced. Heat production also increases directly with increasing kVp, at least in the diagnostic range Although the relationship between varying kVp and varying heat production is approximate, it is sufficiently exact to allow the computation of heat units for use with anode cooling charts. The efficiency of x-ray production is independent of the tube current. The efficiency of x-ray production increases with increasing kVp Characteristic Radiation If the electron interacts with an inner-shell electron of the target atom rather than with an outer-shell electron, characteristic x-rays can be produced. Characteristic x-rays result when the interaction is sufficiently violent to ionize the target atom through total removal of an inner-shell electron. Figure 7-3 illustrates how characteristic x-rays are produced. When the cathode electron ionizes a target atom by removing a K-shell electron, a temporary electron void is produced in the K shell. This is an unnatural state for the target atom, and it is corrected when an outer-shell electron falls into the void in the K shell. The transition of an orbital electron from an outer shell to an inner shell is accompanied by the emission of an x-ray. The x-ray has energy equal to the difference in the binding energies of the orbital electrons involved. By the same procedure, the energy of x-rays resulting from M- to-K, N-to-K, O-to-K, and P-to-K transitions can be calculated. Tungsten, for example, has electrons in shells out to the P shell, and when a K-shell electron is ionized, its position can be filled with electrons from any of the outer shells. All of these x-rays are called K x -rays because they result from electron transitions into the K shell. Similar characteristic x-rays are produced when the target atom is ionized by removal of electrons from shells other than the K shell. Note that Figure 7-3 does not show the production of x- rays resulting from ionization of an L-shell electron. Such a diagram would show the removal of an L-shell electron by the projectile electron. The vacancy in the L shell would be filled by an electron from any of the outer shells. X-rays resulting from electron transitions to the L shell are called L x-rays and have much less energy than K x-rays because the binding energy of an L-shell electron is much lower than that of a K-shell electron. Similarly, M-characteristic x-rays, N-characteristic x-rays, and even O-characteristic x-rays can be produced in a tungsten target. Figure 7-4 illustrates the electron configuration and Table 7-1 summarizes the production of characteristic x-rays in tungsten. Although many characteristic x-rays can be produced, these can be produced only at specific energies, equal to the differences in electron-binding energies for the various electron transitions. Except for K x-rays, all of the characteristic x-rays have very low energy. The L x-rays, with approximately 12 keV of energy, penetrate only a few centimetres into soft tissue. Consequently, they are useless as diagnostic x-rays, as are all the other low-energy characteristic x-rays. The last column in Table 7-1 shows the effective energy for each of the characteristic x-rays of tungsten. Because the electron binding energy for every element is different, the energy of characteristic x-rays produced in the various elements is also different. The effective energy of characteristic x-rays increases with increasing atomic number of the target element. The production of heat and characteristic x- rays involves interactions between the projectile electrons and the electrons of x-ray tube target atoms Bremsstrahlung Radiation A third type of interaction in which the projectile electron can lose its kinetic energy is an interaction with the nuclear field of a target atom. In this type of interaction the kinetic energy of the projectile electron is also converted into electromagnetic energy. A projectile electron that completely avoids the orbital electrons as it passes through a target atom may come sufficiently close to the nucleus of the atom to come under the influence of its electric field (Figure 7-5). Because the electron is negatively charged and the nucleus is positively charged, there is an electrostatic force of attraction The closer the projectile electron gets to the nucleus, the more it is influenced by the electric field of the nucleus. This field is very strong because the nucleus contains many protons and the distance between the nucleus and projectile electron is very small. As the projectile electron passes by the nucleus, it is slowed down and changes its course, leaving with reduced kinetic energy in a different direction. This loss of kinetic energy reappears as an x-ray. These types of x-rays are called bremsstrahlung x-rays. Bremsstrahlung is a German word that means“ slowed-down radiation.” Bremsstrahlung x-rays can be considered radiation that results from the braking of cathode electrons by the nucleus. For example, when an x-ray imaging system is operated at 70 kVp, electrons from the cathode have kinetic energies from zero to 70 keV. An electron with kinetic energy of 70 keV can lose all, none, or any intermediate level of that kinetic energy in a bremsstrahlung interaction. Therefore the bremsstrahlung x-ray produced can have any energy up to 70 keV. This is different from the production of characteristic x-rays, which have very specific energies Figure 7-5 illustrates how one can consider the production of such a wide range of energies through the bremsstrahlung interaction. A low-energy bremsstrahlung x-ray results when the electron is barely influenced by the nucleus A maximumenergy x-ray occurs when the electron loses all its kinetic energy and simply drifts away from the nucleus. Bremsstrahlung x-rays with energies between these two extremes occur more frequently.