Lecture 4 Amino Acids and Proteins PDF
Document Details
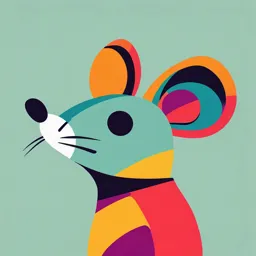
Uploaded by ReadableDivergence
University of Toronto Mississauga
Ichiro Inamoto
Tags
Summary
This document is a lecture on amino acids and proteins, part of a course in introductory cell and molecular biology at the University of Toronto Mississauga. The lecture provides an overview of various amino acids, their properties, and their roles in protein structure and function.
Full Transcript
Lecture 4 Amino Acids and Proteins BIO206 Introductory Cell & Molecular Biology Instructor: Ichiro Inamoto University of Toronto Mississauga 1 Table of contents 4.1 Amino Acids 3 4.2 Pro...
Lecture 4 Amino Acids and Proteins BIO206 Introductory Cell & Molecular Biology Instructor: Ichiro Inamoto University of Toronto Mississauga 1 Table of contents 4.1 Amino Acids 3 4.2 Protein is a polymer of amino acids 20 4.3 Amino acids affect protein folding 27 4.4 Protein structure, primary and secondary 37 4.5 Protein structure, tertiary and quaternary 60 2 Lecture 2.1 Amino Acids 3 Central Dogma of Molecular Biology Storage and inheritance of genetic information Processing genetic information to build proteins Proteins built according to genetic Cellular activity performed by proteins: information. Catalyze metabolic reactions Build cellular structures Send / receive signals 4 and much more Major and minor grooves become binding points for proteins The different ‘shapes’ of nucleotides are exposed on the minor and major grooves. Proteins can access the information without unwinding DNA. Many proteins bind to DNA with high specificity using this information. 5 Protein function is related to its structure Proteins participate in a diverse array of cell functions Enzymes Structural Components Signaling Receptors Motor Proteins Transport Systems Gene Regulation Immune Systems and Antibodies Storage Proteins are a linear polymer of amino acids The amino acid sequence determines how a protein ‘folds’ into its structure 3D structure and chemical reactivity of protein contribute to their function 6 Amino acids are the building blocks of proteins 20 different amino acids are used to build proteins Amino acid composition: central carbon (α carbon) amino group carboxyl group hydrogen side chain (R group) Ionized amino acid at pH 7 4 different groups bonded to the tetrahedral Cα The R group is variable between amino acids. Everything else is consistent. 7 Amino acids side groups determines their properties Amino acid R group varies in Size and shape Hydrophobicity Hydrogen bond forming ability Charge Chemical reactivity hydrophobic hydrophilic / polar acidic basic hydrophobic small larger huge polar, non charged negative charge positive charge sulfur group exposed + + + + + + H2 O− H2 O− + H2 O− H2 O− H2 O− H2 O− H2 O− MBoC6 : Panel 3-1 8 Categorizing amino acids by their polarity and charge Amino acids are grouped in four categories: acid, base, non-charged polar, non-polar Acidic Basic Each amino acid has a three- and a one-letter code. For example, Alanine = Ala = A 9 Amino acids are categorized by their polarity and charge Polarity/charge of an amino acid determines how it interacts with water This has major effect on the influence of that amino acid while the protein folds in aqueous environment (i.e., inside a cell) Polar/charged (hydrophilic) amino acids want to be beside water Non-polar (hydrophobic) amino acids want to be away from water More of this on slides 28 - 30 of this lecture 10 Amino acids are categorized by their polarity and charge Hydrophobicity is not equal for all amino acids Some polar amino acids are more hydrophilic/hydrophobic compared to other polar amino acids For example, the extra methyl group of threonine sidechain makes it slightly more hydrophobic than serine + + H2 O− H2 O− Similarly, non-polar amino acids have varying degrees of how hydrophobic they are 11 Amino acid structure corresponds to their property/function Structure of biological molecules directly correspond to their property and function somewhat large small very large somewhat large polar (hydrophilic) non-polar (hydrophobic) non-polar (hydrophobic) polar (hydrophilic) negatively charged H2+ O− + H2 O− H2+ O− H+2 O− 12 Amino acids with non-polar side-chains Hydrophobic + + + + H2 O− H2 O− H2 O− H2 O− R group is a hydrocarbon isoleucine or other nonpolar, uncharged group + + H2 O− H2 O− + + H2 O− H2 O− 10 in total + + H2 O− H2 O− 13 Amino acids with polar, uncharged side-chains Hydrophilic, but has no charge + + H2 O− H2 O− R group contains an amide or a amide hydrocarbon 5 in total + + + H2 O− H2 O− H2 O− hydroxy 14 Amino acids with polar, charged side-chains Hydrophilic, charged + + H2 O− H2 O− R groups contain acidic / basic groups carboxyl pKa = 3.9 carboxyl such a carboxyl / amino pKa = 4.3 Charged at physiological pH (pH ~ 7.4) + + + H2 O− H2 O− H2 O− 2 acidic amino acids imidazole pKa = 6 3 basic amino acids amino pKa = 10.5 guanidium pKa = 12.5 15 Learning amino acids Note: these slides may not represent actual metabolic pathways used to Start from glycine and modify its structure to derive other amino acids synthesize amino acids in cells Follow how the property of amino acid changes with each modification tiny small very large very large non-polar, hydrophobic non-polar, hydrophobic non-polar, hydrophobic polar, hydrophilic + + + + H2 O− H2 O− H2 O− H2 O− methyl phenyl glycine has a single replace glycine's R group hydroxy hydrogen as its R group hydrogen with a methyl to add a phenyl group to add a hydroxy group to make alanine alanine to make phenylalanine to make glycine is the smallest phenylalanine tyrosine amino acid the amino acid a bit larger phenyl group greatly hydroxy group is hydrophilic, due to its size, having a methyl group is hydrophobic, increases the size which makes tyrosine more glycine in a polypeptide therefore alanine is still hydrophilic than makes that region very hydrophobic phenylalanine is very phenylalanine flexible hydrophobic due to the large hydrophobic surface area 16 Learning amino acids Note: these slides may not represent actual metabolic pathways used to Start from glycine and modify its structure to derive other amino acids synthesize amino acids in cells Follow how the property of amino acid changes with each modification tiny small small medium non-polar, hydrophobic non-polar, hydrophobic polar, hydrophilic polar, hydrophilic + + + + H2 O− H2 O− H2 O− H2 O− methyl hydroxy glycine has a single replace glycine's R group hydrogen as its R group hydrogen with a methyl to add a hydroxy group to add a methyl group to the R make alanine alanine's side chain to make group carbon of serine to glycine is the smallest serine make threonine amino acid the amino acid a bit larger hydroxy group is polar, which increases the size of amino due to its size, having a methyl group is hydrophobic, makes serine much more acid glycine in a polypeptide therefore alanine is still hydrophilic compared to makes that region very hydrophobic alanine makes threonine slightly less flexible hydrophilic compared to serine 17 Learning amino acids Note: these slides may not represent actual metabolic pathways used to Start from glycine and modify its structure to derive other amino acids synthesize amino acids in cells Follow how the property of amino acid changes with each modification tiny small medium medium non-polar, hydrophobic non-polar, hydrophobic polar, hydrophilic negatively charged, hydrophilic + + + + H2 O− H2 O− H2 O− H2 O− amide carboxyl glycine has a single replace glycine's R group hydrogen as its R group hydrogen with a methyl to replace alanine's R group swap the nitrogen of make alanine methyl with an amide to asparagine's amide with an glycine is the smallest make asparagine oxygen to make aspartic acid amino acid the amino acid a bit larger the amide group makes the carboxyl group makes due to its size, having a methyl group is hydrophobic, asparagine more hydrophilic aspartic acid polar and glycine in a polypeptide therefore alanine is still negatively charged under makes that region very hydrophobic physiological condition flexible 18 * Histidine also has an aromatic side-chain (imidazole) but is often categorized as 'polar and charged' since this property Some amino acids has unique features is more important for histidine's function in a protein Some amino acids have unique features due to their R group side-chain connected to backbone nitrogen not an amino acid has aromatic side chains* very large, absorbs UV light at 270 – 280 nm smallest amino acid SH (thiol) group + H2 O− + H2 O− H+ O− H2 + O− H2 + O− + H2 O− 2 Bond angle between backbone nitrogen and alpha carbon locked due to The ‘side-chain’ is only a the sidechain Bulkiest amino acid hydrogen Takes away a lot of Double-ring structure Gives protein a lot of structural flexibility in a structural flexibility protein 19 Lecture 2.2 Protein is a polymer of amino acids 20 Protein function is related to its structure 3D structure and chemical reactivity of protein contribute to their function Proteins are a linear polymer of amino acids The amino acid sequence determines how a protein ‘folds’ into its structure 21 Amino acids are linked to one-another via peptide bonds Peptide bond Peptide group Two amino acids gets condensed into one molecule while the peptide bond forms Notice the NCC-NCC pattern of the peptide backbone 22 Amino acids and amino acid residues These individual subunits before polymerization are called amino acids. This slide shows four amino acids, Met, Asp, Leu, Tyr N-C-C patterns in amino acids become the peptide backbone upon polymerization The central carbons (α-carbon) are linked to the R-groups 23 Amino acids and amino acid residues Technically, amino acids are no longer 'amino acids' once they are polymerized, since the chemical composition of their amino and carboxyl end has changed Individual units of 'amino acids' can still be identified within the polymerized peptide due to their R group side chains These 'remainders' of amino acids within a peptide are called amino acid residues The peptide in this slide contains four amino acid residues, Met, Asp, Leu and Try 24 Amino acids and amino acid residues Polypeptide: any chain of amino acids linked to one another via peptide bonds Definition of 'Protein' is a bit more vague Protein: usually a longer peptide which has folded into a unique 3D shape and with a defined function side chains Typical protein would be about 100 - 1000 amino acids long The polymer in this slide would be considered a peptide 25 Protein Structure | Building Blocks Practice recognizing the NCC repeats to identify the peptide backbone Identify R groups attached to each central carbon Recognize the N-terminus and the C-terminus of each peptide / protein N C 26 Lecture 2.3 Amino acids affect protein folding 27 Proteins fold into a stable conformation to be functional Conformation: protein's final folded structure Protein's conformation may change by interacting with other molecules Also, some proteins need help from other proteins to fold properly Amino acid sequence determines protein folding Conformation must satisfy the 'needs' of protein's amino acid residues Hide hydrophobic residues from water, etc. 28 Proteins fold into a stable conformation to be functional Basic strategy to stably fold proteins in an aqueous environment: 1. Cluster hydrophobic (non- polar) amino acids together and put them away from water 2. Expose hydrophilic (polar) amino acids on the surface so that they are beside water 29 Amino acids influence protein folding: non-covalent interactions For a cytoplasmic protein (i.e., aqueous environment): Hydrophilic amino acids prefers being on the surface; they want to be beside water Hydrophobic amino acids prefers clustering in the center of the protein, away from water For a membrane protein (i.e., it interacts with hydrophobic core of lipid bilayer): Protein's surface will have an area of hydrophobic amino acid residues exposed which interacts with the hydrophobic core of lipid bilayer Exposed hydrophobic surfaces can also facilitate multiple proteins to attach to one another 30 Amino acids influence protein folding: non-covalent interactions Various non-covalent interactions attract different regions within polypeptide 31 Protein folds by its peptide backbone Flexibility within peptide backbone rigid peptide bonds varies: Bonds before and after the central carbon (N – Cɑ bond and Cɑ – C bond) are flexible Peptide bond is rigid How the backbone can fold is restricted by this flexibility flexible N – Cɑ and Cɑ – C bonds 32 Amino acids influence protein folding: Proline and its unusual R-group Proline further restricts backbone flexibility by making the N – Cɑ bond no proline not flexible very rigid flexible flexible R | N - C - C - N - C - C - N - C - C - N | | R R + H2 O− with proline not flexible not flexible flexible R | N - C - C - N - C - C - N - C - C - N | R 33 Amino acids influence protein folding: Cysteine and disulfide bonds Cystines can form covalent, disulfide bonds to hold polypeptides together + H2 O− 34 * Histidine also has an aromatic side-chain (imidazole) but is often categorized as 'polar and charged' since this property Review of amino acids with unique features is more important for histidine's function in a protein Some of these unique features have substantial effect on protein folding side-chain connected to backbone nitrogen not an amino acid has aromatic side chains* very large, absorbs UV light at 270 – 280 nm smallest amino acid SH (thiol) group + H2 O− + H2 O− H+ O− H2 + O− H2 + O− + H2 O− 2 Bond angle between backbone nitrogen and alpha carbon locked due to The ‘side-chain’ is only a the sidechain Bulkiest amino acid hydrogen Takes away a lot of Double-ring structure Gives protein a lot of structural flexibility in a structural flexibility protein 35 Representing protein structure 1) 2) Protein structures can be represented in many different ways, some more useful than others 1. Backbone N-C-C represented as lines 3) 4) 2. Ribbon diagram, showing backbone with representations of secondary structures α helix: curled ribbons β sheet: ribbon with arrows 3. All atoms shown (except for hydrogens) 4. Space filling model, showing sizes of all atoms These are the same protein shown in four different ways. 36 Lecture 2.4 Protein structure, primary and secondary 37 Protein structures are categorized by their ‘level of complexity’ Primary structure (1˚) Linear amino acid sequence of the protein Secondary structure (2˚) Small segments of peptide backbone form simple 3D structures such as a-helix and b-sheet Tertiary structure (3˚) The entire peptide backbone (individually folded in their 2˚ structures) folds into the full 3D structure of the protein Quaternary structure (4˚) In many cases, more than one folded protein combine into a larger structure 38 Primary structure (1˚) Primary structure (1˚) Linear amino acid sequence of the protein https://www.ncbi.nlm.nih.gov/protein/NP_062164.1 carbonic anhydrase 2 [Rattus norvegicus] LOCUS NP_062164 260 aa linear ROD 16-FEB-2019 DEFINITION carbonic anhydrase 2 [Rattus norvegicus]. ACCESSION NP_062164 SOURCE Rattus norvegicus (Norway rat) 1 mshhwgysks ngpenwhkef piangdrqsp vdidtgtaqh dpslqpllic ydkvasksiv 61 nnghsfnvef ddsqdfavlk egplsgsyrl iqfhfhwgss dgqgsehtvn kkkyaaelhl 121 vhwntkygdf gkavqhpdgl avlgiflkig pasqglqkit ealhsiktkg kraafanfdp 181 csllpgnldy wtypgslttp pllecvtwiv lkepitvsse qmshfrklnf nsegeaeelm 241 vdnwrpaqpl knrkikasfk 39 Primary structure (1˚) Primary structure (1˚) (also called primary sequence) Linear amino acid sequence of the protein Primary sequence determines how the protein folds into its 3D shape Primary sequences of wild-type proteins and its mutants can be compared to spot mutations 40 Multiple Sequence Alignment Align primary sequences of proteins for comparison In example below, mutant_1 has its 4th valine substituted with leucine, compared to wild-type ('V4L' mutation) position 5 10 15 | | | valine and leucine are both wild-type AIFVKIQPASPQLHR hydrophobic and similarly sized mutant_1 AIFLKIQPASPQLHR this mutation may not have huge mutant_2 AIFEKIQPASPQLHR impact on overall protein activity *** *********** 41 Multiple Sequence Alignment Align primary sequences of proteins for comparison In example below, mutant_2 has its 4th valine substituted with glutamic acid, compared to wild-type ('V4E' mutation) position 5 10 15 | | | valine and glutamic acid are wild-type AIFVKIQPASPQLHR very different (hydrophobic vs. mutant_1 AIFLKIQPASPQLHR negatively charged) mutant_2 AIFEKIQPASPQLHR this mutation may have huge *** *********** impact on overall protein activity 42 Secondary structure (2˚) Secondary structure (2˚) Small segments of peptide backbone form simple 3D structures Major secondary structures a-helix b-sheet other structures turns, nonrepeating (loops and coils), 310 , π, poly-Pro II helices many proteins are intrinsically distorted, but these are still NOT RANDOM 43 Secondary structure (2˚) Secondary structures are created and stabilized by intramolecular hydrogen bonds a-helix b-sheet C N anti-parallel C N right-handed helix parallel 44 α helix α helix is a right hand helix each amino acid residue corresponds to a 100° turn in the helix or 3.6 residues per turn The R-groups point outward Ribbon traces the N-C-C backbone 45 α helix Amino terminus (N) N C N C C N C C α helix is a right hand N helix C C C N C each amino acid N residue corresponds CC N to a 100° turn in the C helix or 3.6 residues C N per turn C C N C C C Carboxy terminus (C) The R-groups point outward Ribbon traces the N-C-C backbone 46 α helix backbone structure C-terminus C⍺ 9 Hydrogen bonds between the ‘NCC’ C' 9 peptide backbone hold the α helix together Every backbone C=O group forms hydrogen bond with the N-H group of the amino acid residue located 4 residues downstream of the peptide hydrogen bond N N C’ 1 1 C⍺ 1 1 C=O group of amino acid N-terminus N-H group of residue 1 amino acid central carbon of reside 1 47 amino acid residue 1 α helix backbone structure C-terminus C⍺ 9 Every backbone C=O group forms hydrogen C' 9 bond with the N-H group of the amino acid residue located 4 residues downstream of the peptide For example, C=O group of aa1 forms hydrogen bonds with N-H group of aa5 C=O group of aa2 forms hydrogen bonds with N-H group of aa6 N Pattern continues throughout the α helix 1 N-terminus C=O group of aan forms hydrogen bonds with N-H group of aan+4 48 C-terminus O II C⍺ 9 – N9 – Cα9 – C’9 – C' 9 amino acid residue 9 I H : : O II amino acid residue 5 – N5 – Cα5 – C’5 – I H : : O II amino acid residue 1 – N1 – Cα1 – C’1 – N I 1 H N-terminus C=O group of aan forms hydrogen bonds with N-H group of aan+4 49 Positioning amino acids in the α helix In the primary sequence, polar and non-polar amino acid may look like they are distributed randomly Primary sequence But is this completely random? 3 4 7 10 11 14 18 Polar amino acids shown in green numbers 50 Positioning amino acids in the α helix Because of the helical structure, amino acids in certain locations of the primary sequence ends up on the same side once α helix forms Primary sequence amphipathic a helix 3 4 7 10 11 14 18 Frequently, hydrophobic / hydrophilic amino acids are grouped on the same side One side of helix will be hydrophobic, and the other side hydrophilic The hydrophobic side can be used to interact with other hydrophobic molecules, each amino acid while the hydrophilic side residue corresponds to can face water etc. secondary structure, a 100° turn in the helix51 viewed top down β sheet Adjacent chains held together by hydrogen bonds between backbone residues antiparallel β sheet C Different configurations of β sheets Antiparallel – backbone run in opposite direction N Parallel – backbone run in same direction Mixed - combination of parallel and antiparallel parallel β sheet C N 52 β sheet Cα - R N C’ Rippled or “pleated” appearance when viewed from the edge R - Cα N side chains alternate pointing up and down C’ Cα - R right-handed twist viewed along strands N C’ R - Cα 5-10 amino acids per strand N 2-15 strands per sheet (avg. ~6) C’ cylinder or barrel shape (b barrels) Cα - R N 53 β sheet hydrogen bond pattern This diagram shows three polypeptide backbone in an anti-parallel β sheet N C C N C C N N C N C C The direction of each sheet can be determined by the relative positions of N, Cα and C’ 54 β sheet hydrogen bond pattern N-H and C=O of neighboring amino acids in two strands form hydrogen bonds – C’ – Cα – N – II I C C N O H : : N C H O I II C – N – Cα – C’ – 55 β sheet hydrogen bond pattern N-H and C=O of neighboring amino acids in two strands form hydrogen bonds No hydrogen bonds occur in the adjacent pair of amino acids – C’ – Cα – N – II I C C N O H N C H O I II C – N – Cα – C’ – 56 β sheet hydrogen bond pattern N-H and C=O of neighboring amino acids in two strands form hydrogen bonds No hydrogen bonds occur in the adjacent pair of amino acids – C’ – Cα – N – Hydrogen bonds occur II I C C N in the third pair of O H amino acids : : N C H O Hydrogen bonding I II C occurs one every two – N – Cα – C’ – amino acid pairs 57 Extremely simplified schematic diagram of an anti-parallel β sheet Only shows: Nitrogens Cargons Hydrogen bonds 58 Amino acids in green box have hydrogen bonds in between. Adjacent pairs do not have h-bonds. Secondary structure is determined by the primary structure Primary structure (sequence) determines the secondary structure Computer programs can be used to predict secondary structures from the primary sequence Secondary structure (2˚) analysis of the Rat CA protein Primary Sequence 2˚ structure prediction Primary Sequence 2˚ structure prediction Primary Sequence 2˚ structure prediction Primary Sequence 2˚ structure prediction H = ⍺-helix E = β-sheet 59 Ashok Kumar, T. (2013). CFSSP: Chou and Fasman Secondary Structure Prediction server. WIDE SPECTRUM: Research Journal. 1(9):15-19. Lecture 2.5 Protein structure, tertiary and quaternary 60 Tertiary structure (3˚) 3-Dimensional arrangement of the polypeptide 2° structures fold on to each other, forming the final 3-D shape of the protein single-domain proteins Proteins shown here all have a single discreet region (domain) which can fold and function independently Three examples of single domain proteins: A. composed mostly of α-helix B. mixed α-helix and β-sheets C. composed mostly of β-sheets 61 Tertiary structure (3˚) Many proteins has multiple domains Multi-domain protein has more than one domains which are connected to one another by the peptide backbone Typically, each domain in a multi-domain protein can fold independently and has a discreet function Individual functions of all domains in the multi-domain combines to give the protein its overall function N domain 1 domain 2 domain 3 C 62 protein represented in linear form domain 1 Tertiary structure (3˚) domain 3 Many proteins has multiple domains Multi-domain protein has more than one domains which are connected to one another by the peptide backbone Typically, each domain in a multi-domain protein can fold independently and has a discreet function Individual functions of all domains in the multi-domain combines to give the protein its overall function domain 2 N domain 1 domain 2 domain 3 C 63 protein represented in linear form DNA binding DNA cleavage domain domain Tertiary structure (3˚) Individual domains of a multi-domain Two-domain protein can get separated by genetic protein which engineering, genetic recombination attach to and during evolution, etc. cleave DNA Separated domains can retain their folding and function Likewise, independent protein domains can get combined (fused) together to give rise to a new multi-domain protein Nature has used domain shuffling to mix and match already-existing protein domains to give rise to new proteins over the course of evolution DNA binding protein DNA cleaving protein 64 Some domains are found in many proteins – Domain shuffling Evolution does not necessarily “re-invent the wheel” Complex organisms, on average, use more domains It recombines previously selected domains per protein of similar function than lower organisms65 Quaternary structure (4˚) Multiple protein associates together to form a larger, more complex structure Hemoglobin: A heterotetramer composed of 2 identical α subunits and 2 identical β subunits. Quaternary structure (4˚) A Monomer of protein A X-mer Monomer – 1 polypeptide chain Dimer – 2 polypeptide chains A A Homodimer of two protein A Trimer – 3 Tetramer – 4 Pentamer – 5 Hexamer – 6 Heterodimer of protein A Octamer - 8 B A and protein B Homo- or heteromer B A Each polypeptide in an X-mer Heterotetramer of protein A and protein B (especially larger ones) called B A ‘subunits’ Proteins assemble into complex structures 68