Lecture 2: Interaction of X-rays with Matter PDF
Document Details
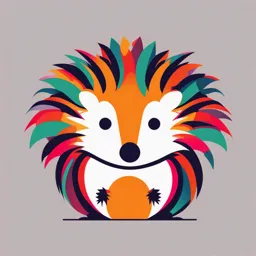
Uploaded by PoisedCosine
University of the Philippines Los Baños
IR Colambo
Tags
Summary
This document is a lecture on the interaction of X-rays with matter. It covers the history of X-rays, including key discoveries and Nobel Prizes, and different interactions such as Compton scattering and photoabsorption. It also explains synchrotron radiation and various x-ray spectroscopy methods, such as photoemission spectroscopy.
Full Transcript
Lecture 2: Interaction of X-rays with Matter APHY 191 IR Colambo X-rays X-rays are produced in an evacuated glass tube when high speed electrons decelerate quickly. APHY 191...
Lecture 2: Interaction of X-rays with Matter APHY 191 IR Colambo X-rays X-rays are produced in an evacuated glass tube when high speed electrons decelerate quickly. APHY 191 IR Colambo History of X-rays 8 November 1895, Röntgen first detected x-rays Röntgen would receive the first ever Nobel Prize in Physics in 1901 APHY 191 IR Colambo History of X-rays In 1909, characteristic x-radiation was discovered by the British physicists Barkla and Sadler Received a Nobel Prize in 1917 APHY 191 IR Colambo History of X-rays Henry Moseley at the Trinity– Balliol laboratory and his published plot of x-ray emission frequencies versus atomic number. APHY 191 IR Colambo History of X-rays Max von Laue and the first x- ray diffraction pattern of copper sulfate. awarded the Nobel Prize in Physics in 1914 for this discovery APHY 191 IR Colambo History of X-rays W. H. Bragg, his son W. L. Bragg, and the diffraction of x-rays by crystals. awarded the Nobel Prize in Physics only 1915 ‘for their services in the analysis of crystal structure by means of x-rays’. APHY 191 IR Colambo History of X-rays The discovery of the structure of DNA. Watson and Crick and their model of DNA. The determination of its structure was much facilitated by the beautiful x-ray diffraction images of crystals of DNA salts recorded by Rosalind Franklin APHY 191 IR Colambo History of X-rays The structures of myoglobin (left) and haemoglobin (right), determined by Kendrew and Perutz using x-ray diffraction. The Nobel Prize in Chemistry 1962 APHY 191 IR Colambo History of X-rays Dorothy Hodgkin’s molecular model of penicillin, based on three orthogonal projections of its electron-density distribution, calculated. The Nobel Prize in Chemistry 1964 APHY 191 IR Colambo History of Synchrotron Radiation First observation of synchrotron radiation came on 24 April 1947 at the General Electric Research Laboratory in Schenectady, New York APHY 191 IR Colambo History of Synchrotron Radiation Photon energies produced by modern synchrotrons are three to four orders of magnitude smaller than the electrons’ relativistic kinetic energy APHY 191 IR Colambo Synchrotron radiation: a focal point for multidisciplinary research Publications which explicitly mention ‘synchrotron’ or ‘synchrotron radiation’ Synchrotron users by discipline. Estimated by the author from user data between 2013 and 2016 at the SLS, SSRL, APS, and ESRF. APHY 191 IR Colambo Interaction of X-rays with Matter Analyze Information -electrons -chemistry -photon -geometry -ions -electronic structure -magnetic properties APHY 191 IR Colambo The Electromagnetic Spectrum APHY 191 IR Colambo The Electromagnetic Spectrum where, h = 6.626 × 10−34 J s 𝜈 is the frequency of the radiation in Hz c = 2.9979 × 108 m/s is the speed of light in vacuum 𝜆 is the wavelength of light in vacuum, given in meters. APHY 191 IR Colambo The Electromagnetic Spectrum Cross-section - it is a measure of the probability that photons interact with matter by a particular process where, ΛP is the attenuation length due to process P (that is, the length after which the beam intensity is reduced to 1∕e) Ni is the atomic number-density (atoms/unit volume) of the atom causing process P APHY 191 IR Colambo Cross section APHY 191 IR Colambo Compton Scattering kinetic energy of the photon may be transferred to the electron, resulting in the scattered photon having a lower energy Energy los in Compton scattering: Compton scattering length: hv > mec2 APHY 191 IR Colambo Compton Scattering Compton scattering Incident photon is beyond 30 keV APHY 191 IR Colambo Thomson Scattering Thomson scattering is the elastic scattering of electromagnetic radiation by a free charged particle - the particle's kinetic energy and photon frequency do not change as a result of the scattering. Thomson scattering length: Elastic scattering from a single electron has a total cross-section: APHY 191 IR Colambo Photoabsorption (X-ray absorption process) Photons incident on an atom can be absorbed by that atom to induce excitation of a bound electron either to an unoccupied bound state, or to produce an unbound photoelectron APHY 191 IR Colambo Absorption coefficient describes the exponential drop in intensity of an incident beam passing through a medium Depends on: types of atoms constituting the medium how they are distributed nature of their bonding Beer–Lambert law for linear absorption magnetism light polarization wavelength APHY 191 IR Colambo Absorption coefficient beryllium (red curve) silicon (yellow) lead (blue) APHY 191 IR Colambo X-ray absorption process Conservation of energy Pauli’s exclusion principle requires that the final quantum state Ef was unoccupied before absorption APHY 191 IR Colambo Energy-level Schemes of Atoms, Molecules, and Solids energy of a level n in a hydrogen atom RH = Rydberg constant = 13.6 eV n = principal quantum number APHY 191 IR Colambo Energy-level Schemes of Atoms, Molecules, and Solids Energy of a photon resonant to a transition from 1s to n excited state APHY 191 IR Colambo Energy-level Schemes of Atoms, Molecules, and Solids APHY 191 IR Colambo Energy-level Schemes of Atoms, Molecules, and Solids Large number of such electrons (of the order of Avogadro’s number, 6 × 1023) APHY 191 IR Colambo Absorption Edges and Nomenclature APHY 191 IR Colambo Absorption Features Core-level absorption edges APHY 191 IR Colambo X-ray Fluorescence characteristic x-ray lines result from the transition of an outer-shell electron relaxing to the hole left behind by the ejection of the photoelectron from the atom APHY 191 IR Colambo Auger Emission First, a core-electron is ejected from an atom by absorption of a photon. Next, the system relaxes by an outer electron falling into the core-level, releasing an energy |En − Ec|. In the Auger process, this energy is channelled into the ejection of a second, or Auger, electron. APHY 191 IR Colambo Nomenclature and Emission Energies Siegbahn nomenclature of x-ray fluorescence lines APHY 191 IR Colambo Photoemission Fermi's Golden Rule: APHY 191 IR Colambo Work function the minimum photon energy required to produce direct photoelectrons lies around 5 eV APHY 191 IR Colambo Inelastic Mean Free Path Universal Curve IMFP Λe of an electron in condensed matter is the distance it will travel on average before being inelastically scattered depends strongly on the electron’s kinetic energy APHY 191 IR Colambo Photoelectron Spectroscopy Ekin= h - ϕ - BE 1. X-ray Photoelectron Spectroscopy (XPS) – uses X-ray with photon energies between 100 eV– 10 keV to excite core level electrons 2. Ultraviolet Photoelectron Spectroscopy (UPS) – uses photon in the UV spectral range photon energies between 5–100 eV to excite valence electrons. APHY 191 IR Colambo Chemical Shifts in XPS Doublet Peaks in XPS Doublet Peaks in XPS Degeneracy of the Two States FIRST EXAM: 10 October 2024, 5:30-7 PM Journal Presentation -Spectroscopy Groups – November 14 -Scattering Groups - November 19 -Imaging Groups - November 21 Prepare a 20- to 25-min presentation (all members should do the presentation) Research Project (Beamtime proposal) -Statement of the Problem -Rationale (Why the problem needs to be investigated using SR) -Objectives -Methodology (summary only) APHY 191 IR Colambo The Measurement Process Synchrotron radiation Angle-resolved photoelectron spectroscopy (ARPES) 2D valence bandstructure of ordered surfaces Ei(k//) // // APHY 191 IR Colambo APHY 191 IR Colambo Angle-Resolved Photoelectron Spectroscopy (ARPES) Fermi Surface -2 -1 0 1 2 2 2 1 1 k||y(Å ) -1 0 0 -1 -1 -2 -2 -2 -1 0 1 2 -1 k||y(Å ) APHY 191 IR Colambo Why go NANO? Nanotechnology: control and restructure, atomic and molecular levels(1–100 nm). => new properties and functions. 49 Microscopy Cheng et al. Carbon Optical Microscope TEM 49, 2917 (2011) Antares Graphene Topographic SEM information! STM Hupalo et al. Surf. Sci. 493, 526 (2001) Lefebvre et al. PRL 90, 217401 (2003) Razado, Masters thesis (2001) 50 First nanoARPES available at Synchrotron SOLEIL Normal synchrotron beam size: 90-100 μm Focused beam size: 100 nm nanoSPOT and nanoPOSITIONING Sample Interferometer Scienta controllers Analyzer OSA ZP Optical microscope Resolution around 100 nm Focused beam 3D Piezo-scanner Thermal stability Mechanical stabilty Precise motion + High SPATIAL resolution!!! Closed-loop travel 300 x 300 x 300 µm 52 Closed-loop resolution 1 nm Highly oriented pyrolitic graphite STEP SIZE: 300 nm © ◊ STEP SIZE: 100 nm 54 K point HOPG graphite 96 94 Kpoint ) 92 V e ( y 90 g r 2D image - STEP SIZE: 100 nm e n E it 88 c e in K 86 84 82 1.0 1.5 2.0 2.5 kp (A-1) STEP SIZE: 300 nm 95 ) V e ( y 90 g r e n e c ti e n i K 85 Scienta R4000 as detector Intensity at the Fermi level 80 1.0 1.5 2.0 2.5 kpara M point 55 HIGHLY ORIENTED PYROLITIC GRAPHITE (HOPG): II Nano-ARPES image of the K point intensity: Direct electronic information about the orientation of the granular structure of the HOPG Nano-ARPES band structure INSIDE the grain (A) and OUTSIDE de grain (B) 56 APHY 191 IR Colambo HIGHILY ORIENTED PYROLITIC GRAPHITE (HOPG): III © ◊ Fermi surface Multigrain of one grain Fermi surface 57 APHY 191 IR Colambo Individual graphene flakes on Si02 by exfoliation 58 Nano-XPS Photoemission Detector hv= 350 eV Graphene 1ML: C 1s C 1s 1s at 63.3 eV ke:63.3 eV Outside the sample 70 um x 70 um 59 Searching our microsample ! Single layer of exfoliated graphene 60 Graphene obtained by liquid synthesis and its oxide layer h=550 eV Region A Graphene ZP=1500 um ; A r=100 nm Oxide C1s: 317.5 eV R400 Scienta B detector C Region B Region C Interface Graphene C1s: 317.5 eV C1s: 318.2 eV and 318. 2 eV 61 Searching our micro_sample ! Single layer of exfoliated graphene 62 Mother of all graphitic forms Graphite Graphene 2D 0D 1D 3D Graphene Fullerenes Nanotubes Graphite Graphene is a 2D building material for carbon materials of all other dimensionalities. It can be wrapped up into 0D buckyballs, rolled into 1D nanotubes or stacked into 3D graphite. APHY 191 IR Colambo Graphene properties APHY 191 IR Colambo graphene band-structure tight-binding calculation Wallace Phys. Rev. 719, 622 (1947) Dirac cone: - linear dispersion - K and K’ Dirac point - electron-hole symmetry Wilson Phys. Today 59, 21 (2006) Graphene Lattice 1st Brillouin zone Mallet Graphene Int. School, Cargese (2010) real space: reciprocal space: - interatomic spacing: 1.42 Å - || = 1.70 Å-1 - pattern: 2 inequivalent C atoms - || = 1.45 Å-1 - |ai| = 2.46 Å - density: 3.8 1015 C/cm2 - identical to one graphite layer 66 APHY 191 IR Colambo existing commercial substrates (up to 4 ’’) polytypes Si face (0001) C face (0001) Starke et al. Surf. Rev. Lett. 6, 1129 (1999) 3C 4H 6H lattice 3.27 3.33 4.35 (Å) 10.1 15.1 gap 2.39 3.27 3.02 (eV) Lauffer et al. Phys. Rev. B 77, 155426 (2008) SiC density: 1.2 1015 C/cm2 / SiC bilayer ~3 SiC bilayers / graphene density: 3.8 1015 C/cm2 graphene layer Molecular Beam Epitaxy (MBE) Epitaxial growth of thin films with precise control of the thickness down to a single layer of atoms. => unidirectional flow of molecules or atoms => Epitaxy – ordered growth of one crystalline layer Optimum growth conditions (growth temperature, upon another (substrate), thus crystallographic growth rate) direction is always related to the substrate -surface difussion -chemisorption or nucleation -lattice incorpotation 69 APHY 191 IR Colambo UHV MBE System to grow graphene 70 APHY 191 IR Colambo Molecular Beam Epitaxy UHV MBE system (IEMN, France) APHY 191 IR Colambo Graphene Field-Effect Transistor APHY 191 IR Colambo X-ray photoelectron spectroscopy (XPS) E K = h - E B - Element-specific C 1s C-C C-Si Overview Graphene on silicon carbide (SiC) Core level Chemical shifts Elemental analysis 73 APHY 191 IR Colambo APHY 191 IR Colambo Graphene Stacking AB AA Rotated/Twisted layers θ=5° θ=10° APHY 191 IR Colambo Graphene on the two faces of SiC 0.0 1 ML Si-face -0.5 Energy AB -1.0 2 ML -1.5 KE=106,05 eV Mallet et. al., PRB 76, 041403(R) (2007) -0.4 -0.2 0.0 0.2 0.4 ky Rotational disorder 3-5 MLs C-face Varchon et. al., PRB 77, 165415 (2008) Graphene on the C-face of SiC: STM (a) (b) θ=1.5° (c) θ=17.9° I II 20x20 nm2 20x20 nm2 20x20 nm2 (d) (e) D Top layer Bottom layer 3x3 nm2 θ=17.9° θ=17.9° Previous studies: Graphene on the C-face of SiC Conventional ARPES (beam size=100 microns) N azimuth W azimuth 1.6 ML FWHM 0.060 Å-1 (quite broad!) M K’ Γ K kx Stacking Classical ARPES always give a single π Dirac band even for ky multilayers! Band structure -beam spot is larger than the domain size! First nanoARPES available at Synchrotron SOLEIL Normal synchrotron beam size: 90-100 μm Focused beam size: 100 nm nanoSPOT and nanoPOSITIONING Sample Interferometer Scienta controllers Analyzer NanoARPES is readily compatible to probe the electronic structure of single graphene domains of our samples! OSA ZP Optical microscope Focused beam 3D Piezo-scanner Resolution around 100 nm + High SPATIAL resolution!!! Closed-loop travel 300 x 300 x 300 µm Closed-loop resolution 1 nm k-Microscope Synchrotron radiation ANTARES h Zone Plate Interferometer e- analyser EF Binding Energy (eV) Position Control z x e- y sample Interferometer k//(Å-1) X stage APHY 191 IR Colambo Conventional ARPES (beam size=100 microns) N azimuth W azimuth 1.6 ML FWHM 0.060 Å-1 (quite broad!) M K’ Γ K kx Stacking Classical ARPES always give a single π Dirac band even for ky multilayers! Band structure -beam spot is larger than the domain size! NanoARPES: N azimuth beam size=100 nm 1.6 ML (a) 1 2 Y (μm) X (μm) (a) NanoARPES image from π states near EF => continuous line structures (b) (c) Spectrum at regions (1) (2) => multiple Dirac bands => Consistent with AB stacking NanoARPES: W azimuth (beam size=100 nm) NanoARPES image from π states near EF The broad single Dirac band in classical ARPES is a => domain distribution is less organized superposition of several bands from the different domains probed by the large beam size! (b) Region 1 => two split Dirac bands => AB (occur in very rare cases) FWHM (b) 0.019 Å-1, 0.028 Å-1 (c) 0.035 Å-1 (c) (d) Regions 2 and 3 (d) 0.026 Å-1 => single Dirac band (very sharp!) => either 1 ML or twisted layers NanoARPES (3.4 ML): beam size=100 nm Multiple Dirac bands consistent with 4-6 ML AB N azimuth stacked graphene! Single Dirac bands consistent with either W azimuth 1 ML or twisted domains! APHY 191 IR Colambo NanoXPS vs. nanoARPES 3.4 ML NanoARPES Point 1 – N domain, multiple Dirac band (AB) , 6 ML as shown by the MDC (momentum distribution curve) fitting in (e). Point 2 – no graphene signal along N, so domain is oriented along W NanoCL From the band structure, N Point 1 – N domain domains are typically 4-6 ML higher C 1s intensity … but what about the W (higher thickness) domains?? Point 2 – W domain lower C 1s signal But need to quantify! (lower thickness) nanoXPS 3.4 ML XPS attenuation equation Single Dirac bands observed in W domains are associated to twisted bilayer graphene grains!