Lecture 2 Extensions of Mendelian Inheritance PDF
Document Details
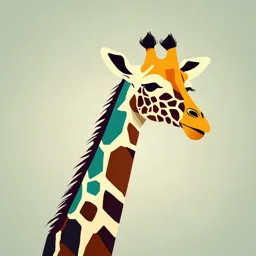
Uploaded by AuthenticField
Craig Lovell
Tags
Summary
This lecture covers extensions of Mendelian inheritance, exploring different inheritance patterns beyond simple dominant-recessive relationships. It details various concepts like incomplete dominance, codominance, and X-linked inheritance.
Full Transcript
EXTENSIONS OF MENDELIAN INHERITANCE © Craig Lovell/Photoshot © McGraw-Hill Education. 4-1 INTRODUCTION Mendelian inheritance describes inheritance patterns that obey two laws Law of segregation Law of INDEPENDENT assortment Simple Mendelian inherita...
EXTENSIONS OF MENDELIAN INHERITANCE © Craig Lovell/Photoshot © McGraw-Hill Education. 4-1 INTRODUCTION Mendelian inheritance describes inheritance patterns that obey two laws Law of segregation Law of INDEPENDENT assortment Simple Mendelian inheritance involves A single gene with two different alleles Alleles display a simple dominant/recessive relationship © McGraw-Hill Education. 4-2 INTRODUCTION (2 OF 2) IN THIS CHAPTER WE WILL EXAMINE TRAITS THAT DEVIATE FROM THE SIMPLE DOMINANT/RECESSIVE RELATIONSHIP THE INHERITANCE PATTERNS OF THESE TRAITS STILL OBEY MENDELIAN LAWS However, they are more complex and interesting than Mendel had realized © McGraw-Hill Education. 4-3 SIMPLE INHERITANCE PATTERNS There are many ways in which two alleles of a single gene may govern the outcome of a trait Table 4.1 describes several different patterns of Mendelian inheritance These patterns are examined with two goals in mind Predict the outcome of crosses Understand the relationship between the molecular expression of a gene and the trait itself © McGraw-Hill Education. 4-4 TABLE 4.1 (1 OF 3) Mendelian Inheritance Patterns Involving Single Genes Type Description Inheritance: This term is commonly applied to the inheritance of alleles that obey Mendel’s laws and follow a strict dominant/recessive relationship. In this chapter, we will see that Simple some genes are found in three or more alleles, making the relationship more complex. Mendelian Molecular: 50% of the protein, produced by a single copy of the dominant (functional) allele in the heterozygote, is sufficient to produce the dominant trait. Inheritance: In the case of dominant traits, this pattern occurs when a dominant phenotype is not expressed even though an individual carries a dominant allele. An example is an individual who carries the polydactyly allele but has a normal number of fingers and Incomplete toes. penetrance Molecular: Even though a dominant allele is present, the protein encoded by the gene may not exert its effects. This can be due to environ-mental influences or due to other genes that may encode proteins that counteract the effects of the protein encoded by the dominant allele. Inheritance: This pattern occurs when the heterozygote has a phenotype that is intermediate between either corresponding homozygote. For example, a cross between Incomplete homozygous red-flowered and homozygous white-flowered parents will produce heterozygous offspring with pink flowers. Dominance Molecular: 50% of the protein, produced by a single copy of the functional allele in the heterozygote, is not sufficient to produce the same trait as the homozygote making 100%. © McGraw-Hill Education. 4-5 TABLE 4.1 (2 OF 3) Type Description Inheritance: This pattern occurs when the heterozygote has a trait that is more beneficial than either homozygote. Overdominance Molecular: Three common ways that heterozygotes gain benefits: (1) Their cells may have increased resistance to infection by microorganisms; (2) they may produce more forms of protein dimers with enhanced function; or (3) they may produce proteins that function under a wider range of conditions. Inheritance: This pattern occurs when the heterozygote expresses both alleles simultaneously without forming an intermediate phenotype. For example, in blood typing, an individual carrying the A and B alleles will have an AB blood type. Codominance Molecular: The codominant alleles encode proteins that function slightly differently from each other, and the function of each protein in the heterozygote affects the phenotype uniquely. Inheritance: This pattern involves the inheritance of genes that are located on the X chromosome. In mammals and fruit flies, males have one copy of X-linked genes, whereas females have two copies. X-linked Molecular: If a pair of X-linked alleles shows a simple dominant/recessive relationship, 50% of the protein, produced by a single copy of the dominant allele in a heterozygous female, is sufficient to produce the dominant trait (in the female). Males have only one copy of X-linked genes and therefore express the copy they carry. © McGraw-Hill Education. 4-6 TABLE 4.1 (3 OF 3) Type Description Inheritance: This pattern refers to the effect of sex on the phenotype of the individual. Some alleles are recessive in one sex and dominant in the opposite Sex-influenced sex. inheritance Molecular: Sex hormones may regulate the molecular expression of genes. This can influence the phenotypic effects of alleles. Inheritance: This pattern refers to traits that occur in only one of the two sexes. An example is breast development in mammals. Sex-limited Molecular: Sex hormones may regulate the molecular expression of genes. inheritance This can influence the phenotypic effects of alleles. In this case, sex hormones that are primarily produced in only one sex are essential to produce a particular phenotype. Inheritance: An allele that has the potential of causing the death of an organism. Lethal alleles Molecular: Lethal alleles are most commonly loss-of-function alleles that encode proteins that are necessary for survival. In some cases, the allele may be due to a mutation in a nonessential gene that changes a protein to function with abnormal and detrimental consequences © McGraw-Hill Education. 4-7 4.2 DOMINANT AND RECESSIVE ALLELES Prevalent alleles in a population are termed wild-type alleles These typically encode proteins that Function normally Are made in the proper amounts Genetic Polymorphism can produce more than one wild- type in large populations © McGraw-Hill Education. 4-8 4.2 DOMINANT AND RECESSIVE ALLELES Alleles that have been altered by mutation are termed mutant alleles They are often defective in their ability to express a functional protein These tend to be rare in natural populations Such mutant alleles are often inherited in a recessive fashion © McGraw-Hill Education. 4-9 4.2 DOMINANT AND RECESSIVE ALLELES In a simple dominant/recessive relationship, the recessive allele does not affect the phenotype of the heterozygote So how can the wild-type phenotype of the heterozygote be explained? There are two possible explanations 50% of the normal protein is enough to accomplish the protein’s cellular function Refer to Figure 4.2 The heterozygote may actually produce more than 50% of the functional protein The normal gene is “up-regulated” to compensate for the lack of function of the defective allele © McGraw-Hill Education. 4-10 A COMPARISON OF PROTEIN LEVELS AMONG GENOTYPES Dominant (functional) allele: P (purple) Recessive (defective) allele: p (white) Genotype: Amount of functional protein P PP: 100% Pp: 50% pp: 0% © McGraw-Hill Education. 4-11 A COMPARISON OF PROTEIN LEVELS AMONG GENOTYPES 2 Phenotype: Simple dominant/recessive relationship PP-Purple Pp-Purple pp-White 12 Access the text alternative for slide images. Genetic diseases are usually caused by mutant alleles In many human genetic diseases , the recessive allele contains a mutation This prevents the allele from producing a fully functional protein © McGraw-Hill Education. 4-13 EXAMPLES OF RECESSIVE HUMAN DISEASES 1 Disease Protein That Is Produced by Description the Functional Gene* Phenylketonu Phenylalanine hydroxylase Inability to metabolize phenylalanine. The effects of the disease ria can be prevented by following a phenylalanine-free diet. If the diet is not followed early in life, the result can be severe mental impairment and physical degeneration. Albinism Tyrosinase Lack of pigmentation in the skin, eyes, and hair. Tay-Sachs Hexosaminidase A Defect in lipid metabolism. Leads to paralysis, blindness, and disease early death. Sandhoff Hexosaminidase B Defect in lipid metabolism. Muscle weakness in infancy, early disease blindness, and progressive mental and motor deterioration. Cystic fibrosis Chloride transporter Inability to regulate ion balance across epithelial cells. Leads to production of thick mucus and results in chronic lung infections, poor weight gain, and organ malfunctions. Lesch-Nyhan Hypoxanthine-guanine Inability to metabolize purines, which are bases found in DNA syndrome phosphoribosyl transferase and RNA. Leads to self-mutilation behavior, poor motor skills, and usually mental impairment and kidney failure. 14 *Individuals who exhibit the disease are either homozygous for a recessive allele or hemizygous (for X-linked genes in human males). The disease symptoms result from a defect in the amount or function of the normal protein. © McGraw-Hill Education. 4-15 DOMINANT MUTANTS Dominant Mutants are much less common than recessive Three explanations for most dominants Toxic Gain-of-function Protein encoded by the mutant gene is changed so it gains a new or abnormal function Dominant-negative Protein encoded by the mutant gene acts antagonistically to the normal protein Haploinsufficiency mutant is loss-of-function heterozygote does not make enough product to give the wild type phenotype © McGraw-Hill Education. 4-16 INCOMPLETE PENETRANCE In some instances, a dominant allele does not influence the outcome of a trait in a heterozygote individual Example = Polydactyly Autosomal dominant trait Affected individuals have additional fingers and/or toes Refer to Figure 4.3 A single copy of the polydactyly allele is usually sufficient to cause this condition In some cases, however, individuals carry the dominant allele but do not exhibit the trait © McGraw-Hill Education. 4-17 INCOMPLETE PENETRANCE © McGraw-Hill Education. 4-18 INCOMPLETE PENETRANCE The term indicates that a dominant allele does not always “penetrate” into the phenotype of the individual The measure of penetrance is described at the population level If 60% of heterozygotes carrying a dominant allele exhibit the trait allele, the trait is 60% penetrant Note: In any particular individual, the trait is either present or not © McGraw-Hill Education. 4-19 EXPRESSIVITY Expressivity is the degree to which a trait is expressed In the case of polydactyly, the number of digits can vary A person with several extra digits has high expressivity of this trait A person with a single extra digit has low expressivity © McGraw-Hill Education. 4-20 EXPRESSIVITY AND INCOMPLETE PENETRANCE The molecular explanation of expressivity and incomplete penetrance may not always be understood In most cases, the range of phenotypes is thought to be due to influences of the Environment and/or Other ‘modifier’ genes © McGraw-Hill Education. 4-21 4.3 ENVIRONMENTAL EFFECTS (1 OF 3) Environmental conditions may have a great impact on the phenotype of the individual Some animals like the arctic fox change coat color grayish brown in summer, white in winter This is an example of a temperature-sensitive allele Humans affected by phenylketonuria (PKU) are unable to metabolize phenylalanine symptoms include mental retardation When detected early, individuals can be fed a restricted diet essentially free of phenylalanine and remain symptom free © McGraw-Hill Education. 4-22 4.3 ENVIRONMENTAL EFFECTS Geneticists often examine a range of conditions when studying the effect of environment on phenotype This allows them to see the norm of reaction of the environmental influence on eye facet number Figure 4.4c shows the norm of reaction for eye facet number in genetically identical flies raised at different temperatures NORM OF REACTION © McGraw-Hill Education. 4-23 4.4 INCOMPLETE DOMINANCE In incomplete dominance the heterozygote exhibits a phenotype that is intermediate between the corresponding homozygotes Example: Flower color in the four o’clock plant Two alleles CR = wild-type allele for red flower color CW = allele for white flower color © McGraw-Hill Education. 4-24 INCOMPLETE DOMINANCE 1 In F1: 50% of the C R protein is not sufficient to produce the red phenotype In F2 : 1: 2 : 1 Is the ratio observed And not the 3:1 ratio observed in simple Mendelian inheritance 25 Access the text alternative for slide images. INCOMPLETE DOMINANCE Whether a trait is dominant or incompletely dominant may depend on how closely the trait is examined Take, for example, the characteristic of pea shape Mendel visually concluded that RR and Rr genotypes produced round peas rr genotypes produced wrinkled peas However, a microscopic examination of round peas reveals that not all round peas are “created equal” © McGraw-Hill Education. 4-26 PHENOTYPE COMPARISON A comparison of phenotype at the macroscopic and microscopic levels Dominant (functional) allele: R (round) Recessive (defective) allele: r (wrinkled) Genotype: Amount of functional (starch-producing) protein RR: 100% Rr: 50% rr: 0% © McGraw-Hill Education. 4-27 PHENOTYPE COMPARISON Phenotype: With unaided eye (simple dominant/recessive relationship) Round Round Wrinkled © McGraw-Hill Education. 4-28 PHENOTYPE COMPARISON Phenotype: With microscope (incomplete dominance) Round Round Wrinkled © McGraw-Hill Education. 4-29 HETEROZYGOTE ADVANTAGE Heterozygote advantage is the phenomenon in which a heterozygote has greater reproductive success compared to both of the corresponding homozygotes It is also called overdominance Example = Sickle-cell anemia Autosomal recessive disorder Affected individuals produce abnormal form of hemoglobin Two alleles HbA Encodes the normal hemoglobin, hemoglobin A HbS Encodes the abnormal hemoglobin, hemoglobin S © McGraw-Hill Education. 4-30 HETEROZYGOTE ADVANTAGE HbSHbS individuals have red blood cells that deform into a sickle shape under conditions of low oxygen tension Refer to Figure 4.7 This has two major ramifications Sickling phenomenon greatly shortens the life span of the red blood cells Anemia results Odd-shaped cells clump Partial or complete blocks in capillary circulation Thus, affected individuals tend to have a shorter life span © McGraw-Hill Education. 4-31 THE HBS ALLELE IS FOUND AT A FAIRLY HIGH FREQUENCY IN PARTS OF AFRICA WHERE MALARIA IS FOUND Malaria is caused by a protozoan, Plasmodium This parasite undergoes its life cycle in two main parts One inside the Anopheles mosquito The other inside red blood cells Red blood cells of heterozygotes, are likely to rupture when infected by Plasmodium This prevents the propagation of the parasite 32 HETEROZYGOTE ADVANTAGE HbAHbS individuals have an “advantage” over HbSHbS, because they do not suffer from sickle cell anemia HbAHbA, because they are more resistant to malaria © McGraw-Hill Education. 4-33 INHERITANCE OF SICKLE CELL DISEASE (a) Normal red blood cell (b) Sickled red blood cell (a): © Mary Martin/Science Source (b): © Science Source © McGraw-Hill Education. 4-34 INHERITANCE OF SICKLE CELL DISEASE (c) Example of sickle cell inheritance pattern © McGraw-Hill Education. 4-35 EXPLANATIONS FOR HETEROZYGOTE ADVANTAGE At the molecular level, overdominance is due to two alleles that produce slightly different proteins But how can these two protein variants produce a favorable phenotype in the heterozygote Well, there are three possible explanations for overdominance at the molecular/cellular level Disease resistance Homodimer formation Variation in functional activity © McGraw-Hill Education. 4-36 1. DISEASE RESISTANCE A microorganism will infect a cell if certain cellular proteins function optimally Heterozygotes have one altered copy of the gene Have slightly reduced protein function Not enough to cause serious side effects, but is enough to prevent infections Examples include Tay-Sachs disease and tuberculosis PKU and fungal toxins © McGraw-Hill Education. 4-37 1. DISEASE RESISTANCE (a) Disease Resistance © McGraw-Hill Education. 4-38 2. HOMODIMER FORMATION Some proteins function as homodimers Composed of two different subunits, encoded by the same gene A1A2 heterozygotes Make A1A1 and A2A2 homodimers AND A1A2 heterodimers For some proteins, the A1A2 heterodimer may have better functional activity Giving the heterozygote superior characteristics © McGraw-Hill Education. 4-39 2. HOMODIMER FORMATION (b) Dimer formation The homozygotes that are A1A1 or A2A2 will make homodimers that are A1A1 and A2A2, respectively. The A1A2 heterozygote can make A1A1 and A2A2 and can also make A1A2 homodimers, which may have better functional activity. © McGraw-Hill Education. 4-40 3. VARIATION IN FUNCTIONAL ACTIVITY A gene, E, encodes a metabolic enzyme Allele E1 encodes an enzyme that functions better at lower temperatures Allele E2 encodes an enzyme that functions better at higher temperatures E1E2 heterozygotes produce both enzymes An advantage in that they function over a wider temperature range than either E1E1 or E2E2 © McGraw-Hill Education. 4-41 3. VARIATION IN FUNCTIONAL ACTIVITY 27° to 32° Celsius (optimum 30° to 37° Celsius (optimum temperature range) temperature range) (c) Variation in functional activity A heterozygote, E1E2, would produce both enzymes and have a broader temperature range (that is, 27° to 37° Celsius) in which the enzyme would function. © McGraw-Hill Education. 4-42 MULTIPLE ALLELES Many genes have multiple alleles Multiple alleles are commonly found within natural populations Typically three or more different alleles However, for genes present in a single copy/haploid genome, a maximum of two alleles are found in any particular diploid individual The ABO blood group provides an example of multiple alleles © McGraw-Hill Education. 4-43 MULTIPLE ALLELES 2 Blood type is determined by the type of antigen present on the surface of red blood cells Antigens are substances that are recognized by antibodies produced by the immune system There are three different alleles that determine which antigen(s) are present on the surface of red blood cells Allele I A , produces antigen A Allele I B , produces antigen B Allele i, does not produce either antigen 44 CODOMINANCE: ABO BLOOD TYPES 1 Allele i is recessive to both I A and I B Alleles I A and I B are codominant They are both expressed in a heterozygous individual 45 Access the text alternative for slide images. CODOMINANCE: ABO BLOOD TYPES 2 The carbohydrate tree on the surface of RBCs is composed of multiple sugars Another sugar can be added by a glycosyl transferase enzyme The i allele codes a defective enzyme The carbohydrate tree is unchanged A I codes a form of the enzyme that can add the sugar N-acetylgalactosamine to the carbohydrate tree I B codes a form of the enzyme that can add the sugar galactose to the carbohydrate tree 46 FORMATION OF A AND B ANTIGENS BY GLYCOSYL TRANSFERASE 47 Access the text alternative for slide images. CODOMINANCE: ABO BLOOD TYPES 3 The A and B antigens are different enough to be recognized by different antibodies For safe blood transfusions to occur, the donor’s blood must be an appropriate match with the recipient’s blood Example: If a type O individual received blood from a type A, type B or type AB blood Antibodies in the recipient blood will react with antigens in the donated blood cells This causes the donated blood to agglutinate A life-threatening situation may result because of clogging of blood vessels 48 4.5 GENES ON SEX CHROMOSOMES Many species have males and females that differ in their sex chromosome composition Certain traits are governed by genes on the sex chromosomes A pedigree for an X-linked disease shows that it is mostly males that are affected with their mothers as carriers Refer to Figure 4.10 © McGraw-Hill Education. 4-49 PEDIGREE FOR DUCHENNE MUSCULAR DYSTROPHY © McGraw-Hill Education. 4-50 PUNNETT SQUARES OF X-LINKED TRAITS Punnett squares of X-linked traits show different results depending on which sex is affected Male can transmit his one X or a Y chromosome An affected male and unaffected female have no affected offspring, but females are carriers An affected female and unaffected male have all male offspring affected and females all carriers Refer to Figure 4.11 © McGraw-Hill Education. 4-51 EXAMPLES OF X-LINKED DYSTROPHY INHERITANCE PATTERNS (b) Examples of X-linked muscular dystrophy inheritance patterns © McGraw-Hill Education. 4-52 SEX CHROMOSOMES AND TRAITS Sex-linked genes are those found on one of the two types of sex chromosomes, but not both X-linked Hemizygous in males Only one copy Males are more likely to be affected Y-linked Relatively few genes in humans Referred to as holandric genes Transmitted only from father to son © McGraw-Hill Education. 4-53 SEX CHROMOSOMES AND TRAITS Pseudoautosomal inheritance refers to the very few genes found on both X and Y chromosomes Found in homologous regions needed for chromosome pairing © McGraw-Hill Education. 4-54 4.6 SEX-INFLUENCED TRAITS Traits where an allele is dominant in one sex but recessive in the opposite sex Thus, sex influence is a phenomenon of heterozygotes Sex-influenced does not mean sex-linked Sex-influenced traits are autosomal © McGraw-Hill Education. 4-55 SEX-INFLUENCED INHERITANCE 1 Example: Scurs (hornlike growth) in cattle Caused by an autosomal gene that exists in two alleles: Allele Sc P is dominant in males, but recessive in females Allele Sc A is dominant in females, but recessive in males Superscript P represents the allele in which scurs are present. Superscript A represents the allele in which scurs are absent Genotype Phenotype in Males Phenotype in Females Sc P Sc P Scurs Scurs Sc P Sc A Scurs No scurs (hornless) Sc ASc A No scurs No scurs 4.6 SEX-INFLUENCED TRAITS (3 OF 3) Scur formation in cattle This trait is characterized by small hornlike growths on the frontal bone. Courtesy of Sheila M. Schmutz, Ph.D. © McGraw-Hill Education. 4-57 SEX-LIMITED INHERITANCE Traits that occur in only one of the two sexes Genes are controlled by sex hormones or the sexual development pathway For example in humans Ovary development is limited to females Testes growth is limited to males In birds males have more ornate plumage © McGraw-Hill Education. 4-58 EXAMPLE OF A SEX-LIMITED TRAIT Feather plumage in chickens (a) Hen (a): © Pixtal/agefotostock RF (b) Rooster (b): © Image Source/PunchStock RF © McGraw-Hill Education. 4-59 4.7 LETHAL ALLELES A lethal allele is one that has the potential to cause the death of an organism These alleles are typically the result of mutations in essential genes They are usually inherited in a recessive manner Essential genes are those that are absolutely required for survival The absence of their protein product leads to a lethal phenotype It is estimated that about one-third of all genes are essential for survival Nonessential genes are those not absolutely required for survival © McGraw-Hill Education. 4-60 4.7 LETHAL ALLELES 2 A lethal allele may produce ratios that seemingly deviate from Mendelian ratios Example: Manx cat Carries a dominant mutation that affects the spine This mutation shortens the tail This allele is lethal as a homozygote 61 EXAMPLE OF MANX INHERITANCE PATTERNS (b) Example of a Manx inheritance pattern © McGraw-Hill Education. 4-62 LETHAL ALLELES Time when lethal effect is exerted can vary Many lethal alleles prevent cell division These will kill an organism at an early age Some lethal alleles exert their effect later in life Huntington disease Characterized by progressive degeneration of the nervous system, dementia and early death The age of onset of the disease is usually between 30 and 50 © McGraw-Hill Education. 4-63 CONDITIONAL LETHAL ALLELES Conditional lethal alleles may kill an organism only when certain environmental conditions prevail Temperature-sensitive (ts) lethals A developing Drosophila larva may be killed at 30° Celsius But it will survive if grown at 22° Celsius (permissive temperature) Typically caused by mutations that alter structure of the protein at the non-permissive temperature © McGraw-Hill Education. 4-64 SEMI-LETHAL ALLELES Semi-lethal alleles Kill some individuals in a population, not all of them Environmental factors and other genes may help prevent the detrimental effects of semi-lethal genes © McGraw-Hill Education. 4-65 4.8 UNDERSTANDING COMPLEX PHENOTYPES CAUSED BY MUTATIONS IN SINGLE GENES Most genes actually have multiple effects Multiple effects of a single gene on the phenotype of an organism is called pleiotropy Can be caused because The gene product can affect cell function in more than one way The gene may be expressed in different cell types The gene may be expressed at different stages of development © McGraw-Hill Education. 4-66 PLEIOTROPIC EFFECTS Cystic fibrosis as an example Normal allele encodes the cystic fibrosis transmembrane conductance regulator (CFTR) Regulates ionic balance by transporting Cl- ions Mutant does not transport Chloride effectively In lungs, this causes very thick mucus On the skin, causes salty sweat Males are often sterile because Cl- transport is needed for proper development of the vas deferens Defect in CFTR can have multiple effects © McGraw-Hill Education. 4-67 PLEIOTROPY One gene has many symptoms or controls several functions Example: porphyria variegata, an autosomal dominant disorder King George III Symptoms appeared every few years in a particular order. Homozygous recessive individual PLEIOTROPY IN Abnormal hemoglobin SICKLE-CELL ANEMIA Sickling of red blood cells Rapid destruction of Clumping of cells and sickle cells leads to anemia interference with blood circulation leads to local failures in blood supply Impaired mental function Pneumonia Heart failure Heart failure Kidney failure Weakness Abdominal and fatigue pain Paralysis 4.9 GENE INTERACTIONS Gene interactions occur when two or more different genes influence the outcome of a single trait Essentially all traits are affected by contributions of many genes Morphological traits such as height weight and pigmentation are affected by many different genes in combination with environmental factors © McGraw-Hill Education. 4-70 TYPES OF MENDELIAN INHERITANCE PATTERNS INVOLVING TWO GENES Type Description Epistasis An inheritance pattern in which the alleles of one gene mask the phenotypic effects of the alleles of a different gene. Complementation A phenomenon in which two parents that express the same or similar recessive phenotypes produce offspring with a wild-type phenotype. Gene modification A phenomenon in which an allele of one gene modifies the phenotypic outcome of the alleles of a different gene. Gene redundancy A phenomenon in which the loss of function in a single gene has no phenotypic effect, but the loss of function of two genes has an effect. Functionality of only one of the two genes is necessary for a wild-type phenotype; the genes are functionally redundant. 71 GENE INTERACTION A Gene interaction can exhibit epistasis and complementation Inheritance of flower color in the sweet pea Also discovered by Bateson and Punnett Lathyrus odoratus normally has purple flowers Bateson and Punnett obtained several true-breeding varieties with white flowers They carried out the following cross P: True-breeding purple X true-breeding white F1: Purple flowered plants F2: Purple- and white-flowered in a 3:1 ratio These results were not surprising Next they crossed different strains of white flowered plants © McGraw-Hill Education. 4-72 CROSS BETWEEN TWO WHITE PEA VARIETIES Complementation: Each recessive allele (c and p) is complemented by a wild-type allele (C and P). This phenomenon indicates that the recessive alleles are in different genes. Epitasis: Homozygosity for the recessive allele of either gene results in a white phenotype, thereby masking the purple (wild- type) phenotype. Both gene products encoded by the wild-type alleles (C and P) are needed for a purple phenotype. © McGraw-Hill Education. 4-73 CROSS BETWEEN TWO WHITE PEA VARIETIES 1 74 Access the text alternative for slide images. CROSS BETWEEN TWO WHITE PEA VARIETIES 2 Complementation: In the F1 generation, each recessive allele (c and p) is complemented by a wild-type allele (C and P). This phenomenon indicates that the recessive alleles are in different genes. Epitasis: Homozygosity for the recessive allele of either gene results in a white phenotype, thereby masking the purple (wild-type) phenotype. Both gene products coded by the wild-type alleles (C and P) are needed for a purple phenotype. 75 CROSS BETWEEN TWO WHITE PEA VARIETIES Thus, the F2 generation contained purple and white flowers in a ratio of 9 purple: 7 white Bateson and Punnett reasoned that flower color is determined by two different genes C (one purple-color-producing) allele is dominant to c (white) P (another purple-color-producing) allele is dominant to p (white) cc or pp masks P or C alleles, producing white color Thus, a plant that is homozygous for either recessive white allele would develop a white flower regardless whether of not the other gene contains a purple-producing allele © McGraw-Hill Education. 4-76 EPISTASIS The term epistasis describes the situation in which a gene can mask the phenotypic effects of another gene Epistatic interactions often arise because two (or more) different proteins participate in a common cellular function Example pathway: colorless Enzyme C colorless Enzyme P purple → → precusor intermediate pigment © McGraw-Hill Education. 4-77 EPISTASIS Recessive alleles do not produce a functional enzyme If an individual is homozygous for either recessive allele It will not make any functional enzyme C or enzyme P Therefore, the flowers remain white Epistasis is when the alleles of one gene mask the phenotypic effects of the alleles of another Epistasis is considered relative to a particular phenotype © McGraw-Hill Education. 4-78 EPISTASIS Note: Mendel’s laws of segregation and independent assortment still hold! Geneticists consider epistasis relative to a particular phenotype. If possible, the wild-type phenotype is the reference The cc and pp genotypes exhibit recessive epistasis © McGraw-Hill Education. 4-79 COMPLEMENTATION The cross of two true-breeding mutant lines with the same phenotype producing wild type offspring colorless Enzyme C colorless Enzyme P purple → → precusor intermediate pigment In this pathway, a mutation in EITHER enzyme results in the SAME phenotype (colorless) This is called complementation Complementation shows that the two mutant lines had the same phenotype caused by mutations in different genes © McGraw-Hill Education. 4-80 In epistasis, two genes interact – alleles of a gene at one locus inhibit or mask the effects of alleles of a different gene at a different locus The result of epistasis is that some expected phenotypes do not appear among offspring Epistasis is an important factor in determining an individual’s susceptibility to common diseases such as insulin resistance EPISTASIS The dominant B allele produces black fur color in BB or Bb Labs – the recessive b allele produces brown fur in bb Labs The dominant allele E of a second gene permits pigment deposition – pigment deposition is blocked in homozygous recessive ee individuals Epistasis by the E gene eliminates some of the expected classes from crosses among Labs – BB ee, Bb ee, and bb ee genotypes all have yellow fur EPISTASIS IN LABRADOR RETRIEVERS A. Black labrador B. Chocolate brown labrador C. Yellow labrador Erik Lam/Shutterstock.com c.byatt-norman/Shutterstock.com c.byatt-norman/Shutterstock.com EPISTASIS IN LABRADORS Black Yellow Homozygous parents: Black F1 puppies: F2 offspring from cross of Gametes from one Bb Ee F1 dog: two F1 Bb Ee dogs: Gametes from another Bb Ee F1 dog: Stepped Art F2 phenotypic ratio is 9 black : 3 chocolate : 4 yellow GENE MODIFICATION A phenomenon in which an allele of one gene modifies the phenotypic outcome of the alleles of a different gene. Example: Feather coloration in parakeets 85 EXAMPLE OF GENE MODIFICATION IN PARAKEETS 1 Feather coloration in parakeets Is regulated by two pigments psittacofulvin eumelanin Psittacofulvin, a yellow pigment, and eumelanin, a black pigment, are distributed in the feathers in varying patterns A wild-type parakeet’s yellow base body color is due to psittacofulvin pigment. Eumelanin crystals, despite being black pigment, reflect only blue light and absorb other wavelengths. The reflected blue combined with the yellow from psittacofulvin pigment, produces a green color 86 EXAMPLE OF GENE MODIFICATION IN PARAKEETS 2 The psittacofulvin gene is designated Y for yellow Dominant allele (Y) results in psittacofulvin synthesis Recessive allele (y) prevents psittacofulvin synthesis The eumelanin gene is designated A for albino Dominant allele (A) results in eumelanin synthesis Recessive allele (a) prevents eumelanin synthesis 87 EXAMPLE OF GENE MODIFICATION IN PARAKEETS 3 (+) Psittacofulvin synthesis only = yellow feathers (+) Eumelanin synthesis only = blue feathers (+,+) Both pigments are synthesized = green feathers (−, −) If neither pigment is synthesized = white feathers Wild-type parakeets possess both pigments and therefore exhibit yellow base body color and green underside. The blue color modifies yellow to green on the underside of these birds, an example of gene modification. The alleles that affect feather color can be dominant or recessive or exhibit incomplete dominance. 88 FEATHER COLORATION IN PARAKEETS TABLE 4.4 Feather Coloration in Parakeets 89 Access the text alternative for slide images. GENE REDUNDANCY Due to gene redundancy, loss-of-function alleles may have no effect on phenotype Geneticists have developed techniques to directly generate loss-of-function alleles This is called a gene knockout Allows scientists to understand the affects of the gene on structure or function of the organism Interestingly, many knockouts have no obvious effect on phenotype © McGraw-Hill Education. 4-90 CAUSES OF GENE REDUNDANCY Gene duplication A species may have two or more copies of similar genes These copies are not identical due to the accumulation of random changes during evolution, called paralogs If one gene is missing, a paralog may be able to carry out the missing function Proteins that are involved in a common cellular function When one of the proteins is missing, the function of another protein may be increased to compensate for the missing protein and thereby overcome the defect © McGraw-Hill Education. 4-91 A MOLECULAR EXPLANATION FOR GENE REDUNDANCY © McGraw-Hill Education. 4-92 GENE REDUNDANCY IN SEED CAPSULE SHAPE Shepherd’s purse seed capsule shape investigated by George Shull Triangular capsule plant crossed with Ovate capsule plant F1 was all triangular F1 self fertilized to make F2 Ratio in F2 was 15 triangular to 1 Ovate At least one copy of T or V present is triangular Only recessive for both genes ttvv is ovate © McGraw-Hill Education. 4-93 INHERITANCE OF CAPSULE SHAPE © McGraw-Hill Education. 4-94