Lecture 10 (1).pdf
Document Details
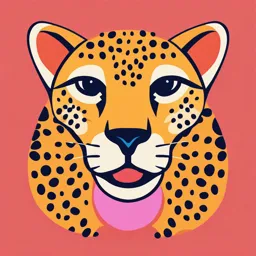
Uploaded by SparklingLoyalty
University College London, University of London
Tags
Full Transcript
Harvesting of Cells for Therapy BENG0011 – Manufacturing Regenerative Medicines: From Lab Bench to Industry Dr Rana Khalife Lecture 10 Lecture overview Harvesting and downstream processing of cells for therapy Preservation and delivery to clinic Overview of a Bioprocess Bio...
Harvesting of Cells for Therapy BENG0011 – Manufacturing Regenerative Medicines: From Lab Bench to Industry Dr Rana Khalife Lecture 10 Lecture overview Harvesting and downstream processing of cells for therapy Preservation and delivery to clinic Overview of a Bioprocess Bioprocess Operation Units General Production Workflow Cell Therapy Processes DSP for Cell Therapies (Pattasseril et al. 2013) Product requirements and DSP (Pattasseril et al. 2013) Autologous vs Allogeneic Dose Requirements (Pattasseril et al. 2013) DSP Technology comparison (Pattasseril et al. 2013) Cell Therapy Processes hMSC microcarrier process example hMSC Bank Microcarrier (P1) Thaw Cell Seeding Monolayer T-Flask Expansion Expansion Microcarrier (Scale-out) Expansion Monolayer Harvest Monolayer Microcarrier Harvest Bioreactor Harvest Downstream Processing Expansion (Scale-up) Filtration Centrifugation Microcarrier Coating Centrifugation Formulation & Hold (up to 4 Hours) Product Formulation Cryo- Product Cell Product Thaw Preservation Bank & Analysis Harvesting papers Harvesting hMSCs from microcarriers Very little published in the literature regarding harvesting – Most harvested 0.5 – 1.0 mL samples Began with microcarrier manufacturer’s protocol “assuming” it would work SoloHill harvesting procedure Where were the cells? …still attached to the microcarriers 100 µm 100 µm Harvest strategy How do we get the cells off the microcarriers? Brief period of intense agitation to aid detachment of cells whilst in contact with dissociation reagent Question: Would this damage the cells? SoloHill harvesting procedure Add dissociation PBS Wash (agitate at Aspirate media reagent 40rpm for 15 min) for 15 min, 37°C Quench dissociation Pipette microcarrier-cell Separate cells from reagent with growth suspension to dislodge microcarriers via medium the cells vacuum filtration SoloHill harvesting procedure 100 mL hMSC spinner flask culture with Plastic microcarriers after 144h (Mean value ± SD, n=4) 1.8e+7 1.6e+7 1.4e+7 hefficiency < 5 % Viable cell number 1.2e+7 1.0e+7 8.0e+6 6.0e+6 4.0e+6 2.0e+6 0.0 Expected harvest Actual harvest Stresses on cells 1. Stress arising as a result of turbulence a) Kolmogorov’s theory of microscales of turbulence Provided the size of the biological entity suspended in flow < Kolmogorov scale, K, then the entity should not be damaged ~ 180 µm K ( / T max ) 3 1/ 4 Equation 1 > Size of biological entity Kinematic viscosity Max. local specific energy dissipation rate close to an impeller ~ 9 x 10-4 W/kg T max T Equation 2 T P / M Po L N 3 D 5 / M Equation 3 Novel harvesting procedure Significantly increased speed for a short time to enhance detachment but less than that at which bubbles would be dragged into the suspension Selected a speed of 150 rpm and a time of 7 min for dissocation Whilst value of T max increased to ~0.11 W/kg, Kolmogorov scale was only reduced to 55 μm, greater than the size of the cells Therefore, once detached, damage to the cells should not occur. It was decided to use this approach to try to improve the technique proposed by SoloHill. Novel harvesting procedure Agitate at 150 rpm with PBS Wash (agitate at Aspirate media dissociation reagent 40rpm for 15 min) for 7 min, 37°C Cell Detachment Quench dissociation Separate cells from Cell concentration via reagent with growth microcarriers via centrifugation medium vacuum filtration Cell Separation Comparison of harvesting procedures hefficiency < 5 % hefficiency > 95 % 1.8e+7 Manufacturer's harvesting method Novel harvesting method 1.6e+7 1.4e+7 Viable cell number 1.2e+7 1.0e+7 8.0e+6 6.0e+6 4.0e+6 2.0e+6 0.0 Expected harvest Actual harvest Expected harvest Actual harvest Novel harvesting procedure Single cells in suspension 100 µm 100 µm Cell quality studies post-harvest Preservation of Cells for Therapy BENG0011 – Manufacturing Regenerative Medicines: From Lab Bench to Industry Lecture overview Part 2 – Preservation and delivery to clinic Cell Therapy Processes Part 2: Preservation and delivery to the clinic Why Preserve? Transport Banking – Consistent starting material – No need for continuous culture- saving cost/time & limiting potential for pheno/genotypic changes and contamination Uncouple… – expansion/manipulation of cells from the clinic or cell- based assays Example: blood products – RBCs refrigerated shelf life up to 42 days stocks kept in hospitals – balance between supply and wastage infusion to be started within 30mins of removal from fridge – Fresh frozen plasma (FFP) Frozen stocks kept in hospital to be defrosted onsite before use – must be used within 24 hours if thawed and stored at 4ºC – Platelets shelf life of 5 days from donation (i.e. more like 3 days) stored at blood bank must be requested by hospital Deciding factors Cell therapy – Autologous – Allogeneic – Scale All linked Business model – Single or multi-site manufacture – On or off-site manufacture Preservation method – Determines transportation – Determines flexibility within the system – Determines level of at-clinic processing Challenges Storage of product – Is this actually a challenge? Storage can be built into the manufacturing site Hospitals are set up to store blood products e.g. monitoring / alarming / record keeping – achievable. – Yes Short-shelf life – impact on business model, ability to carry out QC Do we understand the impact of storage on product? Back up systems Contamination – liquid nitrogen, leaky bags/vials Developing countries? Challenges Transport – The challenge is not availability Refrigerated transport Frozen transport – Dry ice – Dry shippers – expensive, laborious to prepare – The challenge is understanding the impact of transport on the product Delays & shelf life Vibrations & hypothermic storage Fluctuations in temperature (during transport, as product is moved from container to fridge/freezer) Challenges The clinic Can any on-site processing be done? If so, what? – Thawing – Washing? – Cell recovery period? – What is the impact of processing on cells (viability/function)? Matching cell, surgeon and patient availability Levels of investment required in – Equipment – Staff training – technicians/nurses/surgeons/doctors Specialised clinics vs. every hospital – Specialised clinics = ‘cell therapy’ or disease-specific? How to preserve? Cryopreservation – Medium to long-term – Mammalian cells, micro-organisms, plant cells Desiccation – Medium to long-term – Micro-organisms, some mammalian cells Hypothermia – Short-term – Micro-organisms, some mammalian cells When to preserve cells? Cell isolation Cell-based therapies Cell banks Product shelf-life An ideal preservation method… Widely applicable – “platform technology” Maintains cells/organisms’ key characteristics (including clinical function if relevant) GMP compatible Output is easily stored and transported – & can withstand some fluctuations in e.g. temperature, humidity Cell recovery requires minimal manipulation – esp. in the clinic Is cost effective – preservation method + storage/shipping. Cryopreservation Preservation of cells below 0ºC – Typically in liquid nitrogen or its vapour phase 2 options – Slow / conventional / controlled-rate freezing – Vitrification Slow Freezing Typically… Suspend Cells Freeze at Harvest Cells in Freezing ~1ºC/min Solution Wash Thaw cells Cell damage during cryopreservation Avoiding cell damage – Cryoprotective Agents (CPAs) Cryoprotective Agents Penetrating – E.g. Dimethyl Sulphoxide (DMSO) (10% v/v + FBS) for mammalian cells – Replace intracellular water, reduce cell shrinkage – Reduce freezing point of intracellular water- intracellular vitrification occurs before formation of intracellular ice. Non-penetrating – E.g. PEG – Typically used in conjunction with penetrating CPAs Avoiding cell damage - vitrification Avoids formation of extra and intracellular ice crystals Generates amorphous ice Water has a glass-transition temperature of -138°C Very rapid cooling rates needed: vitrification of pure water requires cooling rates of >106°C/min – Requires use of CPAs at high concentrations – increases viscosity, decrease rate of nucleation and ice crystal growth and increase glass-phase transition temperature of the solution enables vitrification to occur at achievable cooling rates (e.g. 20000°C/min) – Requires use of small volumes and/or large surface area Vitrification Typically… Suspend Cells Plunge Harvest Cells in Freezing into LN2 Solution Wash Thaw cells Raises several issues: Uncertainty over whether freezing was the cause Inappropriateness of ‘typical’ testing Lack of understanding over why an ‘established’ technique suddenly failed. Issues: CPA toxicity - DMSO Found in most freezing solutions (including commercially available ones) Cells: – Widely believed to be cytotoxic at temperatures > 0°C – Mechanism poorly understood – As a result told to “work quickly” when cells are in DMSO-containing freezing solution. – Note: has also been reported to induce differentiation Patients: – Can cause adverse reactions if infused into patients Cox et al, 2012, Cell Tissue Bank, 13: 203-215 Issues: DMSO cytotoxicity Hunt et al, 2003, Cryobiol, 46: 76-87 Heidemann et al, 2010, Biotechnol. Prog., 26: 1154-1163 Concluded that for these rBHK cells, a cryobag banking process should not exceed 1.5 hours. Issues: DMSO cytotoxicity There is also a lot of work going into finding alternatives to DMSO and/or reducing the concentration of DMSO used – E.g. trehalose Issues: CPA toxicity in vitrification Very high concentrations required (e.g. ~4 M) of DMSO, ethylene glycol, 1,2-propanediol. Toxic + osmotic imbalance (hence stepwise loading protocols) Protocols which require lower [CPA] are being developed – e.g. He et al, 2008, Cryobiol, 56: 233-232 – e.g. Zhang et al, 2010, Biomed Microdev., 12: 89-96 Alternatives Dessication – Based on inherent capacity of some cells to survive almost complete dehydration Lyophilisation Vacuum desiccation – Medium - Long-term – Would enable transport at ambient temperatures – impact on cost. Desiccation - lyophilisation Also known as freeze drying Removes moisture by sublimation of ice into water vapour Primary Secondary Freezing drying drying Requires the use of lyoprotectants – e.g. trehalose Desiccation - lyophilisation The main focus has been the generation of freeze-dried blood. Freeze-drying mESCs, spermatozoe and sheep granulose cells has resulted in recovery of viable genetic material. Desiccation - lyophilisation A small number of reports are emerging: – Natan et al, 2009, PLoS One, 4:e5240 Mononuclear cells (from umbilical cord blood) – similar levels of CD34+ HSC in population before and after lyophilisation (retained ability to differentiate, ~75% CFU compared to control). Looked at 3 and 7 days, 4°C and ambient, residual moisture content 90% viability, proliferated and expressed key surface markers. Authors admit large inconsistency between runs Desiccation concerns? Impact on cell function Lack of long-term studies – Including impact of variation in storage temperature/humidity Will it be widely applicable? Will this be accepted by the cell biology community or the regulators? Alternatives Hypothermia – “cell pausing” – Based on cold storage of organs - Cells are preserved at >0ºC – Short-term – Mild hypothermia (25-33ºC) – cells can still proliferate, can be used in biopharma to improve protein yield. – Can uncouple cell-culture from cell-based assays/QC testing + enable transport at ambient temperatures or 4ºC – Tested on a range of cell types: Hepatocytes, MSCs, red blood cells, neurons, renal cells, endothelial cells, hESCs Cryopreserva on Cell Pausing Harvest Cells Harvest Cells Media Change Media Change with Addi ves with Addi ves Processing Centre Characterisa on Slow Freeze Cell Pausing Quality tests 1°C per minute (Ambient temp. or 4°C) -80°C Safety tests Processing Centre Efficacy tests Characterisa on Transport to Liquid Nitrogen Quality tests Medical Site APPROVED Vapour phase -196°C -Liquid phase -150°C Safety tests (Ambient temp. or 4°C) Efficacy tests Rewarming & Transport to APPROVED Retrieval for Medical Site (Temperature controlled Cell Therapy equipment) Rewarming & Retrieval for Simplified Process Cell Therapy Hypothermia Some studies have indicated cells can be paused in growth medium: – rCHO and rHEK293 cells paused for up to 3 weeks at 4-24°C. Protein production retained (Hunt et al, 2005, Biotechnol. Bioeng., 89: 157-163) – hESC paused at 4 or 25°C for up to 48 hours retained a normal karyotype and expressed pluripotency markers (Heng et al, 2006, Int J Med Sci, 3: 124-129) Cell changes during cold exposure: – Decreased membrane fluidity – Changes in pH – Osmotic imbalances – Waste product build up – Activation of unfolded protein response – Oxidative stress – Initiation of apoptosis Specialized media have therefore been developed to improve hypothermic preservation Hypothermia- specialised media Cold storage media: – University of Wisconsin Solution – UWS (also known as Viaspan) – EuroCollins – Celsior – Custodiol (also known as Bretschneider’s HTK solution) – HypoThermosol platform – several now available (includes serum- free GMP compliant formulations and a ‘preparation’ solution). Can be administered directly to patients. Hypothermia- specialised media Generally – Mimic ionic composition of cells at cold temperatures – Contain antioxidants (e.g. glutathione) – Provide pH buffering system – Some nutrients Other components tested include – Iron chelators – Apoptosis inhibitors – Anti-freeze proteins Hypothermia- specialised media Recovery can be variable, despite use of specialized media – Can be dependent on medium composition – E.g. Mathew et al, 2004, Tissue Eng, 10:1662-1671 – C3A liver cells Hypothermia- specialised media Is cell function retained? – Clinical trials/industrial use – Variable results in the literature – e.g. Ostrowska et al, 2009, Arch Toxicol., 83: 493-502 Hepatocytes in HypoThermosol-FRS at 4°C for 72 hours 70% viability retained BUT cell metabolic function ~1/2 of freshly isolated cells Hypothermia– concerns Impact on cell function Is a few days long enough? Impact of variation in storage temperature/humidity? Lack of research into rewarming process Widespread applicability Ability to preserve cells in suspension – lack of widespread reporting – 3 clinical trials currently mention HypoThermosol – ReNeuron – patent EP 2367419 A1 Summary: Cryopreservation is the most widely used method of cell preservation Hypothermic storage is becoming widely used for cell therapies Desiccation provides an alternative long-term storage solution for but is in its infancy. Summary: We need to understand our products – Impact of storage, transport, etc We need to learn from and where possible slot into existing processes – e.g. blood products Multiple options for storage of cells, but many factors to consider Recommended Reading- reviews focused on stem cell/cell-based therapy preservation Coopman K, 2011, Biotechnol Prog 27(6): 1511-1521 Thirumala et al, 2009, Organogenesis, 5: 143-154 Hanna & Hubel, 2009, Organogenesis, 5: 134-137 Meryman, 2007, Transfusion, 47: 935-945 Li & Ma, 2012, BioResearch, 1: 205-214 Hunt, 2011, Trans Med Hemother, 38: 107-123 Coopman & Medcalf, 2014, Stem Books, From production to patient: challenges and approaches for delivering cell therapies