Lecture 1 Introduction to Engineering Materials and Their Properties PDF
Document Details
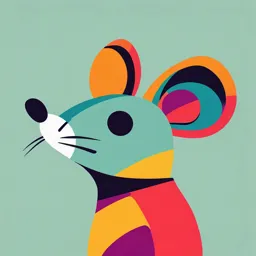
Uploaded by UnabashedConnemara1414
University of Hertfordshire
Dr Mose Bevilacqua
Tags
Summary
This lecture introduces engineering materials and their properties, focusing on characteristics, classifications, and applications. It details examples like cups, bolts, and springs, made from metals, ceramics, and polymers, highlighting their strengths, weaknesses, and usage in specific contexts. The lecture also covers several important material properties and their importance in engineering design.
Full Transcript
Introduction to engineering materials and their properties Dr Mose Bevilacqua Senior Lecturer in Mechanical Engineering School of Physics, Engineering and Computer Science [email protected] Some objects can be made from metals, ceramics or polymers. Something The resulting part to...
Introduction to engineering materials and their properties Dr Mose Bevilacqua Senior Lecturer in Mechanical Engineering School of Physics, Engineering and Computer Science [email protected] Some objects can be made from metals, ceramics or polymers. Something The resulting part to will,Consider however, have different properties and hence be suitable for different applications depending on the material class chosen. Consider the following examples for: A cup A bolt A spring A metal cup: + strong, tough, hard wearing, light, high melting point - good conductor of heat A cup Used by campers / army can be treated roughly and cooked in: stainless steel, Al, enamel (ceramic) coated steel A ceramic cup: + poor conductor of heat, high melting point - brittle (fragile) Used for hot and cold drinks but don’t drop it!: ceramics (china) and glass A polymer cup: + light, cheap, poor conductor of heat - weak, low melting point -Used for hot but more often cold drinks, disposable: PS, PE A metal spring: + strong, tough, hard wearing, high melting point - stiff A Spring Used for most engineering applications: spring steels A ceramic spring: + strong, high melting point, low thermal expansion (stable) - stiff, brittle (fragile in tension) Used for precision instruments / machines: glass A polymer spring: + light, cheap, - weak, low melting point, high expansion - Used for toys: PE, ABS, Nylon A metal bolt: + strong, stiff, tough, hard wearing, high melting point - Used for most engineering applications: engineering steels A Bolt A ceramic bolt: + high melting point, low thermal expansion, insulating - brittle (fragile in tension) Used for niche applications : engineering ceramics A polymer bolt: + light, cheap, - weak, low melting point, high expansion - Used for low stress, low T : Nylon Topic covered in this lecture What is Material Science? Properties of Materials Classifications of Materials The Young’s Modulus Fatigue fracture Examples of fatigue What is Material Science? https://www.youtube.com/watch?v=_cUEjPtVlIM Materials Science is an interdisciplinary subject, spanning the physics and chemistry of matter, engineering applications and industrial manufacturing processes. Modern society is heavily dependent on advanced materials, for example, lightweight composites for faster vehicles, optical fibres for telecommunications and silicon microchips for the information revolution. Materials scientists study the relationships between the structure and properties of a material and how it is made. Why is Material Science important? Material Science the very significant role of materials selection for design and manufacturing processes of new needed products, having the highest attainable quality and performance at the optimum and possibly the lowest cost level. The engineering design processes cannot be set apart either from the material design, being more and more often computer aided, or the technological design of the most suitable manufacturing processes. Why is Material Science important? Materials exhibit countless number of properties, including the following: Mechanical properties (Strength of materials) Chemical properties (Chemistry) Electrical properties (Electricity) Thermal properties (Thermodynamics) A 6 μm diameter carbon filament (running from Optical properties (Optics and Photonics) bottom left to top right) siting atop the much larger Magnetic properties (Magnetism) human hair Properties of Materials Properties of Materials Why is knowledge of material properties so important ? To complete any design, a material that can withstand the service conditions must be selected i.e. it must be capable of withstand ing: the thermal and mechanical stresses the service environment (hot, corrosive, fricti on) and fulfil other requirements ligh t weight, lo w cost Failure to choose the ri gh t material coul d lead to catastrophic failure Properties of Materials How do we choose the right material ? The performance of a material under different service conditions can be defined by the appropriate materials property Values can be used as a metric to compare the benefits of a one material versus another For new materials, these properties can be determined by standardised tests Often it is not one single material property that is important, the cost and ease of manufacture should also be taken into consideration. Properties of Materials Why do we need to understand the basis behind them ? To be able to create new and improved materials and to innovate in design, we need to be able to understand the physical basis for the properties of materials With this knowledge we can tailor the structure or composition of the material, thereby designing a new material with improved properties In this way we can push the envelope for the properties of materials and develop components with greatly enhanced properties and performance Properties of Materials There are thousands of materials available for use in engineering Metals applications. Most materials fall into one of three classes that Ferrous are based on the atomic bonding forces of a particular metals and alloys (irons, carbon steels, alloy steels, material. stainless steels, tool and die Polymeric steels) Thermoplastics plastics Nonferrous Thermoset plastics These three classifications are metallic, ceramic and polymeric. metals and alloys Elastomers (aluminum, copper, magnesium, nickel, titanium, Additionally, different materials can be combined to create a precious metals, refractory composite material. metals, superalloys) Composites Within each of these classifications, materials are often further Ceramics Reinforced plastics organized into groups based on their chemical composition or Glasses Metal-matrix composites Glass ceramics Ceramic-matrix certain physical or mechanical properties. Graphite composites Diamond Sandwich structures Concrete Composite materials are often grouped by the types of materials combined or the way the materials are arranged together. Metals https://www.youtube.com/watch?v=vOuFTuvf4qk Iron/Steel - Steel alloys are used for strength critical applications Aluminium - Aluminium and its alloys are used because they are easy to form, readily available, inexpensive, and recyclable. Copper - Copper and copper alloys have a number of properties that make them useful, including high electrical and thermal conductivity, high ductility, and good corrosion resistance Titanium - Titanium alloys are used for strength in higher temperature (~1000°F) application, when component weight is a concern, or when good corrosion resistance is required Nickel - Nickel alloys are used for still higher temperatures (~1500-2000°F) applications or when good corrosion resistance is required. Refractory materials are used for the highest temperature (> 2000° F) applications. Ceramics A ceramic has traditionally been defined as “an inorganic, nonmetallic solid that is prepared from powdered materials, is fabricated into products through the application of heat, and displays such characteristic properties as hardness, strength, low electrical conductivity, and brittleness.“ The word ceramic comes the from Greek word "keramikos", which means "pottery." They are typically crystalline in nature and are compounds formed between metallic and nonmetallic elements such as aluminum and oxygen (alumina-Al2O3), calcium and oxygen (calcia - CaO), and silicon and nitrogen (silicon nitride-Si3N4). Ceramics Depending on their method of formation, ceramics can be dense or lightweight. Typically, they will demonstrate excellent strength and hardness properties; however, they are often brittle in nature. Ceramics can also be formed to serve as electrically conductive materials or insulators. Some ceramics, like superconductors, also display magnetic properties. They are also more resistant to high temperatures and harsh environments than metals and polymers. Due to ceramic materials wide range of properties, they are used for a multitude of applications. Ceramics The broad categories or segments that make up the ceramic industry can be classified as: Structural clay products (brick, sewer pipe, roofing and wall tile, flue linings, etc.) Whitewares (dinnerware, floor and wall tile, electrical porcelain, etc.) Refractories (brick and monolithic products used in metal, glass, cements, ceramics, energy conversion, petroleum, and chemicals industries) Glasses (flat glass (windows), container glass (bottles), pressed and blown glass (dinnerware), glass fibers (home insulation), and advanced/specialty glass (optical fibers)) Abrasives (natural (garnet, diamond, etc.) and synthetic (silicon carbide, diamond, fused alumina, etc.) abrasives are used for grinding, cutting, polishing, lapping, or pressure blasting of materials) Cements (for roads, bridges, buildings, dams, and etc.) Ceramics Advanced ceramics Structural (wear parts, bioceramics, cutting tools, and engine components) Electrical (capacitors, insulators, substrates, integrated circuit packages, piezoelectrics, magnets and superconductors) Coatings (engine components, cutting tools, and industrial wear parts) Chemical and environmental (filters, membranes, catalysts, and catalyst supports) https://www.youtube.com/watch?v=qRgRV1iMpEM https://www.youtube.com/watch?v=Pp9Yax8UNoM Polymers A polymeric solid can be thought of as a material that contains many chemically bonded parts or units which themselves are bonded together to form a solid. The word polymer literally means "many parts." Two industrially important polymeric materials are plastics and elastomers. Plastics are a large and varied group of synthetic materials which are processed by forming or molding into shape. Just as there are many types of metals such as aluminium and copper, there are many types of plastics, such as polyethylene and nylon. Elastomers or rubbers can be elastically deformed a large amount when a force is applied to them and can return to their original shape (or almost) when the force is released. Polymers Polymers have many properties that make them attractive to use in certain conditions. Many polymers: are less dense than metals or ceramics, resist atmospheric and other forms of corrosion, offer good compatibility with human tissue, or exhibit excellent resistance to the conduction of electrical current Polymers The polymer plastics can be divided into two classes, thermoplastics and thermosetting plastics, depending on how they are structurally and chemically bonded. Thermoplastic polymers comprise the four most important commodity materials – polyethylene, polypropylene, polystyrene and polyvinyl chloride. There are also a number of specialized engineering polymers. The term ‘thermoplastic’ indicates that these materials melt on heating and may be processed by a variety of molding and extrusion techniques. Alternately, ‘thermosetting’ polymers can not be melted or remelted. Thermosetting polymers include alkyds, amino and phenolic resins, epoxies, polyurethanes, and unsaturated polyesters. Polymers Rubber is a natural occurring polymer. However, most polymers are created https://www.youtube.com/watch?v=bYS63HkhoWE by engineering the combination of hydrogen and carbon atoms and the arrangement of the chains they form. The polymer molecule is a long chain of covalent-bonded atoms and secondary bonds then hold groups of polymer chains together to form the polymeric material. Polymers are primarily produced from petroleum or natural gas raw products but the use of organic substances is growing. The super-material known as Kevlar is a man-made polymer. Kevlar is used in bullet-proof vests, strong/lightweight frames, and underwater cables that are 20 times stronger than steel. Composites https://www.youtube.com/watch?v=HMnHlsxwBGI A composite is commonly defined as a combination of two or more distinct materials, each of which retains its own distinctive properties, to create a new material with properties that cannot be achieved by any of the components acting alone. Using this definition, it can be determined that a wide range of engineering materials fall into this category. For example, concrete is a composite because it is a mixture of Portland cement and aggregate. Fiberglass sheet is a composite since it is made of glass fibers imbedded in a polymer. Composite materials are said to have two phases. The reinforcing phase is the fibers, sheets, or particles that are embedded in the matrix phase. The reinforcing material and the matrix material can be metal, ceramic, or polymer. Typically, reinforcing materials are strong with low densities while the matrix is usually a ductile, or tough, material. Composites Some of the common classifications of composites are: Reinforced plastics Metal - matrix composites Ceramic - matrix composites Sandwich structures Concrete Properties of Materials Aims of this section... We shall lo ok at im p ort a nt p ro p ert ie s of ma terial s We wi ll de fi ne t he m an d explain t h e ir releva nce Give me t ho ds f o r m ea su rin g t he p ro pe rt i e s Explain t he o rig i n of t hese p ro pe rt i e s Comp are t he values f o r d if fe re nt ma te rial s Go t hro u gh wo rke d examples of h o w t o use da ta in de si gn pro bl em s an d in t he se le ct ion of ma te rial s Engineering Properties Classes of property Price and availability Economic properties Density Modulus (stiffness) Strength Bulk mechanical Fracture toughness properties Fatigue strength Creep resistance Oxidation and corrosion Surface properties Friction and wear Ease of manufacture and joining Production properties Appearance, texture, feel Aesthetic properties Properties of Materials We shall look at... Stiffness or elastic modulus Elastic properties : Yield, tensile strength, ductility, Plastic properties: toughness, hardness Physical properties: Density Others: Cost The Elastic Modulus Definition... The elastic modulus (or stiffness, E) defines the resistance of a material to elastic deformation It is defined as the ratio (for small strains) of the stress to strain The SI units are those of stress (N/m 2 ) To understand the nature of elastic modulus more clearly we first need to understand the concepts of stresses and strains in materials Data for Engineering Materials Material Young's modulus (E) in GPa Rubber (small strain) 0.05 Low density polyethylene 0.2 Nylon 3 Oak wood (along grain) 11 High-strength concrete (under compression) 30 2 AluminiumAlloy 69 0 Glass (all types) 72 Titanium (Ti) 110 Carbon fibre reinforced plastic 150 Wrought iron and steel 210 Silicon Carbide (SiC) 450 Diamond (C) 1100 Engineering Stress Tensile stress, : Shear stress, : Ft = Ao original area before loading Stress has un its : N/m 2 o r Pa Usually quoted in MPa o r MN/m 2 (MPa = 10 6 Pa) = N/ mm 2 Common States of Stress lOMoARcP 96 25 SD| 9 73 Simple tension: cable F = Ao Ski lift Simple shear: drive shaft F = s Ao Note: = M/AcR here. Other Common Stress States Simple compression: Ao Canyon Bridge, Los Alamos, NM Note: compressive Balanced Rock, Arches structure member National Park ( < 0 here) Elastic Stored Energy lOMoARcP SD| 9 73 96 25 Stored energy per unit volume of material (or work done in J/m3 ) can be determined from the area under the stress-strain curve The maximum elastic (recoverable) energy stored corresponds to an applied stress equal to the elastic limit The stored energy can be determined considering either the applied stress or strain alone using the elastic modulus W= =E W= W= 2 2E 2 Summary Stress and Strain... Materials respond to stress by straining, At low stresses and strains the deformation is elastic and obeys Hooke’s law Hooke’s law states that stress is proportional to strain. The constant of proportionality is the Elastic modulus (also called Young’s Modulus or stiffness, E) =E Stiffer materials (higher elastic modulus) will strain (or deflect) less at a given stress For a given stress, stiffer materials will store less Stress-Strain (tensile) Testing Typical tensile testing machine and data logger NB We need an extensometer to measure small displacements accurately in order to measure E Von Mises Stress Yield criterion Recalling the Tensile Test (Cylindrical Specimen) Load Cell: Measures the Force Stress Strain Grip 𝐹 Δ𝑙 Extensometer: 𝜎= 𝜀= Measures the Displacement 𝐴0 𝑙0 Grip F F Von Mises Stress Yield criterion Stress Yield Stress Ultimate Tensile Stress Fracture Elastic Plastic - Necking Uniform Plastic the slope is called Young’s modulus 𝜎 = 𝐸𝜀 Strain Von Mises Stress Yield criterion http://www.youtube.com/watch?v=W5A8gU37wGg Von Mises Stress Yield criterion Stress-Strain Curve – Mild Steel, Aluminium, etc. Stress Yield Stress Draw a parallel line at Strain = 0.2% Strain Von Mises Stress Yield criterion ▪ Generally, structures should be designed so that they are stressed below Yield. ▪ Can you think of a practical example in which you deliberatly stress a material above Yield? Ductility is the amount of plastic deformation a material can withstand without rupture. This is an important property of most metal alloys. Von Mises Stress Yield criterion Fractures in Steel Ductile Brittle Ductility and Toughness Ductility is an indication of how much plastic strain a material can withstand before it breaks.. The larger the strain the more ductile the material. The opposite is brittl e Ductility is a strain and is unitless. It is often described as the % elongation or reduction in a rea at fractu re Toughness is the resistance to fracture of a material when stressed and is defined as the energy needed to break a unit volume of material (in J/m3 ) Brittle is the opposite of tough. A brittl e material will absorb very little energy durin g fracture The strength of a material is its ability to withstand an applied load without failure or plastic deformation Ductility, % Elongation l MoARcP O 9625 SD| 9 73 Plastic tensile strain at failure: %EL = L f − L o x100 Lo As the strength of a material increases the ductility decreases A − Af %AR = o x100 Another ductility measure: Ao Toughness (J/m3) l MoARcP O 9625 SD| 9 73 Energy to break a unit volume of material Given by the area under the stress-strain curve Engineer ing smaller toughness (ceramics) tensile larger toughness stress, (metals, PMCs) smaller toughness- unreinforced polymers Engineer ing tensile str ain, Ceramics - high strength - low ductility - low toughness Metals - high strength - high ductility - high toughness Polymers - low strength - high ductility - low toughness Energy to Fracture (Charpy impact) l MoARcP O 9625 SD| 9 73 The energy to fracture can also be measured from a Charpy impact test (in J/m2) The test can be used to compare materials and the effect of test temperature A swinging pendulum impacts a notched sample the energy absorbed is calculated from the reduced height to which the pendulum swings (PE loss) lOMoARcP SD| 9 73 96 25 Origin of Ductility and Toughness The origin of ductility is the ability to undergo plastic deformation. Plastic flow readily occurs in metals (dislocations) so they are ductile, it is limited in ceramics, they are brittle. If the chains can slide easily in polymers they are ductile too Toughness is also dictated by the degree to which plastic flow can occur. Since it is given by the area under the stress – strain curve, a good balance of strength and ductility give a high toughness Plastic flow” the deformation of a material that remains rigid under stresses of less than a certain intensity but that behaves under severer stresses approximately as a Newtonian fluid NB fracture toughness relates to the fracture behaviour in the presence of cracks (we will look at this later) Typical Stress-Strain Curves lOMoARcP 96 25 SD| 9 73 Tough (ductile) or brittle Hard or soft Strong or weak Examples: Ductility and Toughness l MoARcP O 9625 SD| 9 73 Calculate the total and the plastic strain to failure (ductility). What is the energy absorbed per m3 for this material ? Engineering stress, 80 MPa 40 MPa Ductility = plastic strain at failure (must remove the elastic strain !) 0.25 (25%) Toughness = area under the curve Engineering strain, Examples: Ductility and Toughness Total strain = 0.25 Engineering stress, Ductility = 0.2 Toughness = area under the curve this fracture point x y can be approximated These are parallel to a parallelogram Area = base x height f 0.25 approx = 0.2 x 60x10 6 0.2% engineering strain, = 12 MJ/m 3 0.20 Examples: Ductility and Toughness A Charpy impact test sample is fractured by a swinging pendulum hammer. If the 7kg hammer swung from a height of 2.00 m, through the sample to a height of 1.25m, how much energy did the sample absorb during fracture ? PE before = m g h = 7 x 9.81 x 2.00 = 137 J PE after = m g h = 7 x 9.81 x 1.25 = 86 J PE loss = energy absorbed = 51 J This could be converted to J/m 2 but cannot be compared with the energy under the stress – strain curve Summary: Ductility and Toughness Ductility is a measure of the plastic strain of a material at failure. The larger the strain the more ductile the material. Ductility is a strain and is unitless. It is often described as the % elongation or reduction in area at fracture Toughness is the resistance to fracture of a material when stressed and is defined as the energy needed to break a unit volume of material (in J/m3). It is the area under the stress-strain curve The origin of ductility and toughness is the ability to undergo plastic deformation. Those materials unable to undergo plastic flow (ceramics) will be brittle and will absorb very little energy during fracture Hardness Hardness is an expression of a material’s resistance to indentation, more specifically its resistance to localised plastic deformation. The harder the material, the smaller the indent under a given applied load Hardness is determined by the load over the projected area of the indent Hardness has non SI units of force divided by area (kgf/mm2) but should not be confused with pressure as the area considered is not normal to the applied force The kilogram-force is equal to the magnitude of the force exerted on one kilogram of mass in a 9.80665 m/s2 gravitational field Hardness tests are made on the surface of a material and are hence “non-destructive”. It is ideal for quality control and for measuring surface properties Hardness Different test methods have different shaped indenters, the Vickers method uses a pyramid shape made from diamond (as it is harder than everything else) The indent size (l) for a given applied load is measured and a hardness value HV (for Vickers) is determined by: Hardness is reported as xxxHvyy e.g. 200H v 20 is a hardness of 200 measured by the Vickers method with a load of 20kgf Hardness l MoARcP O 9625 SD| 9 73 Resistance to permanently indenting the surface. Large hardness means: --resistance to plastic deformation or cracking in compression (high strength) --better wear properties Because the deformation is compressive ceramics can be tested ! Hardness Hardness / Yield Stress / Mpa Vickers hardness can be converted Material kgf/mm2 to MPa by multiplying by g (9.81). This converted value is then Diamond 8400 54100 approximately 3 times the yield Alumina 2600 11300 stress of the material Tungsten Carbide 2100 7000 Steel 210 700 9.81Hv y = Annealed copper 47 150 3 Annealed aluminium 22 60 Lead 6 16 i.e. 210H v gives y = 687 MPa Nb the table shows real data for Hv and y Relevance of Hardness The selection of a material may not be driven by hardness alone BUT it is a good indication of the yield strength of the material and its resistance to indentation and wear Hardness measurements are also good for rapid assessment of the properties of a material as might be required by quality inspections (as it is simple, can be portable and is rapid). It is often used to check between batches and to ensure processing and heat treatments have had the desired effects on the properties Example: Hardness 2 1 lOMoARcP 96 25 SD| 9 73 A Vickers hardness test is performed on a material with a 20kgf load and produces a square indent of diagonal length 0.66mm. What is the hardness and the approximate yield stress of the material ? y = 9.81Hv 3 Hv = 1.854*20/0.66 2 = 85Hv20 (keep in mm to give Hv) Convert to MPa by multiplying by 9.81 = 834 y = 834/3 = 278 MPa Summary: Hardness Hardness is an expression of a material’s resistance to plastic deformation Hardness is determined by indentation methods, the harder the material, the smaller the indent Hardness has non SI units of force divided by area (kgf/mm2). It can be converted to an approximate yield stress for a material by multiplying by (9.81/3). Hardness is a good indication of the strength and wear resistance of a material. The “non-destructive” surface measurements are ideal for quality control and for measuring surface properties Which properties are we really trying to optimise? Wings: Stiffness-limited Landing Gear: Strength-Limited Turbine Blade: Creep-Limited Fan Blades: High Cycle Fatigue-Limited Nuclear Pressure Vessels: Low Cycle Fatigue Limited Material Young’s Yield Stress Density E/ρ Σy/ρ Example Modulus E (GPa) σy (MPa) ρ (MJ/kg) (kJ/kg) (kg/m3) CFRP 120 1200 1600 75 750 Fuselage Ti 5553 110 1400 4500 24 310 Landing Gear Ti 6Al 4V 105 900 4500 23 200 Fan Blade Alloy 7075-T6 Al 70 550 2700 26 200 Wing Skin A300M Steel 210 2050 7830 27 262 Landing Gear DC 10 Failure Flight 232 1989 United Airlines Flight 232 traveling from Philadelphia from Denver The aircraft manufacturer is a McDonnel Douglas DC 10 – 10 The Aircraft was delivered to United Airlines in 1971 At the moment of the crash it flew 42.401 Hours and it made 17 take-off and landing cycles DC 10 Failure Flight 232 1989 The NTSB concludes that the failure of the Engineer No.2 was due to a microscopic imperfection of the Titanium alloy used to make the Engine Fan disk This imperfection developed a crack which slowly grew over the course of 17 years Despite being regularly inspected, the location of the crack make it difficult to detect Thank you for listening Questions?