Lec 4-5 PDF - Microbial Cell Structure and Function
Document Details
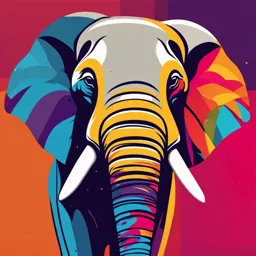
Uploaded by ResplendentMountainPeak
Tags
Summary
This document provides lecture notes on microbial cell structure, including details on morphology, sizes, membranes, transport mechanisms, and different types of cells.
Full Transcript
PowerPoint® Lecture Presentations CHAPTER 2 Microbial Cell Structure and Function © 2018 Pearson Education, Inc. Microbial morphology 2 Microbial morphology • = Cell Shape Major cell morphologies Unusual shapes Microbial morphology • Some cells remain in groups or clusters after cell divi...
PowerPoint® Lecture Presentations CHAPTER 2 Microbial Cell Structure and Function © 2018 Pearson Education, Inc. Microbial morphology 2 Microbial morphology • = Cell Shape Major cell morphologies Unusual shapes Microbial morphology • Some cells remain in groups or clusters after cell division in characteristic arrangements that can assist in identification Morphology & Phylogeny • Morphology typically does not predict physiology, ecology, or phylogeny of a prokaryotic cell • Rod shaped Bacteria and Archaea may look identical under the microscope • Morphology is a genetically encoded property that maximizes fitness in a particular habitat • Optimization of nutrient uptake • Swimming motility in viscous environments or near surfaces • Gliding motility (filamentous bacteria) Cell size E. fishelsoni • Size range for prokaryotes can vary from 0.2 µm to more than 700 µm in diameter • Average rods are 1 x 2 µm • Most eukaryotes are 8 µm or larger Paramecium (eukaryote) • Examples of very large prokaryotes • Epulopiscium fishelsoni • Lives symbiotically in the gut of surgeonfish • Longer than 600 µm (0.6 mm) • Thiomargarita namibiensis • Cocci found in ocean sediments of the continental shelf of Namibia • Largest known prokaryote, with diameter of 400 – 750 µm Chain of T. namibiensis At about 1cm long, Thiomargarita magnifica is roughly 50 times larger than all other known giant bacteria. SCIENCE 23 JUN 2022 VOL 376, ISSUE 6600 The advantage of being small • Small cells have more surface area relative to cell volume than large cells • = High surface to volume ratio • Supports greater nutrient exchange per unit cell volume • Tend to grow faster than larger cells • Limited resources can support a larger population of small cells than of large cells • More cell division = more mutations = faster evolution Lower limits of cell size • Volume must house all essential components of a free- living cell • Proteins, nucleic acids, ribosomes, etc. • 0.1 µm is insufficient, and 0.15 µm is marginal • Open oceans tend to contain very small cells • 0.2 – 0.4 µm in diameter • Many pathogenic bacteria are very small • Genomes are streamlined with some gene functions provided by hosts The cytoplasmic membrane • Thin structure that surrounds the cytoplasm and separates it from the environment • Holds all of the contents of a cell in one place • Disruption of the cytoplasmic membrane causes cell death • The cytoplasmic membrane regulates traffic of substances into and out of the cell • = Selective permeability Cytoplasmic membrane • Semi-fluid phospholipid bilayer with embedded proteins • Phospholipids are composed of both hydrophobic (fatty acid) and hydrophilic (glycerolphosphate) components • In aqueous solution, phospholipids naturally form bilayers • Fatty acids point inward; hydrophilic portions remain exposed to external environment or the cytoplasm Membrane proteins • Integral membrane proteins are permanently embedded in the membrane • Transmembrane integral proteins span the membrane • Other integral membrane proteins have one portion of the protein inserted into the membrane but do not span the membrane • Lipid-anchored proteins have a lipid molecule attached to an amino acid, which inserts into the membrane • Peripheral membrane proteins are associated with membrane surfaces (polar heads of phospholipids, other proteins) without inserting into the membrane Out Phospholipids Hydrophilic groups 6–8 nm Hydrophobic groups In Peripheral proteins Phospholipid molecule Lipid-anchored protein Transmembrane protein Integral membrane proteins Membrane structure in Archaea • Instead of fatty acids, hydrocarbons are derived from isoprene units • Isoprene = 5 carbon branched molecule • Hydrocarbons are attached to glycerol by ether linkages rather than ester linkages Archaeal lipids Two major types: • Glycerol diethers • Two phytanyl groups attached to glycerol • Phytanyl = 20-carbon branched hydrocarbon (4 isoprene units) • Diglycerol tetraether • Two biphytanyl groups attached to a glycerol molecule at both ends • Biphytanyl = 40-carbon branched hydrocarbon • Forms a lipid monolayer instead of a lipid bilayer • Some archaea lipids contain cyclic rings in the hydrocarbon chains • e.g. crenarchaeol • Increases rigidity Archaeal membranes • May be a lipid bilayer, a monolayer, or a combination • Lipid monolayers are extremely resistant to heat and are widely distributed among hyperthermophilic Archaea • Species that grow best at >80°C Permeability • Hydrophobic portion of the membrane is a tight barrier to diffusion of many substances • Water is polar but sufficiently small to pass between phospholipid molecules • Also transported by membrane proteins called aquaporins that accelerate its movement Transport mechanisms in prokaryotes Simple transport: A membrane-spanning transport protein, typically driven by the proton motive force Group translocation: A substance is transported into the cell while being chemically modified at the same time. ABC transporter: Transport is mediated by substratebinding protein (1), the membrane transporter (2) and an ATP hydrolyzing protein (3). The Cell Wall • Protects against lysis & confers shape and rigidity to the cell • Gram-positive and gram-negative bacteria differ in staining due to differences in cell wall structure • Gram-positive bacteria have an inner cytoplasmic membrane and thick layer of peptidoglycan • Gram-negative “cell envelope” consists of inner cytoplasmic membrane, thin layer of peptidoglycan, and outer membrane Gram-positive vs. Gram-negative Gram-positive vs. Gram-negative Gram-positive Gram-negative Peptidoglycan • A polymer composed of a repeating subunit called a glycan tetrapeptide • Consists of two sugar derivatives & amino acids • N-acetylglucosamine and N- acetylmuramic acid • L-alanine, D-alanine, D-glutamic acid, and L-lysine or a lysine derivative (e.g. Diaminopimelic acid) • The enzyme lysozyme can cleave bonds between sugars, weakening cell wall • Present in tears, saliva & other body fluids http://www.youtube.com/watch?v=-sct8GUye-0&feature=related Peptidoglycan • β-1,4-glycosidic bonds connect sugars in long chains, while peptide bonds connect one glycan chain to another • Glycosidic bonds create rigidity and strength in one direction (X), while peptide bonds confer strength in other direction (Y) G = N-acetylglucosamine M = N-acetylmuramic acid Peptidoglycan • In gram-negative bacteria, peptide bonds between chains occur between DAP of one chain and D-alanine of another chain • In gram-positive bacteria, connection occurs via a short string of peptides (= interbridge) that vary from species to species • In S. aureus = Five glycine residues • More than 100 distinct peptidoglycans varying in peptide content have been described Gram-positive cell wall • Up to 90% peptidoglycan • Several cross-linked glycan strands form into “cables” about 50 nm wide • Cables cross-link to form even stronger structure • Also contain teichoic acids • Polymers of glycerol or ribitol joined by phosphate groups • Covalently connected to peptidoglycan or plasma membrane lipids • Negatively charged LPS: The outer membrane • In Gram-negative bacteria, the cell wall consists of a thin layer of peptidoglycan plus an outer membrane • Outer membrane is a lipid bilayer, but is different in composition from the inner membrane • Contains phospholipids, proteins and lipopolysaccharides (= LPS) • LPS replaces much of the phospholipid in the outer half of the outer membrane • Lipoproteins connect the peptidoglycan layer to the outer membrane Structure of LPS • LPS molecules consist of: • Lipid A • Disaccharide of glucosamine phosphate linked to fatty acids through amine groups • Core polysaccharide • Only minor variations between species within a particular genus (e.g. Salmonella), but more variation between genera (e.g. Salmonella vs. Escherichia) • O-specific polysaccharide • Highly variable between different species Periplasm & Porins • Periplasm = region between two membranes • Very high concentration of proteins, including hydrolytic enzymes (degrade food molecules) bindingproteins (transport) and chemoreceptors (cell movement) • Outer membrane is relatively permeable to small molecules due to the presence of porins • = Proteins that function as channels for entrance and exit of solutes • May be specific or nonspecific Porin Gram-negative cell wall What about the Gram stain? • Crystal violet ions (CV+) form an insoluble complex with iodine (I-) inside the cell • Alcohol dehydrates thick cell wall of gram-positive cells causing pores to close & trapping the purple CVIodine • In gram-negative cells, alcohol is able to penetrate into the cells and extracts the CV-Iodine complex • Gram-negative cells are nearly invisible until stained with the second dye Life without a wall? • Most prokaryotes can’t survive without a cell wall • However, some naturally lack cell walls • E.g. Thermoplasma and relatives in Archaea • E.g. Mycoplasma – group of pathogenic bacteria • These organisms either have unusually tough cell membranes or live in an environment with strict osmotic regulation Cell walls of Archaea • Archaea cell walls do not contain peptidoglycan & typically lack an outer membrane • Polysaccharide Walls • Pseudomurein cell walls • Similar to peptidoglycan but contains N-acetylglucosamine linked to N- acetyltalosaminuronic acid (instead of N-acetylmuramic acid) • β-1,3 linkages (instead of β-1,4) • = Lysozyme insensitive • Walls may also contain other polysaccharides instead of pseudomurein • Some Archaea in high salt environments have polysaccharide walls that are highly sulfated (SO42-) Pseudomurein Cell walls of Archaea: S-Layers • Most common type of cell wall in Archaea • Also found in some Bacteria • Formed from interlocking layers of protein or glycoprotein that form a paracrystalline structure • May be present alone or in combination with a polysaccharidebased layer • Always outermost wall layer • Protects from osmotic lysis, acts as a selective sieve & retains proteins near surface Cell inclusions • Granules, crystals, or globules of a substance in the cytoplasm, usually partitioned off from the rest of the cell • Usually store a substance during times of excess for later use, e.g. • Carbon • Usually poly-β-hydroxybutyric acid (PHB, a lipid) • Inorganic phosphate (PO43-) • Stored in polyphosphate chains • Sulfur • Stored by sulfur bacteria that obtain electrons by oxidizing sulfide Gas vesicles • Present in many planktonic prokaryotes • Spindle-shaped, gas-filled structures made of protein • Impermeable to water but permeable to gases • Gas is less dense than the cell itself • Increases buoyancy • Allows cells to adjust vertical position in a water column Capsules & slime layers • Sticky or slimy materials on cell surface, consisting of secreted polysaccharide or protein • Capsules consist of a tight matrix that adheres firmly to the cell wall • Readily visible by light microscopy • Slime layers are loosely attached and can be lost from the cell surface • Function in attachment, immune invasion, and protect from dehydration Bacterial capsules: Fimbriae and Pilli • Filamentous proteins extending from surface of cell • Fimbriae enable cells to stick to surfaces • Pilli are longer & thicker, with fewer present on cell surface • Many different types • Facilitate genetic exchange by conjugation • Adhesion to specific host tissues • Twitching motility Flagella • Threadlike locomotor appendages extending outward from the plasma membrane and cell wall Polar • Function in swimming & motility • Different arrangements • Polar: Flagella attached at one or both ends • Lophotrichous: A tuft (group) of flagella at one end • Amphitrichous: Tufts at both ends • Peritrichous: Many locations around the cell Lophotrichous Peritrichous Flagella structure • Three main components: • Basal body: The base & “motor” of the flagella • Anchors the flagella in cytoplasmic membrane & cell wall. • Hook: Links basal body & filament • Filament: Extends from the cell surface to the tip • Composed of a hollow rigid cylinder of flagellin protein • Helical • Basal body motor rotates the flagella much like the propeller on a boat motor • Rotation powered by proton (H+) movement through Mot proteins in the basal body Archaea flagella • Power swimming by rotation, much like Bacteria, however…. • Thinner in diameter than Bacteria • = slower swimming • Made of several different flagellin proteins with little similarity to Bacteria flagellin • Powered directly by ATP rather than proton motive force • Flagellar motility believed to have evolved separately in Bacteria & Archaea 60 cells lengths/sec 25 body lengths/sec Swimming • Flagella increase or decrease rotational speed in relation to strength of the proton motive force • Swimming speed is genetic • Different species have different maximum speeds • Different flagella arrangements exhibit differences in swimming motions • Peritrichously flagellated cells typically move slowly in a straight line • Polarly flagellated cells move more rapidly, spinning around and dashing from place to place Movement in peritrichous and polarly flagellated prokaryotes Forward motion is powered by counterclockwise (CCW) rotation Gliding motility • Slower and smoother than flagellar swimming • Requires cell contact with a solid surface • Mechanisms: • Secretion of polysaccharide slime • Contacts both the surface & the cell and pulls cell along • Type IV pili “twitching motility” • Repeated extension and retraction of pilli drags cell along the surface • Gliding-specific proteins, e.g. • M. xanthus: Protein complex forms at one pole and remains at fixed position on the solid surface as cell glides forward • Flavobacterium: Glide proteins anchored in the outer membrane move against a solid surface to pull the cell forward by a “ratcheting” mechanism Chemotaxis • Movement toward a chemical attractant, or away from a chemical repellant • Chemicals bind to membrane proteins called chemoreceptors, which transmit the signal internally & alter flagella movement High concentration of repellant No repellant Chemotaxis in E. coli • In absence of an attractant, cells move randomly in the environment by runs (smooth forward swimming) & tumbles (stop & jiggle about) • If moving in a gradient of an attractant, runs in the direction of the attractant are longer, and the cell tumbles less Measuring Chemotaxis Other taxes • Taxis: directed movement in response to chemical or physical gradients • Chemotaxis: response to chemicals • Phototaxis: response to light • Aerotaxis: response to oxygen • Osmotaxis: response to ionic strength • Hydrotaxis: response to water Phototaxis of a colony of Rhodospirillum centenum towards a light source The Bacterial Endospore • A highly differentiated thick-walled structure produced by certain gram-positive bacteria • Resistant to many environmental conditions, including heat, harsh chemicals, radiation, nutrient depletion, and desiccation • Can remain dormant indefinitely & germinate quickly when conditions warrant • Easily dispersed by wind, water, or animal gut The Bacterial Endospore • Life cycle of vegetative cell endospore vegetative cell • Mature endospore is located in a characteristic location in the mother cell, depending on the species of bacteria Endospore formation Endospore structure • Outermost layer is thin protein covering called the exosporium • Moving inward are several spore coats consisting of layers of sporespecific proteins • Cortex is peptidoglycan that is less cross-linked than normal wall • Core consists of cytoplasmic membrane, cytoplasm, nucleoid, ribosomes & other cellular essentials surrounded by a core wall (peptidoglycan) Exosporium Spore coat Cortex Core wall DNA Why so resistant? • Coat protects from chemicals and exogenous enzymes • Core contains high levels of dipicolinic acid and calcium (Ca2+) • Complex together and bind free water, dehydrating the core • Insert between DNA bases & help stabilize it • Dehydration of the core: • Increases resistance to heat and toxic chemicals • Renders enzymes inactive but does not denature them • High levels of small acid-soluble spore proteins (SASPs) • Bind tightly to DNA & protect from damage • Function as a carbon and energy source during germination Reactivation Occurs in three stages: 1. Activation • Prepares spore for germination • Often results from heating 2. Germination • Environmental nutrients are detected • Water uptake and breakdown of cortex • Increased metabolic activity 3. Outgrowth • Exit spore coat & return to vegetative state