BIOL 2020 Lab 1 - Cell Culture Guide Fall 2023 PDF
Document Details
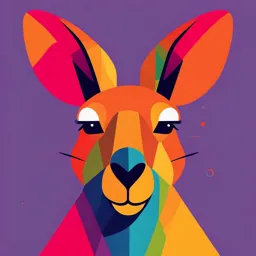
Uploaded by RejoicingOstrich
2023
McCarville, Garant and Tatar
Tags
Summary
This document is a guide for a biology lab focusing on cell culture, apoptosis, morphology, and viability. It provides instructions, learning outcomes, and information on the experimental procedures. The guide will involve looking at different cell types under microscopes.
Full Transcript
Cell Culture: Apoptosis, Morphology & Viability Apoptotic Ptk2 cells stained with Trypan Blue. Photo: M McCarville Welcome to the BIOL 2020 labs! We are glad you are here! These labs are designed as a multi-week experiment that simulates the workflow of a real cell biology research lab. The curric...
Cell Culture: Apoptosis, Morphology & Viability Apoptotic Ptk2 cells stained with Trypan Blue. Photo: M McCarville Welcome to the BIOL 2020 labs! We are glad you are here! These labs are designed as a multi-week experiment that simulates the workflow of a real cell biology research lab. The curriculum provides opportunities for you to experience authentic lab skills. (It is a bonus that these activities are super fun 😊) This semester we will focus on apoptosis, a type of programmed cell death. This highly controlled, orderly process of self-destruction happens in normal, healthy cells in response to various types of cell signaling. Today we start this term-long experiment by stressing mammalian cells via acid shock or oxidative stress. After the cells have had time to respond to the stressor, you will observe cell morphology and viability to determine if the cells have become apoptotic. In later weeks you will use molecular techniques to investigate protein expression in stressed and non-stressed cells. General Lab Etiquette and Important Reminders: • To avoid congestion in the room, please store your jackets and backpacks in the coat rack area inside the lab or under your bench. • Discard all pipette tips, broken glass, cover slips, and other sharp objects in the yellow sharps container on your bench or in the large cardboard box labeled “BROKEN GLASS”. • The Cell Lab is a (mostly) device-free zone! For this reason, you will not be able to access electronic versions of the lab documents. We encourage you to print everything in advance so you have paper to make notes, but we will also have copies of the lab guides on each bench. • Always leave your bench clean and tidy. Aim to re-set the items so that they are arranged in the same way as they were when you arrived. • You will complete an in-lab worksheet during the lab session. You are encouraged to work with your partner and/or benchmates. Each student will submit their own worksheet prior to leaving. It is okay (and expected!) that your responses may be similar or identical to those of your partner(s). • Your flowchart is due at the beginning of the lab. Your TA will collect it when you arrive. McCarville, Garant and Tatar (2023/2024) Preparing for Lab! Learning Outcomes: By the time this lab is complete, you should be able to: • • • • Demonstrate pipetting proficiency. Describe how cells can be maintained in vitro. Appreciate the difference between brightfield and phase contrast microscopy. Calculate cell concentration using a hemocytometer. BEFORE Lab 1, you must: • • • • • Carefully read the rest of this document and print it. (However, hard copies of the lab guides will be available on the benches.) Watch the Pipetting Video on Brightspace. Watch the Brightfield and Phase Contrast Microscopy Video on Brightspace. Complete a flowchart for Lab 1. (See instructions on Brightspace in the Lab Content folder!) Complete the pre-lab quiz on Brightspace. (This quiz includes content from this document and also from the Syllabus!) DURING Lab 1, you will: • Be introduced to the Cell Bio labs and meet the teaching team. 😊 • • • • Practice micropipetting. Set-up a simple experiment using mammalian cells. Examine cells using brightfield and phase contrast microscopy. Complete the In-Lab Worksheet (provided to you during the lab) and submit before you leave. Now let’s get started! 😊 McCarville, Garant and Tatar (2023/2024) BIOL 2020 Labs: Big Picture! Throughout the term, we will compare stressed and unstressed Ptk2 cells. We will look at: • Morphology (what does the entire cell look like?) • Protein Expression (what happens to the expression of a particular protein?) • Protein Localization (what happens to the location of a particular protein?) Lab 1: Cell Culture (Morphology and Viability) You will stress Ptk2 cells using either vinegar (acid shock) or hydrogen peroxide (oxidative stress). You will also have a flask of unstressed cells. After 30 minutes, you will compare cell morphology between stressed and unstressed cells and estimate how many cells in each culture are living or dead. Prior to Lab 2: During the summer, Mindy and Laura prepared cell lysates from stressed (labelled “T” for “Treatment”) and unstressed (labelled “C” for “Negative Control”) Ptk2 cells. You will use the cell lysate in Labs 2, 3 and 4. Lab 2: Protein Extraction and Quantification You will receive tubes of Ptk2 cell lysate proteins that Mindy and Laura prepared during the summer. Your task is to determine the total protein concentration in each sample by performing a Bradford Assay. Lab 3: SDS-PAGE You will separate the Ptk2 cell lysate proteins from the previous week on an SDSPAGE gel and transfer the proteins to a nitrocellulose membrane. This is in preparation for Lab 4, where you will use western blotting to test for the expression of key proteins that are involved in apoptotic pathways. Lab 4: Western Blotting To complete the apoptosis experiment, you will use antibodies to test for specific apoptotic proteins (your options are p53 or Hsp27) to determine if its expression has changed in response to the stressor. (i.e. did the cell make more of that protein, less of that protein, or the same amount of that protein in response to the stressor?) Lab 5: Fluorescence: (Not on apoptosis, but super duper awesome) To conclude the term, you will use immunofluorescence and DNA-binding dyes to visualize microtubules and nuclei, respectively, in Ptk2 cells. This lab is not directly part of the apoptosis experiment, but it does demonstrate another elegant technique that uses antibodies to test for the presence and location of proteins in a cell. McCarville, Garant and Tatar (2023/2024) Cell Culture: Definitions + Biosafety: The ability to grow cells in vitro is a fundamental part of molecular biology research. Growing cells in the lab can also be used for clinical purposes (diagnostics, personalized medicine) and for practical biotechnology (production of proteins, pharmaceuticals, and A biological safety cabinet. viruses). Photo: M. McCarville. Primary cells are taken directly from a tissue. Unless modified in some way, these cells have a finite lifespan. They may divide a few times but will eventually die. Primary cells are excellent for some applications because they closely resemble in vivo physiology (i.e. they are more representative of the cells in the organism), but they have the disadvantage of being a bit trickier to grow, and ultimately, dying. Immortal (‘Continuous’) cell lines divide indefinitely. These have originated as cancerous cells, or they were transformed in the lab to give them this ability. The advantages of immortalized cells are that they are easier to grow and that they divide over and over. However, they are less representative of in vivo systems, and with time acquire increased levels of genetic modifications. Cell lines are generally described as being either adherent or suspension. Adherent cells attach to a substrate and cells growing in suspension do not. Adherent cells will eventually grow and divide to the point where they form a solid monolayer, with minimal space between the cells. This is referred to as 100% confluency. Depending on the particular cell line, some cells will stop growing at this point, while other types of cells (like cancer cells) may continue growing on top of each other. Some of the most common immortal cell lines that are used in labs around the world include: • • • • HeLa – the first human immortal cell line, established in 1951, derived from cervical cancer tumor HEK 293 – human embryonic kidney epithelial cells SF9 – insect cells that can be grown in suspension Other cancer cells: MCF-7 (breast cancer), Saos-2 (bone cancer), PC3 (prostate cancer) Risk Group (RG) Classifications: All microorganisms, proteins, and nucleic acids are assessed to determine their risk to the individual/animal and public health. They are assigned to one of four risk group (RG) categories. These risk group classifications are determined by the Public Health Agency of Canada and the Canadian Food Inspection Agency. Risk Group 1: Very low risk. e.g. Saccharomyces cerevisiae (yeast), Ptk2 cells. Risk Group 2: These pathogens are capable of causing serious disease in a human but are unlikely to do so. For example, Listeria monocytogenes, HEK293, Norwalk virus, SARS-CoV-2 RNA. At Dalhousie, all human cell lines are treated as Risk Group 2. Risk Group 3: These pathogens are likely to cause serious disease in a human or an animal. e.g. Mycobacterium tuberculosis, SARS-CoV-2 whole live virus. Risk Group 4: These pathogens can produce highly contagious, serious or fatal diseases for which there are no treatments or vaccines. e.g. Ebola virus. McCarville, Garant and Tatar (2023/2024) Containment Facilities: Containment levels refer to the minimum physical containment and operational practices required for safe handling of infectious materials and toxins. There are four containment level (CL) classifications: CL1: This is a “regular” type of teaching lab. They do not have any special design features other than a functional working space and cleanable work surfaces. Open bench work is acceptable and Biological Safety Cabinets are not required. Our regular lab rooms (LSC 7009 and 7012) are CL1 workspaces. CL2: This is a common type of facility in hospitals and universities for either diagnostic, health-care work or for research purposes. Generally, all RG2 pathogens are contained in CL2 facilities. These rooms have BSCs that are equipped with HEPA filters. The tissue culture rooms in the LSC are CL2. CL3: These laboratories require additional primary and secondary barriers to minimize the release of infectious organisms into the environment. This includes sealed windows, the use of a BSC for all work and strictly controlled access. Canada has over one hundred CL3 laboratories. Anyone who is doing research with COVID is working in a CL3 lab. CL4: These facilities provide the maximum level of biosafety and biosecurity. Maximum containment is ensured via a complete seal of the facility perimeter, which includes sealing any conduits crossing the containment barrier, such as electrical conduits and plumbing. The lab worker must wear a full coverage positivepressure suit with its own breathing supply. Personnel wearing these full coverage suits proceed through a chemical shower before removing protective clothing. There are only two CL4 laboratories in Canada – the National Microbiology Laboratory, and the Canadian Science Centre for Human and Animal Health, both housed in Winnipeg. Personal Protective Equipment (PPE) Cell Biology labs require that students wear the following PPE: Lab Coats: These will help to protect you from contamination via spills, splashes and splatter. Lab coats should be removed prior to leaving the lab. It should NEVER be worn outside the lab in order to reduce the risk of releasing potentially contaminated material. You should bring in your own lab coat to the BIOL 2020 labs, but there will be extra lab coats available to borrow in the lab if you don’t have one. It is also recommended that you tie long hair back to prevent contact with chemicals and solutions. Proper Footwear: Open toed shoes, flip-flops, and sandals are NOT allowed in the BIOL 2020 lab. This type of footwear simply cannot protect your skin from spills. Note that you will NOT be allowed to stay in the lab if you show up with inappropriate lab footwear L Gloves: Before you put gloves on, you should check them to make sure there are not rips or tears, or signs of deterioration. If you have an open sore on a hand, you should put on a bandage, and use double gloves. When you remove your gloves, you should peel them off so that each glove is turned inside out. Always assume that the gloves are now contaminated and immediately throw them away. NEVER re-use gloves. Once your gloves are off, immediately wash your hands with soap and water. McCarville, Garant and Tatar (2023/2024) Ptk2 Cell Line: BIOL 2020 students will use a marsupial cell line (Ptk2 cells) for all the experiments! These cells are epithelial kidney cells from a male potoroo, Potorous tridactylis. In 1962, Kirsten Walen and Spencer Brown isolated kidney cells from this small marsupial and put them in culture dishes, where they continued to replicate (Walen and Brown, 1962). Note that despite their rat-like appearance, and the common name ‘rat-kangaroos’, potoroos are indeed relatives of the kangaroo, and are not rodents! As you might expect, potoroos are The long-nosed potoroo. found in the wild in Australia. Photo: Wikipedia The potoroo has only a small number of chromosomes (males have five pairs of autosomes, plus two X chromosomes and one Y chromosome). Walen and Brown observed that the Ptk2 cells stayed relatively flat in culture, making it quite easy to see their large chromosomes. Combined, these characteristics made this cell line ideal for studying genetics and the cell cycle. However, they grow nicely in culture, and are Ptk2 cells growing in culture. a great cell line to use in BIOL 2020! The cells you will be using are the distant Photo: M. McCarville descendants of the Ptk2 cells originally isolated by Walen and Brown! Because these cells are RG1, we can work with them in our teaching lab (a CL1 lab). Cell Culture Equipment: A Biological Safety Cabinet (BSC), i.e. the “hood”, is the single most critical piece of equipment needed for working in a CL2 lab. A BSC protects you from infectious materials or toxins AND protects your specimens from contamination. Note: although superficially they may look very similar, a BSC is NOT a fume hood (which simply sucks the air from the hood out a vent, without filtering)! A properly functioning BSC creates an air curtain across the front opening. This prevents aerosols from escaping out the front AND prevents unfiltered air from entering the working area. This air curtain is fragile, so care must be taken to not disturb it. When the researcher starts working, they slowly and deliberately put their hands into the hood, coming in from the front, and then they wait a few seconds to allow the air curtain to be re-established. Inside the hood, the air moves in a constant, streamlined speed and direction, which creates a laminar flow that contains airborne infectious agents. The air from the cabinet is exhausted through a HEPA (High Efficiency Particulate Air) filter. BSCs also maintain a sterile work environment by filtering the incoming air through a HEPA filter before it blows across the working surface. A CO2 Incubator is required for short-term storage of growing cells. The incubator provides a clean, humidified environment with a constant temperature (usually 37˚C for mammalian cells!) The incubators are supplied with 5% CO2 which maintains the pH at physiological level. For long term storage, cells are cryopreserved in liquid nitrogen. Benchmarkscientific.com McCarville, Garant and Tatar (2023/2024) Inverted microscopes have the lens on the bottom of the microscope and the light source above the specimen. This type of microscope is necessary as cells are usually growing on the bottom of the flask and there is often condensation on the top of the flask. Additionally, flasks and dishes are bulky and the large stage provides an adequate space to view the vessel. Commercially available media is used to provide the nutrients that the cells require. Media typically contains about 5-10% fetal bovine serum (FBS). Antibiotics such as penicillin and streptomycin are often added to the media to minimize the risk of bacterial growth following contamination. The media is pink due to the presence of the pH indicator Phenol Red. If the pH becomes acidic the media will turn orange; if the pH becomes basic it will turn purple. A change in the pH indicator colour is a sign that there is an excess of metabolic by-products and that it is time to either split the cells (also referred to as “passaging” or “subculturing”) if they are confluent or change the media to replenish depleted nutrients. Mammalian cells are grown in specialized culture vessels that have been treated to allow adherent cells to attach to the bottom surface. Examples include flasks, which have screw tops that can be either vented or non-vented, and plates, which have individual wells. Setting up experiments using cell cultures: Many experiments in cell biology test the effect of a single variable on some sort of measurable phenotype (i.e. something you can see or observe). For example: • When cells are exposed to drug X, Is the expression of Protein Y up-regulated or down-regulated? • Does temperature influence the subcellular location of Protein Z? • When cells are infected with virus A, does the functionality of Protein B decrease? We set up these types of experiments by: 1. Growing large quantities of cells. 2. Collecting the cells in a tube and determining the concentration of those cells. 3. Diluting the collected cells with media to a final concentration of 1 x 105 cells/ml (which is a commonly used seeding concentration.) 4. Once the cells are diluted, they are seeded into flasks or plates to start an experiment! Experimental Sample and Controls Terminology: In our multi-week experiment in the Cell Bio labs, our variable is STRESS! (either via acid shock or oxidative stress). The cell sample that tests the effect of the variable is referred to as the “Treatment” or the “Experimental” sample. In our experiment, we are labeling samples as “T” to indicate the sample that was stressed. The sample that is NOT exposed to the variable is referred to as the “Negative Control”. This demonstrates what will happen in the absence of the variable, like a baseline. It is a way to ensure that your observed results are due to the variable, and are not because of another, unknown factor. The negative control should be manipulated in an identical manner to the experimental sample with the exception of the variable (e.g. no temperature change, no pharmaceutical agent added, etc.) In our experiment, we are labeling the unstressed samples as “C”. McCarville, Garant and Tatar (2023/2024) Task #1: Micropipetting The most accurate way to transfer small volumes of liquid is to use a micropipettor. Today you will have access to three different micropipettors; a P20, a P200, and a P1000. Each of these pipettes is optimized to dispense a specific range of volume. You will know which one is which by reading the label at the top of the plunger. Pick up one of the pipettes in front of you and observe how it fits in your hand. Make sure your thumb is on the top (i.e. the plunger). Which pipette are you holding? What range of volumes will it dispense? To set the volume, turn the thumbscrew on the handle and read the volume in the window. P20 P200 P1000 1 – 20 µl 20 – 200 µl 200 – 1000 µl Numbers in black indicate the volume from 1 to 20 µl. The red number on the bottom is tenths of a microliter (i.e., the first decimal point). The red notches on the bottom indicate hundredths of a microliter (i.e., the second decimal point). Each notch is 0.02 µl. = 15.60 µl = 1.56 µl All the numbers are black and indicate the volume from 20 – 200 µl. The black notches on the bottom indicate tenths of a microliter (i.e., the first decimal point). Each notch is 0.2 µl. The top position in red indicates 1000 µl. Unless you are pipetting a volume of exactly 1000 µl, this number will always be zero. The remaining numbers are black and indicate the volume from 200 to 999 µl. The black notches indicate individual microliters (i.e., the ones column). Each notch is 2 µl. = 122.8 µl = 43.7 µl = 1000 µl = 354 µl Tip: Even though the notches go by 0.02, 0.2 or 2 µl (depending on the micropipettor being used), you can always set the micropipettor to an odd number by positioning the arrow between two notches. McCarville, Garant and Tatar (2023/2024) Try transferring tiny volumes of liquid! Use the volumes provided to practice transferring blue liquid into a microcentrifuge tube. To micropipette like a pro, you should: • • • • • Set the correct volume and put on a disposable tip. Push the plunger to the FIRST stop only. Do not push further than the first stop! Put the tip into the solution and gently and smoothly release the plunger. Move the pipette to a new tube and slowly push the plunger back down to the first stop. You may see that there is still a tiny bit of liquid in the tip; push down to the second stop to expel this remaining liquid. It is tricky, but stay steady and keep the plunger pressed down. • Carefully remove the pipette from the tube, release the plunger, and eject the tip. • Extra helpful hint… beware of bubbles. Bubbles are bad. (Bubbles and froth take the place of liquid, meaning that you are not transferring the volume you think you are transferring!) What about when you need to transfer a volume larger than one ml? A common pipette that is capable of this type of transfer is a PipetBoyTM. These batterypowered devices allow you to transfer liquids quickly and accurately. After pushing a sterile pipette into the nozzle, you can use the top button to suck up liquid and the bottom button to dispense liquid. Practise transferring larger volumes of liquid! Use the volumes provided to practice transferring blue liquid into a waste beaker. To use a PipetBoy like a pro, you should: • • • • • • • Avoid touching the part of the pipette that will be in contact with the solutions. Avoid bumping the pipette on any surface. Push on the upper button to draw up the liquid. DO NOT suck the liquid up into the PipetBoy!! Watch the liquid level at all times. Push on the lower button to expel the liquid. Dispose of the pipette by manually pulling the pipette from the PipetBoy. Like the other pipettes, beware of bubbles. Bubbles are still bad. McCarville, Garant and Tatar (2023/2024) Task #2: Induce apoptosis by exposing your Ptk2 cells to a stressor: FYI: Most of the time, work with mammalian cells is performed in a tissue culture room in a Biological Safety Cabinet. However, if the goal is to quickly check cell morphology and viability (like what we are doing today), then it is not critical to maintain that same level of aseptic technique. 😊 Ptk2 cells are a RG1 cell line, so they are safe to manipulate in a CL1 lab, like the BIOL 2020 labs! Approximately 24 hr prior to your lab time, we seeded (i.e. “placed”) Ptk2 cells into 6-well plates containing glass coverslips. By the time you arrive in the lab, the cells should be well adhered to the top surface of the coverslip and have grown to our desired confluency. Four of the wells will have cells on a coverslip. The middle two wells are not needed today and are empty. Pair #1 Treatment Pair #2 Treatment Pair #1 Negative Control Pair #2 Negative Control Treatment Options for Inducing Apoptosis in Ptk2 Cells: Pair # 1: Oxidative Stress Hydrogen peroxide will create oxidative stress in the cells by generating reactive oxygen species. This type of stress is related to many human diseases and conditions. We will provide you with hydrogen peroxide at a concentration of 3% (the same as what you can find at the drugstore). It is suggested that you add a volume in the range of 5 to 30 µl to each well. Your choice! Pair #2: pH Stress Regular vinegar purchased from a grocery store is 5% acetic acid. Adding even just a little bit to the media will drop the pH and serve as an acid shock. A small amount of experimentation suggests that an addition of 10 µl will be sufficient to drop the pH to ~6, while an addition of 50 µl will be enough to drop it to ~5. You can choose how much to add, within a range of 10 to 50 µl. The trick here is to stress the cells enough to trigger apoptosis, but not to shock them so badly that they freak out and die via necrosis! (Necrosis is also a type of cell death, but it is less orderly… rather more like cells going “SPLAT”!) This is a delicate balancing act, and as is most often the case with real science, we don’t have a definitive answer as to what you should do. Try something out and see what happens! Once you have determined what volume of stressor you will add to your Treatment cells, you will need to think carefully about how to set up an appropriate negative control for your experiment. The negative control cells will also need something added to them. What liquid should you add? What volume should you use? Now that you have chosen what you are going to test, go ahead and add the appropriate volumes of solutions to the appropriate wells and set a timer for 30 min. While you wait, you will set up your microscope and get prepared to check out your cells. McCarville, Garant and Tatar (2023/2024) Skill #3: Real-world Microscopy: Once the cells have incubated for 30 min, you will use a microscope to: • • Observe morphology/shape of the cells. Estimate the percentage of living vs dead cells Light Microscopy using the Olympus CX41 microscope The Olympus microscopes in the Cell Lab have ocular lenses with 10x magnification and objective lenses that are 10x or 40x. Total magnification is the product of the ocular and objective lenses. At 10x: the total magnification is (10x)(10x) = 100X At 40x: the total magnification is (10x)(40x) = 400X Before you can use the microscope, you must first ensure that the light path from the light bulb, through the field iris, through the condenser iris, to your eye is perfectly aligned. This is done using a process called Kohler illumination. We have aligned the microscope for you, so you should not see any shadows or dark spots. Phase Contrast Microscopy Small, unstained specimens such as living cells can be difficult to see using Brightfield. Phase contrast makes highly transparent objects more visible by converting differences in light phase shifts into differences in light intensity. Phase contrast is ideal for examining thin, living specimens. Phase contrast is made possible by aligning a phase plate that sits at the back of each objective with a special filter in the turret. To achieve maximum contrast, this alignment is critical. Quick Set-up for Phase Contrast: • Plug in the microscope and turn it on. • Ensure the turret is set to the appropriate setting for phase contrast. The turret setting will be written on the barrel of the objective. For example, at 10x, the correct turret position would be “Ph1”. For 40x, the correct turret position is “Ph2”. Each objective has a different setting. • Place a corn (Zea mays) stem slide onto the stage and focus on the image. TIP: The BEST way to do this is to bring the stage ALL THE WAY UP and then, while looking through the eyepiece, slowly bring the stage down until your image is focused. This way you will not miss seeing the sample on the slide! • On Phase, the image will be dark - play with the light intensity by adjusting the knob beside the off/on switch. McCarville, Garant and Tatar (2023/2024) Skill #4: Observe Cells and Determine Cell Viability: Trypan Blue is a common stain that is used to determine cell viability (i.e. how many cells are alive and how many are dead?) Dead, or dying cells with compromised cell membranes will allow the blue dye to leak into the intracellular space. It is frequently called an “exclusion test” as living cells with intact cell membranes will exclude the dye and remain clear/grayish in colour. Clear/gray cells = Alive! Blue cells = Dead! • Once your cells have had 30 min to respond to the treatment, retrieve the 6-well plate. • Retrieve two glass slides. Use a Sharpie to label one “T“ and one “C”. • Put 25 µl of Trypan Blue onto each slide. • Use the sharp dissecting tool to lift the coverslip (this is tricky!) and grab it with the tweezers. Carefully touch the edge of the coverslip to a KimWipe to remove excess media. • Place the coverslip cell side down onto the drop of Trypan Blue on the slide. • Make sure there is no liquid surrounding the coverslip and put the slide onto the microscope stage. • Using the 10x objective, focus on the cells using Brightfield (turret setting = “O”). • Staying at 10x, change the setting to Phase Contrast (turret setting = “Ph1”). • Consider which of the two settings is easier for you to see the cells. Using your preferred setting, carefully increase magnification to the 40x objective. (Remember, if you prefer the phase contrast setting, you will need to change the turret setting to “Ph2”!) Observe Cells: Visually, does it appear that the stressed cells have become apoptotic? There are many sorts of molecular markers that can be used to confirm apoptosis, but there are also a few features that should be visible with light microscopy: • Membrane blebbing. Once apoptosis signaling pathways have been initiated, the cell will start to fragment and small vesicles will bud off from the membrane. This is one of the most defining characteristics of apoptotic cells. • Cell shrinkage. As the cell fragments, it will become smaller in size. In contrast, necrotic cells will swell and appear larger than normal. Determine Viability: Estimate the percent of living and dead cells in your stressed (“T”) and unstressed (“C”) samples. You do not need to count the cells, an approximation is fine! McCarville, Garant and Tatar (2023/2024) Skill #5: Determine Cell Concentration: The previous step (examining the coverslip in trypan blue) was a quick and easy way to get a sense of what was happening with your cells. However, if we are seeding cells in new flasks to set up an experiment, it becomes important to accurately determine cell concentration. The standard way to count cells is to use a hemocytometer, a specialized slide with an etched grid. Cells are pipetted into a chamber on top of the grid, which makes it easy to count how many cells are in each block. There is a hemocytometer set up (without cells in the chamber) on the side bench. Go over and take a look at it so that you understand what is meant by “the grid”. Often cells are mixed with an equal volume of trypan blue (e.g. 20 µl of cells + 20 µl of trypan blue) before they are loaded into the hemocytometer. Unless the cell concentration is SUPER high or low, we count the cells in the 4 corner squares. (See image above – A, B, C, and D) Cells per 0.1 µl = Number of cells you counted Number of large squares containing counted cells Psst… The calculation above represents the concentration of cells once they have been mixed with trypan blue. This is not particularly helpful for setting up an experiment as you need to know the concentration of cells that you initially collected! Multiply by the dilution factor (see right!) to bring the number back up to the actual concentration. Dilution = volume sample + volume diluent Factor volume sample Finally, note that this calculation will give number of cells per 0.1 µl. However, it is more practical to have the concentration represented as cells per ml, so multiply by 104 to make the conversion. 😊 To summarize: Cells per ml = Number of cells you counted Number of large squares containing counted cells X Dilution Factor X 104 At this point, you can move on to the in-lab worksheet. 😊 You are encouraged to work through the questions with your partner(s), and it is acceptable for group members to submit similar or identical answers. However, everyone will need to pass in their own paper! McCarville, Garant and Tatar (2023/2024)