L4 NEUR3101 Spinal control 1 PDF
Document Details
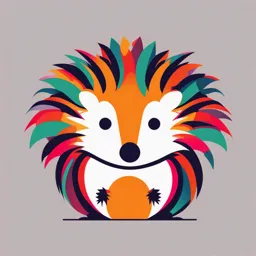
Uploaded by ThoughtfulRetinalite
null
null
A/Prof Ingvars Birznieks
Tags
Summary
These lecture notes cover spinal control of movement and locomotion, focusing on reflexes, muscle afferent pathways, and central pattern generators (CPGs). The material draws on information from various neuroscience textbooks, including Purves et al., Kandel et al., and Bear et al.
Full Transcript
NEUR3101 Motor control Lecture 4 Spinal control of movement and locomotion Reflexes controlled by muscle and cutaneous afferents A/Prof Ingvars Birznieks Chapter 16, Purves et al., Neuroscience (6th Ed) Chapters 35-36, Kandel et al., Prin...
NEUR3101 Motor control Lecture 4 Spinal control of movement and locomotion Reflexes controlled by muscle and cutaneous afferents A/Prof Ingvars Birznieks Chapter 16, Purves et al., Neuroscience (6th Ed) Chapters 35-36, Kandel et al., Principles of Neural Science (5th Ed) Chapter 13, Bear et al., Neuroscience: exploring the brain (4rd Ed) Learning Outcomes You should be able to: Describe the spinal reflex and its components Describe how reflexes are modulated accordingly to the task Describe various muscle afferent reflex pathways ( e.g., Ia, Ib) Describe different classes of muscle receptors, and demonstrate their role in motor control Demonstrate how rhythmic movements are controlled and describe the role of central pattern generators (CPGs) Spinal reflex What is a spinal reflex? An involuntary response to activation of a sensory receptor that is mediated through spinal pathways. The concept of a “reflex” is changing Once perceived as “hard-wired” (i.e. always get the same response to a given stimulus) now even the simplest reflex is viewed as highly modifiable Now the concept of reflex modulation predominates - a reflex response depends on the context/task being performed - reflexes are incorporated with the voluntary motor command Spinal reflex arc Sensory systems controlling reflexes Proprioception Proprioception from Latin proprius, meaning "one's own", "individual" and perception, is the sense of the relative position of neighbouring parts of the body position of limbs and other body parts in space strength of effort being employed in movement Specialised mechanoreceptors – proprioceptors (“receptors for self”) Muscle spindles Golgi tendon organs Joint receptors To be able provide this information signals from specialised proprioceptors have to be integrated with signals from other receptor types and sensory systems: Vestibular sensory system Skin (cutaneous) mechanoreceptors may provide proprioceptive information to signal body part location by sensing pattern of skin stretch Visual system (also plays an important role continuously calibrating the proprioceptive system) Proprioception Muscle spindles Extrafusal muscle fibres – true force producing fibres of the muscle. Intrafusal muscle fibres – part of the sensory organ - muscle spindles. Keep sensory elements stretched to be able to maintain sensitivity to changes in stretch regardless of the overall muscle length. Primary endings or group Ia afferents – show rapidly adapting responses to changes in muscle length. Provide information about velocity of the movement. Secondary endings or group II afferents – produce sustained response to muscle length, thus largely provide information about extent of muscle stretch. ɤ-motor neurons activate intrafusal muscle fibres and by changing tension significantly impact on sensitivity of muscle spindles. α-motor neurons activate extrafusal (force producing) muscle fibres The highest density of muscle spindles is in extraocular muscles, intrinsic muscles of the hand and muscles of the neck. Figure 9.7 Muscle spindles are not present in middle ear muscles. Co-activation of α and γ motor neurons Muscle spindles respond to muscle stretch, but muscle contraction shortens muscle rather than stretches it Figure 16.11 Functions of spinal reflexes Rapidly respond to perturbations Allow very fast initiation of corrective responses following an unexpected perturbation Examples: Stretch reflex, Cutaneous reflex, Flexor withdrawal reflex Contribute to the motor control and movement adjustments Take care of the details of movement execution to unload higher control centers Reflexes can be highly organised and modulated accordingly to the task A perturbation of one arm causes an excitatory reflex response in the contralateral elbow extensor muscle when the contralateral limb is used to prevent the body from moving forward by grasping a table. The same stimulus produces an inhibitory response in the muscle when the contralateral hand holds filled cup. Kandel Schwartz & Jessel (2000). Principles of Neural Science 4/e McGraw-Hill. Muscle stretch reflex Stretch reflex also known as “myotatic”, “deep tendon”, “knee-jerk” or “patellar” reflex is a monosynaptic reflex with biological function to maintain muscle at the desired length. The afferent neuron is connected to a muscle spindle, which detects stretch in a muscle. The efferent neuron is a motor neuron, which causes muscle to twitch. Figure 13.7 Different ways to evoke a spinal reflex Rotation of joint (most natural stretch reflex) Tendon tap Electrical stimulation (H-Reflex) Muscle stretch reflex Biological function of the stretch reflex is to maintain muscle at a desired length. From the control point of view stretch reflex is a feedback control mechanism. Deviation from a desired length is detected by muscle spindles. The increase or decrease in stretch of muscle spindles alter their discharge rate, which directly translates into excitation of α-motor neurons and muscle contraction. The induced muscle contraction will return muscle to the desired length and limb to its initial position restoring muscle spindle activity to a background level. During neurological testing the input is mostly from Ia muscle spindle afferents. Normally muscles are always under some degree of stretch, this reflex circuit mediated by group II muscle spindle afferents is responsible for the steady level of muscle tension in muscle called muscle tone. Figure 16.10 Spinal reflexes as part of the motor command The central nervous system can take advantage of the functional organisation of spinal cord to reduce processing requirements of higher centres. Gamma drive to muscle spindles allows stretch reflex contribute to muscle activity during slow contractions. Kandel Schwartz & Jessel (2000). Principles of Neural Science 4/e McGraw-Hill. Gain of the stretch reflex The gain of the stretch reflex depends on excitability of α-motor neurons sensitivity of muscle spindles regulated by γ-motor neurons The level of γ-motor neuron activity referred to as γ- bias or gain can be adjusted by upper motor neuron pathways as well by a local circuitry. The gain of the myotatic reflex refers to the amount of muscle force generated in response to a given stretch of the muscle spindle. Under various demands of voluntary and involuntary movement α and γ motor neurons are often co-activated by higher centres to prevent muscle spindles from being unloaded or overstretched/ overactivated. γ motor neuron activity can be modulated independently of α-motor neuron activity if the context of movement requires it. In general γ motor neuron activity is high if movement is relatively difficult and demands rapid and precise execution. Fusimotor set Muscle spindle sensitivity adjusted for different conditions or behavioural “states” 1) differential control of static (γs) and dynamic (γd) gamma activity 2) more complex tasks have increased γ drive to increase sensitivity “state” rest sit stand slow fast imposed paw beam walk walk mov’t shake walk γd γs Kandel Schwartz & Jessel (2000). Principles of Neural Science 4/e McGraw-Hill. Proprioception Golgi tendon organs (GTO) Golgi tendon organs are formed by branches of group Ib afferents distributed among collagen fibres that form tendons. They provide information about muscle tension. GTOs are arranged in series with a small number (10-20) of extrafusal muscle fibres. Population of afferents provide accurate sample of tension which exists in a whole muscle. Figure 9.7 Golgi tendon organs - a negative feedback system to regulate muscle tension Golgi tendon organ circuit is a negative feedback system to regulate muscle tension contacts Ib inhibitory interneurons in local circuits Golgi tendon organ circuit counteracts small changes in muscle tension by increasing or decreasing the inhibition of α-motor neurons. Golgi tendon organ control system tends to maintain a steady level of force, counteracting effects that diminish muscle force, for example, fatigue. It plays a protective role at large forces. Ib inhibitory interneurons receive modulatory synaptic inputs from various sources including upper motor neurons, joint receptors, muscle spindles and cutaneous receptors. In summary Muscle spindle system is a feedback control system that monitors and maintains muscle length and thus keeps limbs in a desired position. Golgi tendon organ system is a feedback control system that monitors and maintains muscle force. Protective reflexes Protective reflexes: flexion reflex pathways Triggered by cutaneous nociceptors (Aδ myelinated fibres) Polysynaptic pathway Excitation of ipsilateral flexors and inhibition of extensors Inhibition of contralateral flexors and excitation of extensors, thus providing postural support during withdrawal Descending pathways regulate suppression of the reflex Following damage to descending pathways and after removing inhibition other types of stimuli can trigger the flexion reflex Figure 16.14 Recurrent inhibition and Renshaw cell Identified in the 1940’s by Renshaw Renshaw cell receives monosynaptic excitatory input from motoneuron axon collaterals Renshaw cell output is monosynaptic inhibition back to homonymous and synergistic motoneurones (feedback) It is called “Recurrent inhibition” Renshaw cell-function Regulation of motoneurone firing by higher level inputs Inputs from supraspinal inputs can inhibit and the brain facilitate the Renshaw cell this is a mechanism to control how motoneurones respond to inputs (gain control) when Renshaw cell is inhibited, the motoneurone is more responsive to inputs when Renshaw cell is excited, the motoneurone is inhibited and becomes less responsive to inputs - decreased gain means - smaller output for same input Rothwell JC (1994) Control of Human Voluntary Movement. 2nd ed, Chapman and Hall, Fig 5.11 Rhythmic movements Sequence of coordinated motor actions performed in repetitive manner: locomotion, flying, breathing, swallowing and vomiting Lamprey locomotion Feline locomotion Human locomotion Why differentiate from other movements? The basic pattern for rhythmic movements is thought to be controlled by oscillating circuits within the spinal cord: CENTRAL PATTERN GENERATORs (CPGs) Signals from higher centres (supraspinal) and peripheral receptors (afferent feedback) influence the basic pattern produced by the CPG to produce a flexible functional output. Advantages of spinal control. Spinal cord circuitry and locomotion Central pattern generators (CPGs) are biological neural networks that produce rhythmic patterned outputs. CPGs don’t require sensory input to be initiated, but their activity could be modified by sensory feedback. They are fully capable of controlling the timing and coordination of complex patterns of movement and adjusting them in response to altered circumstances without coordinating input from the brain. Cats after transection of the spinal cord at thoracic level can walk on a treadmill making coordinated movements with their hind limbs. Speed of locomotor movements is determined by the speed of the treadmill, suggesting that the stretch reflex is a part of a motor program. When dorsal roots are also transected and thus there is no sensory input, locomotion can be induced by intravenous injection of L-DOPA (dopamine precursor). In humans CPGs have not been successfully activated to restore continuous natural walking pattern. Warning may be Figure 16.15 https://www.youtube.com/watch?v=sK7nKweiDro (cat) unpleasant to watch! https://www.youtube.com/watch?v=oPerfpxYJ1U (rat; CPG + spinal cord regeneration) CPGs in intact animals Central pattern generators play a broad role in all animals: besides various types of locomotion (on the ground, in the water, in the air) they might also be involved in generation of respiratory and swallowing patterns. In intact animals, spinal CPGs are activated by tonic (non-rhythmic) descending drive from the brain. The CPG produces the basic oscillating pattern. Central pattern generators show amazing variability and adaptability: Sensory information shapes the basic oscillating pattern to make the motor output appropriate for the environment. Signals from the brain can modify the motor output to meet goals or refine the CPG command. Locomotion in the leech The leech propels itself through the water by sequential contraction and relaxation of the body wall musculature of each segment. The segmental ganglia in the ventral mid line coordinate swimming, with each ganglion containing a population of limited number of neurons. Electrical recordings from the ventral (EMGV) and dorsal (EMGD) longitudinal muscles in the leech and the corresponding motor neurons show a reciprocal pattern of excitation for the dorsal and ventral muscles of a given segment. NEUR3101 Motor control Lecture 6 Brain and movement The ascending and descending tracts A/Prof Ingvars Birznieks Characteristics of voluntary movements Goal-directed Greater flexibility than reflex or rhythmic movements Reflexes and rhythmic movements can be part of voluntary movements Program Execution Primary motor cortex Supplementary motor area Premotor area Idea plan Octopus: voluntary movements vs complex movements controlled by CPGs https://www.youtube.com/embed/dxQmOR_QLfQ Ika odori don Motor cortex Primary motor cortex and premotor cortex Mediate the planning and initiation of complex temporal sequences of voluntary movements. Cortical areas receive regulatory inputs from basal ganglia and cerebellum via relays in the ventrolateral thalamus. Figure 17.2 Primary motor cortex Theodor Fritsch and Eduard Hitzig demonstrated that electrical stimulation of motor cortex in dogs elicit contraction of muscles on the contralateral side. Based on observations in progression of epileptic seizures John Hughlings Jackson proposed that motor cortex contains an organised spatial representation, or map, of the body’s musculature. Charles Sherrington published maps of the organisation SOMATOSENSORY of motor cortex in great apes. During 1930s Sherrington’s student American neurosurgeon Wilder Penfield demonstrated in great detail that human motor cortex contains a motor map of the body. His data were based on examination of 400 neurosurgical patients. The cortical homunculus is a visual representation of the concept of "the body within the brain“. Homunculus means little man. It was first drawn by an artist hired by Penfield Mrs. H. P. Cantlie. MOTOR Homunculi Primary motor cortex Finer organization assessed by cortical microstimulation and spike triggered EMG averaging revealed that structure of the maps is rather different from what was initially believed. Figure 17.6 Primary motor cortex Even smallest currents capable to illicit response excited several muscles and simultaneously inhibited others suggesting that organized movements rather than individual muscles are represented in the map. This peripheral muscle group is referred to as a muscle field. Note the difference: motor neuron pool refers to all motor neurons innervating a single muscle. Edward Evarts (NIH, USA) found in behaving animals that force generated by contracting muscles correlated with firing rate of upper motor neurons even prior to the execution of movement. Within the same body parts a particular movement could be elicited by stimulation of widely separated sites supporting argument that neurons are connected in networks to create specific movement patterns. Primary motor cortex Michael Graziano from Princeton used microstimulation varying stimulation time so that it would resemble volitional movements (hundreds of milliseconds to several seconds). Resulting movements were sequentially distributed across multiple joints and were strikingly purposeful and target oriented. Output encoded not simply the trajectory of arm motion, but also the final position of the hand: hand to mouth as if to feed (left panel) hand to central space as if to inspect an object (right panel) + starting position final position ▬ movement path Figure 17.7 Primary motor cortex Summary: Activity of upper motor neurons in the cortex controls movements rather than individual muscles. Such organisation represents dynamic and flexible means for encoding higher order movement parameters leading to the coordinated activation of multiple muscle groups across several joints to perform behaviourally useful actions. Primary motor cortex Primary motor cortex layer 5 pyramidal cells are the upper motor neurons. Betz cells are the largest neurons (by soma size) in body, but constitute only 5% of pyramidal cell projections to the spinal cord. Betz pyramidal neurons are primary responsible for skilled movements of the hands and fingers. Corticospinal and corticobulbar (to brainstem) tract axons pass through the internal capsule. 90% of pyramidal tract neurons cross midline (decussate). 10% form anterior corticospinal tract and terminate ipsilaterally or bilaterally. Mostly control axial and proximal limb muscles. Only limited number of axons has privilege to synapse directly with α-motor neurons limited to forearm and hand. Some elements of corticobulbar and corticospinal tracts originate from somatosensory cortex and terminate in dorsal horn which is mostly sensory. They are likely to be involved in modulation of proprioceptive signals. Figure 17.3&4 Pyramidal and extrapyramidal pathways Pyramidal system Corticospinal tract - connects to spinal motor neurons Lateral corticospinal tract – the major corticospinal tract Anterior = ventral = medial corticospinal tract – smaller, gradually diminishes in size as it descends, it ends about the middle of the thoracic region Corticobulbar tract - composed of the upper motor neurons of the cranial nerves Extrapyramidal system Tectospinal tract Rubrospinal tract Reticulospinal tract Vestibulospinal tract Pyramidal tracts Pyramidal tracts Corticobulbar tract connects upper motor neurons in the cortex to lower motor neurons in the motor nuclei of the cranial nerves located in the brainstem. Motor nuclei of the cranial nerves should be viewed functionally analogous to the spinal cord. However, they are located in the brainstem only because they mainly control muscles in the head. Note that lower motor neurons are located only in the nuclei of cranial nerves, other nuclei involved in higher motor control function also located in the brainstem do not have direct access to muscles and thus do not contain any lower motor neurons. The brainstem consists of the midbrain, the pons, and the medulla oblongata. Ventral and lateral corticospinal tracts Very important descending motor system in humans with approx. 1 million fibres Originates in the primary motor cortex, premotor areas, and somatosensory cortex! Consists from Lateral corticospinal tract Controls distal limb muscles Ventral / anterior corticospinal tract Controls axial (neck & back) Fig. 11.2 p 197 muscles Noback et al. (2005) The Human Nervous System. 6th Ed. Humana Press, Totowa, NJ, USA Kandel Schwartz & Jessel (2000). Principles of Neural Science 4/e McGraw-Hill. Lateral corticospinal tract ~ 90% of corticospinal fibres Crosses the midline at the pyramidal decussation. Project to local circuit neurons, and in some cases directly on the alpha and gamma motor neurons of distal muscles of upper and lower extremities - hands and feet. Humans have more monosynaptic direct connections to motor neurons than any other animals. This allows greater flexibility and fine control of digits. Small proportion of CS tract fibres (~2%) descend without decussating as the uncrossed lateral corticospinal tract (i.e. control distal muscles on the ipsilateral side) Kandel Schwartz & Jessel (2000). Principles of Neural Science 4/e McGraw-Hill. Ventral / Anterior corticospinal tract ~ 10% of corticospinal fibres Does not cross the midline at the pyramidal decussation. Fibres project bilaterally to ventro- medial spinal cord (axial muscles – neck, shoulder, trunk), meaning that they cross at the level of the target spinal segment. Extrapyramidal system Pyramidal system Tectospinal tract Pyramidal tract - coordinating head and eye - control most of our fine movements movements as part of the visual and auditory reflexes Rubrospinal tract - fractionation of fine movements activation of flexor muscles Vestibulospinal tract - controls postural muscles Reticulospinal tract - projects from the reticular formation - inhibition or facilitation of movement From : The Central Nervous System, P. Brodal - feedforward control Extrapyramidal pathways Rubrospinal Receives afferent fibres from the motor cortex and cerebellum. Regulates tone: excitatory effect on limb flexor muscles, inhibitory effect on extensor muscles. Neural activity in the red nucleus is related to force, velocity and direction of fine movements. Responsible for fractionation of movement. Fractionation is the ability to apply movement to one joint in isolation without affecting other joints. Reticulospinal Descends from reticular formation in two tracts to act on the motor neurons supplying the trunk and proximal limb muscles. Involved mainly in locomotion and predictive (feed-forward) postural control. Tectospinal Coordinates head and eye movements. Connects midbrain tectum superior colliculus with motor nuclei of cranial nerves and cervical region of the spinal cord. Mediates reflex postural movements of head in response to visual (tectum/superior colliculus) and auditory (inferior colliculus) stimuli. Vestibulospinal The nuclei relay motor commands through the vestibulospinal tract in response to vestibular input. Function of these motors commands is to alter muscle tone, extend, and change the position of the limbs and head with the goal of supporting posture and maintaining balance of the body and head. Direct projections from vestibular nuclei to the spinal cord ensure a rapid compensatory feedback response to any postural instability detected by vestibular apparatus. Summary of pyramidal and extrapyramidal pathways The pyramidal pathways accomplish skilled, discrete muscle actions. The extrapyramidal pathways provide the framework (muscle tone, posture, regulation of reflex activity). The lower motor neurons are referred to as the final common pathway. Both pathways control lower motor neurons. These lower motor neurons may receive both excitatory and inhibitory stimulation from both pathways. The sum of this input determines the final response of an individual neuron in the pathway. In case of spinal cord injury the overall dominating effect is loss of excitatory input on alpha motor neuron, loss of inhibitory input on gamma motor neuron. Spinal cord transection and spinal shock When the spinal cord is suddenly transected, essentially all its functions, including spinal cord reflexes, immediately become depressed. This is called “spinal shock”. There is complete loss of all reflexes, no muscle tone, paralysis, complete anaesthesia. The cause is sudden cessation of tonic input upon spinal cord interneuron pool which is normally present and transmitted via pyramidal and extrapyramidal tracts. Period of spinal shock is couple of days in humans. It may vary depending on the level spinal cord injury. The more complex is the animal the greater is the spinal shock period. After the spinal shock During recovery from spinal shock, the excitability of spinal cord reflexes increase due to the lack of descending inhibition and possible denervation hypersensitivity. Reflexes will reappear, but mostly exaggerated. Strong afferent stimuli from one of paralysed body parts may irradiate to other reflex centres including autonomic. Descending pathways - Tectospinal tract Cortical control of movement Part 1: Mapping the primary motor cortex Dr. Frederic von Wegner WARNING This material has been reproduced and communicated to you by or on behalf of the University of New South Wales in accordance with section 113P of the Copyright Act 1968 (Act). The material in this communication may be subject to copyright under the Act. Any further reproduction or communication of this material by you may be the subject of copyright protection under the Act. Do not remove this notice Motor pathways and networks pathway view: serial connection of two neurons: a) upper motor neuron (brain) b) lower motor neuron (spinal cord) corticospinal tract pyramidal tract (brainstem decussation) Guyton&Hall, Textbook of Medical Physiology Duus’ Topical Diagnosis in Neurology Motor pathways and networks pathway view: serial connection of two neurons: a) upper motor neuron (brain) b) lower motor neuron (spinal cord) corticospinal tract pyramidal tract (brainstem decussation) Guyton&Hall, Textbook of Medical Physiology Duus’ Topical Diagnosis in Neurology Motor pathways and networks network view, there are many loops: a) cortico-cortical b) cortex-basal ganglia c) cortico-thalamic d) cortex-cerebellar e) cortex-brainstem... Guyton&Hall, Textbook of Medical Physiology Duus’ Topical Diagnosis in Neurology Focus on voluntary movement Common structures Grillner, Physiol Rev, 2020 Current Principles of Motor Control Do you need a motor cortex to move? Answer: no Ebbesen, Nat Rev Neurosci 2017, Motor cortex, to act or not to act? The map perspective historically, many lesion studies (trauma, armed conflicts) postmortal examinations of focal lesions early brain surgery Lüders, Textbook of Epilepsy Surgery The map perspective historically, many lesion studies (trauma, armed conflicts) postmortal examinations of focal lesions early brain surgery cortex localisation movement of a body region concept: cortex = map Lüders, Textbook of Epilepsy Surgery Experimental evidence Intraoperative brain stimulation (identify functionally important regions) Presurgical diagnostics (epilepsy surgery): implantation of intracranial electrodes, option to record and stimulate electrode contacts at defined anatomical sites intracranial electrode Multiple contacts: 1…8 Lüders, Textbook of Epilepsy Surgery Cortex mapping by electrical stimulation Penfield, Brain, 1937 map-like activation characteristics Cortex mapping by electrical stimulation lateral Other experiments: map-like inhibition ventral characteristics medial Penfield (from Ebbesen, Nat Rev Neurosci, 2017 Motor cortices Guyton&Hall, Textbook of Medical Physiology Somatotopic map of the primary motor cortex Duus’ Topical Diagnosis in Neurology Guyton&Hall, Textbook of Medical Physiology Somatotopic map of the primary motor cortex Somatotopic mapping: each body region has its own representation in the brain Neighbouring body parts are neighbours in the brain The representation is distorted: body regions with higher innervation density occupy larger cortex areas https://en.wikipedia.org/wiki/Cortical_homunculus Cortical control of movement Part 2: Motor cortices – an overview Dr. Frederic von Wegner WARNING This material has been reproduced and communicated to you by or on behalf of the University of New South Wales in accordance with section 113P of the Copyright Act 1968 (Act). The material in this communication may be subject to copyright under the Act. Any further reproduction or communication of this material by you may be the subject of copyright protection under the Act. Do not remove this notice Primary motor cortex (M1) somatotopic organization (brain has a body map) encodes activation of (single muscles and) groups of muscles Guyton&Hall, Textbook of Medical Physiology Primary motor cortex (M1) somatotopic organization encodes activation of (single muscles and) groups of muscles encodes force production in these muscles, but also inhibits others (task dependent) Kandel, Principles of Neural Science Primary motor cortex (M1) somatotopic organization encodes activation of (single muscles and) groups of muscles encodes force production in these muscles, but also inhibits others encodes direction of movement Each tick = 1 action potential Kandel, Principles of Neural Science Primary motor cortex (M1) somatotopic organization encodes activation of (single muscles and) groups of muscles encodes force production in these muscles, but also inhibits others encodes direction of movement Kandel, Principles of Neural Science Primary motor cortex (M1) somatotopic organization encodes activation of (single muscles and) groups of muscles encodes force production in these muscles, but also inhibits others encodes direction of movement the population vector: characterizes the directional preference of a large group of neurons Each ray: 1 neuron, length=discharge rate, angle = preferred movement direction Blue vector: vector sum of discharge rates Dashed vector: actual arm movement Conclusion: vector sums of neuronal activities predict the actual movement Kandel, Principles of Neural Science Directional tuning of M1 neurons somatotopic organization encodes activation of (single muscles and) no load external load groups of muscles encodes force production in these muscles, but also inhibits others encodes direction of movement the population vector (many neurons) combining direction and force: the brain adjusts the activity of all motor cortex neurons to counteract an opposing external force to reach a target (error correction) Kandel, Principles of Neural Science Premotor cortex / area (PM) extended region frontal to the primary motor cortex, lateral of the SMA encoding of complex action sequences, coordination with cerebellum and basal ganglia connection with visual and afferent areas hand-related activity: grasp, manipulation coordinated eye-head movements (frontal eye field) direct connection to the spinal cord > isolated M1 lesions recover > isolated PM lesions recover > combined lesions much less Guyton&Hall, Textbook of Medical Physiology Premotor cortex / area (PM) extended region frontal to the primary motor cortex, lateral of the SMA encoding of complex action sequences, coordination with cerebellum and basal ganglia connection with visual and afferent areas hand-related activity: grasp, manipulation coordinated eye-head movements (frontal eye field) direct connection to the spinal cord > isolated M1 lesions recover > isolated PM lesions recover > combined lesions much less Supplementary motor area (SMA) frontal to M1 near the midline, medial to PM encodes bilateral movements and movements of all limbs encodes multi-muscle, multi-joint movements and sequences motor sequence rehearsing (motor imagery) Guyton&Hall, Textbook of Medical Physiology Supplementary motor area (SMA) frontal to M1 near the midline, medial to PM encodes bilateral movements and movements of all limbs encodes multi-muscle, multi-joint movements and sequences motor sequence rehearsing (motor imagery) Prefrontal cortex Prefrontal cortex makes complex movement plans: sequences of bilateral, multi-joint, multi-muscle movement patterns lying down/standing up, cycling can include articulation (sounds, speech) connection to motivational and emotional brain regions Uncontrolled activity leads to complex, potentially dangerous movements (jerking, jumping) Complex prefrontal activity (epileptic) Prefrontal cortex makes complex movement plans: sequences of bilateral, multi-joint, multi-muscle movement patterns lying down/standing up, cycling can include articulation (sounds, speech) connection to motivational and emotional brain regions Uncontrolled activity leads to complex, potentially dangerous movements (jerking, jumping) Executive functions (prefrontal cortex) Luria, Higher Cortical Functions in Man Executive functions (prefrontal cortex) repetitive task performance = perseveration characteristic symptom of prefrontal cortical lesions Luria, Higher Cortical Functions in Man Functional movement disorders emotions affect movement planning and execution very frequent disorders often: tremor, myoclonic tics/jerks, dystonia, non-epileptic seizures in elderly: phobic gait disorder neural correlates in fMRI: stronger connectivity between motor cortices and amygdala (emotional processing, fear) Baizabal-Carvallo, Neurobiol of Disease, 2019 Summary there is a system of motor cortices we discussed: primary motor cortex (M1), premotor areas (PM) and the supplementary motor area (SMA) they are connected to all other motor systems (basal ganglia, brainstem, cerebellum...) M1 encodes: activation of single muscles/small muscle groups, force, orientation PM encodes: sequential and multi-muscle activation, hand-related coordination SMA encodes: bilateral, sequential and multi-joint movements the fundamental connection volition – motor initiation remains unclear