Inorganic Chemistry II Practice Questions for Chapter 4 PDF
Document Details
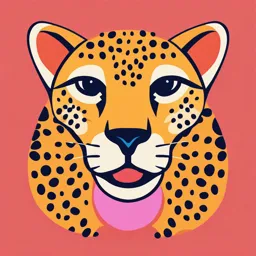
Uploaded by LoyalSteelDrums
Kent State University
null
null
Tags
Related
- Module 2 Coordination Compounds and Organometallics Lecture Notes PDF
- Coordination Compound PDF
- Supramolecular Chemistry Cation-Binding Hosts PDF
- Inorganic Chemistry II Practice Questions for Chapter 4 PDF
- Week 10 Crystal Field Theory Workbook - Monash S2 2024 PDF
- Metal Complexes and Organometallics - Winter Semester 2024-2025 PDF
Summary
This document contains practice questions regarding coordination complexes and organometallic compounds. The questions explore the differences between these two types of compounds and go on to evaluate examples with various structures. It discusses valence electrons and the 18 electron rule.
Full Transcript
**Inorganic Chemistry II -- CHEM-40302-001** Practice Questions for Chapter 4 ================================ 1. What are the major distinctions between coordination complexes and organometallic compounds? Ans: Coordination complexes are normally charged, have variable d-electron count, and...
**Inorganic Chemistry II -- CHEM-40302-001** Practice Questions for Chapter 4 ================================ 1. What are the major distinctions between coordination complexes and organometallic compounds? Ans: Coordination complexes are normally charged, have variable d-electron count, and are soluble in water, while organometallic compounds are often neutral, have fixed d electron count (18 or 16 valence electrons), and are soluble in organic solvents (e.g. THF = tetrahydrofuran). In other words, most organometallic compounds have properties that are much closer to organic compounds than inorganic salts. For example, many of organometallic compounds have low melting points. Some are liquid at room temperature. 2. Do (a) \[IrBr~2~(CH~3~)(CO)(PPh~3~)~2~\] and (b) \[Cr(η^5^-C~5~H~5~)(η^6^-C~6~H~6~)\] obey the 18 electron rule? Ans: There are two different methods for counting valence electrons: neutral-ligand method and donor-pair method. For these two questions, the neutral-ligand method gives results more quickly. (a) The Ir atom (Group 9) has nine valence electrons, both Br atoms and the CH~3~ group are the X-type ligands, and each of them is considered one-electron donor. CO and PPh~3~ are the L-type ligands, and each of them is considered two-electron donor. Thus, the number of valence electrons on the metal atom is 9 + (3×1) + (3×2) = 18\. (b) The Cr atom (Group 6) has six valence electrons, the η5-C~5~H~5~ ligand donates five electrons, and the η6-C~6~H~6~ ligand donates six, so the number of metal valence electrons is 6 + 5 + 6 = 17. This complex does not obey the 18-electron rule, and is not stable. 3\. Assign the oxidation number and count the valence electrons on the metal atom in (a) \[IrBr~2~(CH~3~)(CO) (PPh~3~)~2~\], (b) \[Cr(η^5^-C~5~H~5~)(η^6^-C~6~H~6~)\], and (c) \[Mn(CO)~5~\]^-^. Ans: When oxidation number needs to be assigned, the donor-pair method is better. In this method, ligands are considered to donate electrons in pairs, resulting in the need to treat some ligands as neutral and others as charged. \(a) We treat the two Br groups and the CH~3~ as three singly negatively charged two electron donors and the CO and the two PPh~3~ ligands as three two-electron donors, providing 12 electrons in all. Because the complex is neutral overall, the Group 9 Ir atom must have a charge of +3 (that is, have oxidation number +3) to balance the charge of the three anionic ligands, and thus contributes 9 - 3 = 6 electrons. This analysis gives a total of 18 electrons for the Ir(III) complex. (b) We treat the η^5^-C~5~H~5~ ligand as C~5~H~5~^-^ and thus it donates six electrons, with the η^6^-C~6~H~6~ ligand donating a further six. To maintain neutrality, the Group 6 Cr atom must have a charge of +1 (and an oxidation number of +1) and contributes 6 - 1 = 5 electrons. The total number of metal electrons is 12 + 5 = 17 for a Cr(I) complex. This complex does not obey the 18-electron rule and is unlikely to be stable. (c) We treat each CO ligand as neutral and contributing two electrons, giving 10 electrons. The overall charge of the complex is -1; because all the ligands are neutral, we consider this charge to reside formally on the metal atom, giving it an oxidation number -1. The Group 7 Mn atom thus contributes 7 +1 electrons, giving a total of 18 for a Mn(-1) complex. Ans: Two requirements for the formation of this and the other similar complexes are: \(1) strong-field ligands (which most of the organometallic ligands discussed in Chapter 22 are), and (2) a d^8^ metal ion. The complexes fulfilling these two requirements will be stable and adopt square planar geometry. For the purposes of counting valence electrons in this complex, the donor-pair method should be used in order to reveal the oxidation number (O.N.) of the metal center. First, the Pt atom (from Group 10) has 10 valence electrons, but when we assign O.N. for the Pt center is +2 because three Cl^-^ ions contribute 3- charges, and the complex itself has 1- overall charge, thus this metal ion has O.N. = +2, which makes the valence electron count for Pt^2+^ become d^8^. Since we use the donor-pair method to count electrons for the ligands, the three Cl^-^ ions and ethane molecule are all 2-electron donors. Therefore, the total number of valence electrons on the metal center is 8 + (4×2) = 16. The geometry of the Pt center is square planer (if you view the dash line as the fourth bond). 5. A quick perusal of Table 22.1 in the lecture notes reveals that Group 9 and Group 10 metals can form stable complexes with either 18- or 16- valence electrons. Consult the textbook or any reference book and design a similar 16-electron complex with one of these metals rather than platinum. Comment on the electron count of the metal center and of the total valence electrons. Predict the molecular geometry of the complex you have just designed. Ans: A quite large number of complexes in this class could be (and have been) synthesized, but if you had designed the following complex before 1970's, it could have won you the fame (i.e. the Nobel prize in chemistry) and/or fortune. Since this was already done by a chemist named Sir Geoffrey Wilkinson, the only thing left for you to do now is to provide valence electron count for this complex and give a plausible explanation to its molecular geometry. 6. Dihydrogen (H~2~) can act as a neutral 2-electron donor ligand to form organometallic complexes containing this molecule as least in formality. Provide a brief description to the bonding interactions in such complexes, and if necessary, sketch proper diagrams of orbital interactions to illustrate the bonding features. Ans: The bonding of dihydrogen to the metal atom is made up of two components: (1) a σ donation of the two electrons in the H-H bond to the metal atom, and (2) a π backdonation from the metal to the σ\* antibonding orbital of H2. As the π backbonding from the metal atom increases, the strength of the H-H bond decreases and the structure tends to that of a dihydride: 7. Why is the following reaction referred to as the oxidative addition? What valence electron count is required to the metal center to readily undergo such process, and what is the oxidation number of the Ir center before and after the reaction. Ans: Simply put, the metal center is oxidized with an increase in the O.N. from +1 to +3, while the dihydrogen (H~2~) is added into the metal center as two separate hydride ligands. Increase. To be able to readily undergo such a reqction, the valence electron count of the metal center needs to be 16, and then becomes 18 after the reaction. The O.N. = +1 before the reaction, and the O.N. = +3 after the reaction. 8. Without consulting a textbook, use your own words to describe the bonding interactions in metal carbonyls based on the following MO diagram of CO. Ans: The lone pair on the carbon atom acts as a Lewis σ base (i.e. an electron-pair donor) to donate electron density to an empty d orbital of the metal center (left), while and the empty CO antibonding orbital (i.e. the 2π energy level in the above diagram which consists of two antibonding orbital) acts as a Lewis π acid (i.e. an electron-pair acceptor) to accept the back-bonding electron density of an filled or partially filled d orbital of the metal center (right). The overall effect is that the stronger the metal-carbon bond becomes, the weaker the CO bond becomes. In the extreme case, when two electrons are fully donated by the metal center to the p-antibonding orbital of CO, a formal metalcarbon double bond is formed, resulting in a decrease in the bond order of the CO to 2. In practice, the bonding is somewhere between M-CΞO, with no back-bonding, and M=C=O, with complete back-bonding. 9. Rank the following compounds in the order of *decreasing* CO IR stretching frequencies, and provide a plausible explanation for your ranking order: (a) \[Cr(CO)~6~\]; (b) \[V(CO)~6~\]^-^, (c) \[Mn(CO)~6~\]^+^, and (d) \[Ti(CO)~6~\]^2-^. Ans: The order of *decreasing* CO IR stretching frequencies is \[Mn(CO)~6~\]^+^ \> \[Cr(CO)~6~\] \> \[V(CO)~6~\]^-^ \> \[Ti(CO)~6~\]^2-^ , which indicates the bond strength of the triple bond in the CO ligands is decreasing. The plausible explanation is that the -back-bondingg from the metal center to the CO ligands increases from \[Mn(CO)~6~\]^+^ to \[Ti(CO)~6~\]^2-^. Since all these complexes obey the 18-electron rule with the d6 configuration for the metal center, the overall charge determines the degree of metal to CO back-bonding. 10. Do the following 3d metal carbonyl complexes obey the 18-electron rule: (a) Cr(CO)~6~, (b) Mn~2~(CO)~10~, (c) Fe(CO)~5~, (d) Co~2~(CO)~8~, and (e) Ni(CO)~4~? Ans: Yes, they all do as shown in the following table (you should practice counting valence electrons in each of these).