Introduction To The Central Nervous System PDF
Document Details
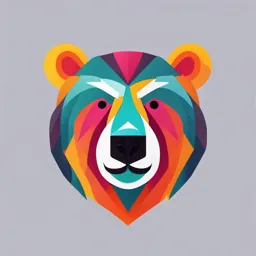
Uploaded by UnrivaledZeugma
Tags
Related
- Introduction And Organization Of Nervous System Part I PDF
- Unit 3 - Organisation of the Nervous System: CNS & PNS (Structure and Functions) PDF
- Neuroanatomy Peripheral Nervous System PDF
- The Nervous System PDF
- Biology 1: Basics of the Nervous System: Neuroanatomy (University of Nottingham) PDF
- Nervous System Physiology 2024 PDF
Summary
This document provides an introduction to the central nervous system, covering key concepts such as glial cells, neurons, and action potentials. It explores the role of astrocytes in maintaining the extracellular environment and regulating blood flow. The document also details the structure of neurons, including cell bodies, dendrites, axons, and axon terminals, as well as the process of axonal transport.
Full Transcript
**Introduction to the Central Nervous System\_W16:** **Learning Outcomes:** 1. **The role of the astrocyte type of glial cells** 2. **The main proteins and structures involved in axonal transport, including dynein, kinesis and tubulin** 3. **The characteristic of a "generic" action potent...
**Introduction to the Central Nervous System\_W16:** **Learning Outcomes:** 1. **The role of the astrocyte type of glial cells** 2. **The main proteins and structures involved in axonal transport, including dynein, kinesis and tubulin** 3. **The characteristic of a "generic" action potential (controlled by voltage-gated sodium channels, and the resulting release of neurotransmitter from the axonal terminal.** 4. **The definitions of the terminology including: presynaptic, postsynaptic, vesicles, exocytosis, and the synaptic cleft.** **GLIAL CELLS:\ \ There are multiple glial cell types, including:** **\ Central glial cells:** 1. **Microglia:** have phagocytic, immune response functions. 2. **Oligodendrocytes:** myelinate axons of the central nervous system 3. **Astrocytes,** explored in more detail below. **Peripheral glial cells:** 1. **Schwann cells:** myelinate peripheral axons. 2. **Satellite cells:** maintain extracellular environment, support injury/inflammation responses. **ASTROCYTES:** Astrocytes are the most common type of glial cell found in the brain and spinal cord. They play a critical role in **maintaining the extracellular environment**, managing the levels of potassium, sodium, and calcium around neurons. Astrocytes **regulate blood flow** to certain regions of the brain and have a role in the uptake and release of neurotransmitters. They support the formation and function of synapses by maintaining the environment around synapses and **regulating the removal of neurotransmitters from the synaptic cleft.** Astrocytes are involved in information processing and can influence synaptic transmission and neuronal communication. **THE NEURON:** Each neuron has a basic set of features. These features include: 1. **Cell body (soma)** contains the nucleus and other organelles, including nucleolus, mitochondria, endoplasmic reticulum and Golgi apparatus. Acts as the metabolic centre of the neuron. 2. **Dendrites:** branched extensions from the cell body that receive signals from other neurons which conduct electrical impulses towards the cell body. Dendrites are typically covered with dendritic spines, which are small protrusions that form synapses with axons of other neurons. 3. **Axons:** long, singular projection that transmits electrical impulses away from the cell body to other neurons, muscles or glands. Can vary in length from a few mm to over a meter long. The axon hillock is the region where the axon originates from the cell body and is crucial for initiating action potentials.\ \ Note that some axons may collateralise into tens or hundreds of thousands of axon terminals. Collateral projections, or axon collaterals, are secondary branches that come off the main axon of a neuron. These projections enable a single neuron to send signals to multiple target neurons or muscle cells. This branching allows for more complex and widespread communication within the nervous system. 4. **Myelin sheath:** a fatty substance that insulates the axon, increasing the speed of electrical signal transmission. Formed by the oligodendrocytes in the CNS and Schwann cells in the peripheral nervous system (PNS). Myelinated sections are interspersed with Nodes of Ranvier which are gaps in the myelin sheath where ion channels are concentrated. 5. **Axon terminals (also called synaptic buttons):** are the distal endings of the axon that form synapses with other neurons or effector cells. Axon terminals are responsible for releasing neurotransmitters into the synaptic cleft and contain synaptic vesicles filled with neurotransmitters. 6. **Synapse:** the synapse is the junction between two neurons or between a neuron and its target cell (e.g. muscle or gland). A synapse allows for the transfer of information through chemical or electrical signals. The synapse includes the components of the pre-synaptic membrane (axon terminal), synaptic cleft (space between the neurons) and postsynaptic membrane (dendrite or cell body of the receiving neuron).  Recall that a basic definition of an **action potential** is a rapid rise and fall in voltage across a cellular membrane, initiated at the axon hillock and propagated along the axon, which is the primary method of electrical signalling neurons. A single cell is a **neuron**. In medical terms, a **nerve** is a bundle of many axons, containing contributions from many neurons. A neuron, by itself, is pretty useless. Neurons can only carry their functions out when they form connections with other neurons. The nervous system contributes to 25% of the Total Metabolic Rate (TMR) and relies upon many energy-requiring processes including axonal transport. **AXONAL TRANSPORT:** Axonal transport is the process by which proteins, organelles, and other materials are moved along the axon of a neuron. This transport is crucial for the maintenance, function, and survival of neurons, as it ensures that necessary materials are delivered to the correct locations within the cell. **Types of Fast Axonal Transport:** 1. **Anterograde Transport:** Moves cargo including synaptic vesicles, neurotransmitters, mitochondria, lipids, proteins and components needed for axonal growth and maintenance **from the cell body (soma) toward the axon terminal** using **kinesin as the primary motor protein.** **KINESIN PROTEINS:** have heavy chains and light chains. Each kinesin molecule has two heavy chains that form a coiled-coil structure. **The heavy chains contain motor domains (also called "heads") at their N-terminus.** These motor domains have ATPase activity, which means they can hydrolyze ATP to provide the energy needed for movement. The motor domains bind to the microtubules and "walk" along them. This walking involves the heads alternately attaching to and detaching from the microtubule, powered by the hydrolysis of ATP.Each motor domain moves in a hand-over-hand manner, enabling the kinesin molecule to travel along the microtubule. When one head binds to the microtubule and undergoes a conformational change, it moves the other head forward. **The light chains** are associated with the heavy chains and are located at the C-terminus of the kinesin molecule. The light chains are primarily responsible for binding to the cargo that needs to be transported. This cargo can include synaptic vesicles, mitochondria, proteins, and other organelles or molecules required at the axon terminal. By attaching to the cargo, the light chains ensure that the kinesin molecule can transport specific materials along the microtubules to their intended destination within the neuron. **In a process, step-by-step format:** 1. The heavy chains' motor domains bind to the microtubules. The binding and release of these motor domains are regulated by ATP hydrolysis. 2. When a motor domain binds ATP, it undergoes a conformational change that propels the kinesin forward as the hydrolysis of ATP to ADP releases energy, allowing the motor domain to detach and reattach further along the microtubule. 2. **Retrograde Transport:** Moves cargo including endocytosed materials, signalling endosomes, damaged organelles and neurotrophic factors (e.g. growth factors that are taken up at the synapse and transported back to the soma for signalling purposes). from the axon terminal back toward the cell body. Uses **Dynein** as the primary motor protein responsible for retrograde transport. **\ **Dynein uses a slightly different mechanism than Kinesin to attach to microtubules but nonetheless uses Heavy chains and Light chains. **In a process, step-by-step format:** 1. The heavy chains' motor domains bind to the microtubules. The binding and release of these motor domains are regulated by ATP hydrolysis. 2. When a motor domain binds ATP, it undergoes a conformational change that moves the dynein molecule along the microtubule. 3. The hydrolysis of ATP to ADP releases energy, allowing the motor domain to detach and reattach further along the microtubule. This cycle of binding, conformational change, and release enables the dynein to "walk" along the microtubule in a processive manner, moving the cargo toward the minus (-) end of the microtubule, which is oriented towards the cell body. Drugs which affect microtubule assembly and disassembly often have peripheral polyneuropathy as a side effect. **Colchicine** -- binds tubulin, which makes it unavailable for microtubule assembly. Is used to treat gout because gout is a distal buildup of crystals at the site of inflammation. **Vincristine**/**vinblastine** -- aggregates tubulin, resulting in premature depolymerisation. Taxol -- stabilises microtubules, prevents depolymerisation. **FAST AXONAL TRANSPORT IS IMPLICATED IN NUMEROUS DISEASES:** Toxins or pathogens are endocytosed at the axon terminal and take into the neuron. In the case of the Tetanus toxin, the toxin is retrogradely transported to the soma of the infected neuron. The tetanus toxin will also cross the membrane and reach inhibitory interneurons that project onto the alpha motor neurons. Consequently, there is continual contraction of the muscles that are innervated by the alpha motor neurons. This occurs because the toxin **disinhibits** the alpha motor neurons by affecting the inhibitory interneurons. Axon transport may be impaired in a number of degenerative diseases, such as Alzheimer\'s disease, Huntington\'s disease, motor neuron disease, or amyotrophic lateral sclerosis, Parkinson\'s disease, etc. In these diseases, impairment of axonal transport may result in vesicles and large proteins being bound up at the axon hillock and never proceeding down the axon hillock. **PART \#2 INTRO TO CNS\_W16:** **NEUROTRANSMISSION:** Neurons project information onto their target via chemical or chemo-electric modes of neurotransmission. This involves neurotransmitters, whether peptides or amino acids, are bound in vesicles stored within the axon terminal.  **THREE TYPES OF NEURONS:** **\*** A **dendritic arbor** refers to the complex, branching structure of dendrites in a neuron. Dendrites are the extensions of a neuron that receive signals from other neurons. The dendritic arborization increases the surface area available for synaptic connections, allowing a single neuron to integrate a large amount of information from various sources. The extent and pattern of the dendritic arbor can vary greatly between different types of neurons and is crucial for the neuron's role in processing information within the nervous system. \***Neurites** are the projections or extensions from the cell body of a neuron, which include both axons and dendrites. These structures are critical for the transmission of electrical and chemical signals in the nervous system. **BIPOLAR NEURON:** Cell body located between the dendritic arbor and the axon terminals. Commonly observed as sensory receptors. Bipolar neurons are often found in sensory systems, such as the retina of the eye, the olfactory epithelium, and the vestibulocochlear nerve for hearing and balance. Their structure allows them to efficiently relay sensory information from the peripheral sensory organs to the central nervous system. **\ MULTIPOLAR NEURON:** An axon arises out of the cell body, followed by many dendritic projections. **\ PSEUDOUNIPOLAR NEURON:** Unlike typical neurons with multiple distinct dendrites and a single axon, pseudounipolar neurons have a **single process** that extends from the cell body. This single process bifurcates (splits) into two branches: **Peripheral Branch:** Extends to the peripheral tissues, acting similarly to a dendrite in that it receives sensory input. **Central Branch:** Extends to the central nervous system (CNS), transmitting sensory information from the peripheral branch to the spinal cord or brainstem. **NEURONS CAN FULFIL TWO MAJOR FUNCTIONS:** 1. **Projection Neurons:** have long axons relative to soma size, may be myelinated and are usually excitatory (in the CNS). 2. **Interneuron:** generally have shorter axons and may be excitatory or inhibitory (usually inhibitory) in the CNS. **NEUROTRANSMITTER SYSTEMS:** Neurotransmitters are defined as compounds released by pre-synaptic cells which affect the firing or behaviour of downstream tissues, including neurons, glia, muscles, secretory organs, etc. There are over 100 compounds identified as neurotransmitters in humans. Neurotransmitters can act on ion channels directly or through 2^nd^ messenger systems. The activity of neurotransmitters is regulated by complex, activity dependent mechanisms (e.g. pre-synaptic auto receptors, destructive enzymes, reuptake, diffusion, etc). **Vesicles in a Single Nerve Terminus:** Each vesicle typically contains only one type of neurotransmitter. For instance, a vesicle might contain either glutamate, GABA, or dopamine, but not a mix of these. However, in a single neuronal axon terminal, multiple types of vesicles can be present, each type storing a different neurotransmitter. This allows a neuron to have the capacity to release different neurotransmitters depending on the situation. For example, a neuron might have vesicles containing both an excitatory neurotransmitter (like glutamate) and an inhibitory neurotransmitter (like GABA). A single neuron can release more than one type of neurotransmitter or neuromodulator. This is achieved through the release of different vesicles that co-localize within the same nerve terminal. **Examples of neurotransmitters include:** GABA, ATP, glutamate, serotonin, adrenaline, noradrenaline, and acetylcholine. **Modulators:** Besides classical neurotransmitters, neurons can also release neuromodulators---substances that modulate the effect of neurotransmitters. These can include neuropeptides, hormones, or other signalling molecules such as CCK, or opioid transmitters such as enkephalin. **REVIEW OF THE ACTION POTENTIAL:** **1. Resting Membrane Potential** **2. Threshold is eventually reached** **3. Depolarization (Rising Phase)** **4. Peak of Action Potential** **5. Repolarization (Falling Phase)** **6. Hyperpolarization (After-Hyperpolarization Phase)** **7. Refractory Period** **Absolute Refractory Period:** **Relative Refractory Period:** **8. Return to Resting Potential** **THE REFRACTORY PERIOD** is a limiting factor on the rate of action potential firing. All neurons have a downstream target (a muscle, another neuron, an endocrine cell, etc). **COMMUNICATION BETWEEN NEURONS:** ***The synapse***  **CHEMICAL SYNAPSE:** release of neurotransmitter from pre-synaptic terminal activates a post-synaptic receptor. This is going to be the focus of our learning. **\ \ ** **ELECTRICAL SYNAPSE:** transmission of ions and small molecules occurs via channels/pores formed by **connexin proteins**, meaning that cell connected by electrical synapses are virtually the same cell. Connexin proteins are a very fast way to pass information from one cell to another. ***Release of neurotransmitter from the cell*** An action potential will cause the membrane to depolarise. Calcium will initiate release of transmitter via SNARE and SNAP proteins that allow the vesicles to bind to the membrane. The membrane then exocytosis transmitter into the synaptic cleft. **NEURAL PLASTICITY: Preservation of information about the history of neuronal activity can employ some or all of the following mechanisms:** -Making the synaptic cleft more narrow will reduce distance between where the transmitter is released and available receptors. -Increasing the number of post-synaptic receptors, increasing the number of targets and making the post-synaptic cell more sensitive to the transmitter that is released. -Increasing current flow through ion channels (post-synaptic) -Increasing the area of the post-synaptic density -Changes in spine density -Facilitation of transmitter release (pre-synaptic) -Increasing (mobilising) transmitter receptor population **GRADED POTENTIALS:** Graded potentials are changes in the membrane potential of a neuron that vary in size, as opposed to the all-or-none nature of action potentials. They play an important role in the initial stages of neuronal signalling and in the integration of synaptic inputs. Characteristics of graded potentials: 1. **Variable Amplitude:** The amplitude (size) of a graded potential is directly proportional to the strength of the stimulus. A stronger stimulus results in a larger change in membrane potential. 2. **Decremental Conduction:** Graded potentials decrease in amplitude as they travel away from the site of origin. This decremental spread occurs because the current leaks out of the membrane as it travels, reducing the signal strength over distance. 3. **Summation:** Graded potentials can sum together through spatial and temporal summation. **Spatial Summation:** When multiple graded potentials from different locations on the neuron add together. **Temporal Summation:** When multiple graded potentials occur in quick succession at the same location, they can add together.  The probability of firing an action potential for a given cell depends on the timing and location of excitatory and inhibitory presynaptic -temporal summation and spatial summation. If the individual inputs are too small or they\'re too widely spaced in time or too widely spaced along the dendritic arbor to produce a threshold response, there will not be an action potential in the postsynaptic cell. 4. **Local Changes:** Graded potentials occur locally, usually in the dendrites and cell body (soma) of the neuron. They do not travel long distances like action potentials. 5. **Types of Graded Potentials:** **Excitatory Postsynaptic Potentials (EPSPs):** Depolarising graded potentials that make the neuron more likely to fire an action potential by bringing the membrane potential closer to the threshold. **Inhibitory Postsynaptic Potentials (IPSPs):** Hyperpolarising graded potentials that make the neuron less likely to fire an action potential by moving the membrane potential further from the threshold. **Mechanism of Graded Potentials** 1. **Initiation:** Graded potentials are initiated by the opening of ligand-gated ion channels in response to neurotransmitter binding at synapses. For instance, the binding of a neurotransmitter to its receptor can open Na+ channels (causing depolarization) or Cl- channels (causing hyperpolarization). 2. **Ion Movement:** When ligand-gated ion channels open, ions move across the membrane according to their electrochemical gradients. This movement changes the local membrane potential, creating a graded potential. 3. **Amplitude and Duration:** The magnitude of the graded potential depends on the number of ion channels opened and the duration they remain open. A greater stimulus results in a larger graded potential. **Function of Graded Potentials** 1. **Signal Integration:** Neurons receive multiple synaptic inputs, which can be excitatory or inhibitory. Graded potentials allow the neuron to integrate these inputs and determine whether to fire an action potential. **This integration occurs at the axon hillock, where the cumulative effect of all graded potentials is assessed.** 2. **Threshold Determination:** If the sum of the graded potentials at the axon hillock depolarizes the membrane to the threshold level (around -55 mV), voltage-gated sodium channels open, and an action potential is initiated. If the combined graded potentials do not reach the threshold, the neuron will not fire an action potential. 3. **Modulation of Neural Activity:** Graded potentials provide a way for neurons to finely tune their activity. The varying sizes of graded potentials allow for a more nuanced response to different stimuli, compared to the all-or-none nature of action potentials.  In the case of presynaptic inputs that are located close to the soma, they\'re very likely to affect the firing because they\'re very close to the cell body and very close to the axon hillock. B, which is the excitatory postsynaptic potential or input that is further away from the cell body, is likely to have less of an influence compared to A1 or A2. This is called spatial integration or spatial summation. An **excitatory postsynaptic potential**, which occurs at the same site, A1, and then another one, which occurs at A2, or very closely located in time within, say, a half a millisecond or within the duration of a single action potential, **will produce a larger response together than either one of them would produce in isolation**. This is **temporal summation.** **TYPES OF SYNAPSES:** **We can classify synapses based on their locations on the post-synaptic cell.** **AXODENDRITIC SYNAPSES:** **Location:** Between the axon terminal of the presynaptic neuron and the dendrite of the postsynaptic neuron. These synapses often occur on the dendritic shafts or spines of the postsynaptic neuron. **Function: Excitatory Synapses:** Axo-dendritic synapses are typically excitatory, meaning they often cause depolarization of the postsynaptic membrane, increasing the likelihood of an action potential. **Signal Integration:** Dendrites integrate signals from multiple axo-dendritic synapses, allowing the neuron to process a vast amount of synaptic input. This integration is crucial for the spatial summation of excitatory postsynaptic potentials (EPSPs). **Plasticity:** These synapses exhibit significant plasticity, meaning their strength can change based on activity, which is essential for learning and memory **AND;** **\ \ Signal Strength:** Because they are located on dendrites, the signal strength can diminish as it travels toward the soma, but they can still significantly influence the neuron's overall activity through summation. **AXOSOMATIC SYNAPSES:** **Location:** Between the axon terminal of the presynaptic neuron and the soma (cell body) of the postsynaptic neuron. **Function: Influence on Firing:** Axo-somatic synapses have a **strong influence on the postsynaptic neuron's ability to fire an action potential because they are located close to the axon hillock**, where action potentials are initiated. **Inhibitory Synapses:** These synapses are often inhibitory, releasing neurotransmitters like GABA that hyperpolarize the postsynaptic membrane, thereby decreasing the likelihood of an action potential. **CHARACTERISTICS:** **Impact:** Due to their proximity to the axon hillock, axo-somatic synapses can powerfully modulate the neuron's output, effectively controlling whether the neuron will fire or not. **Efficiency:** The signals from axo-somatic synapses reach the axon hillock with less decrement compared to signals from axo-dendritic synapses, making them more efficient in influencing the neuron's activity. **AXO-AXONIC SYNAPSES** **Connection:** Between the axon terminal of the presynaptic neuron and the axon terminal or axon hillock of the postsynaptic neuron. **Functions:** **Modulating Transmitter Release:** Axo-axonic synapses modulate the release of neurotransmitters from the postsynaptic axon terminal. They can either enhance or inhibit this release through mechanisms such as presynaptic inhibition or facilitation. **Specific Regulation:** By acting directly on the axon terminal, axo-axonic synapses can precisely control the amount of neurotransmitter released in response to an action potential. Because of spatial exclusion, there are only a small number of axo-axonic inputs that can be available. Usually, these are inhibitory inputs. **Fine-Tuning:** These synapses provide fine-tuning of synaptic transmission, allowing for the precise regulation of neural circuits. **Complex Control:** Axo-axonic synapses can control the timing and extent of neurotransmitter release, impacting the strength and timing of the synaptic signal received by the post-synaptic neuron. **UPSTREAM NEURONS** fire action potentials which propagate to the neuronal axonal terminal where they initiate transmitter release from the pre-synaptic membrane via exocytosis. **DOWNSTREAM NEURONS** receive action potentials from pre-synaptic cells as post-synaptic potentials. Whether the downstream neuron fires an action potential after receiving an input from the presynaptic cell depends on the type, location, and amplitude of the postsynaptic potential and on past firing history. **CIRCUITS AND FEEDBACK:** Feedback is a mechanism for regulating input at each level of a neural circuit. Feedback projections can be excitatory, inhibitory, or both. Feedback can operate at a single cell level, and across long-distance connections (e.g. between cortical areas).  **FEEDBACK CIRCUITS:** A neural circuit where the output of a neuron or a group of neurons loops back to influence their own activity or the activity of preceding neurons. **FEED-FORWARD CIRCUITS:** A neural circuit where the signal travels in one direction from the input to the output, without looping back. **LATERAL INHIBITION CIRCUITS:** A neural mechanism in which an excited neuron reduces the activity of its neighbours. This process enhances the contrast and sharpness of sensory perception. **TWO PATTERNS OF INFORMATION PROPAGATION:** A diagram of a diagram Description automatically generated **Convergence** is the process by which multiple neurons send their inputs to a single neuron. Convergence allows a single neuron to integrate multiple signals from various sources. This integration is crucial for complex processing tasks such as decision-making, sensory integration, and motor control. The postsynaptic neuron can summate excitatory and inhibitory inputs from different presynaptic neurons. This summation can be both spatial (inputs from multiple locations) and temporal (inputs over time). Convergence can increase the sensitivity of the postsynaptic neuron to weak signals, as the combined input from multiple neurons can reach the threshold for action potential generation. Convergence also provides redundancy, ensuring that the failure of a single input does not completely disrupt the processing of information. **EXAMPLES OF BIOLOGICAL CONVERGENCE:** **Sensory Systems:** In the visual system, multiple photoreceptors (rods and cones) converge onto a single bipolar cell. This convergence allows the bipolar cell to integrate visual information from a broader area of the retina. **Motor Control:** In the spinal cord, multiple sensory neurons and interneurons converge onto motor neurons. This convergence enables motor neurons to integrate various sensory inputs and coordinate muscle contractions. **SEE THE CONVERGENCE BELOW:** **DIVERGENCE:** The process by which a single neuron sends its output to multiple neurons. Divergence allows a single neuron to distribute its signal to various targets, facilitating the simultaneous transmission of information to multiple parts of the nervous system. A single action potential in the presynaptic neuron can influence multiple postsynaptic neurons, amplifying the signal. Divergence allows for **efficient distribution of information**, enabling a single neuron to impact multiple downstream targets. Divergence supports **parallel** **processing**, where different aspects of the same information are processed simultaneously by different neurons or neural circuits. **Examples:** **Sensory Processing:** In the visual system, a single retinal ganglion cell can diverge its output to multiple neurons in the brain, such as those in the lateral geniculate nucleus (LGN) and the superior colliculus. This divergence allows parallel processing of visual information in different brain regions. **Motor Coordination:** A single motor neuron can send branches to multiple muscle fibres, ensuring coordinated muscle contractions. **OVERVIEW OF BRAIN STRUCTURE:** **Cortex =** laminated layered structure of grey matter containing a lot of neurons. Has six layers, each with distinct functions and patterns of cell bodies. Nissl stain is used to identify layers by staining cell bodies. Layer 1 has few cell bodies. Different layers have specialised functions: layer 4 receives input from the thalamus and other sub-cortical structures, especially from the thalamus, layers 2 and 3 receive inputs from other cortical areas, Layer 5 sends outputs to subcortical structures and the spinal cord and Layer 6 sends outputs back to the thalamus and can influence inputs.  All of these cortical functional areas project to each other and they all project to an area called the superior colliculus in the midbrain, which controls movement of the eyes. This movement may include directing the eyes toward the source of a sound or predicting the trajectory of a prey. **At every point of streams, there is crosstalk, and the final pathway,** which is formed onto the superior colliculus, gets information from both streams. **Electroencephalographs take EEGs:** Just follow along in time as we go from left to right. You can see that activity starts off fairly quiet at the surface in all of these electrodes. But then you start to see a buildup or a recurrent pattern of activation in one part of the brain. So the electrodes overlying, in this case, the right temporal lobe. And as you see this pattern of repetitive activity build up, it starts to get larger and larger and larger until we get a takeoff or a paroxysm and cells begin to fire uncontrollably. And that is a seizure. That\'s what happens when the brain becomes what\'s called hyper-synchronized or hyper-excitable. These cortical neurons fire without inhibition and this can be terrifying to watch, but it can also be lethal. 