ICU cEEG Monitoring PDF
Document Details
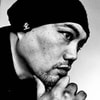
Uploaded by DignifiedHarpy8046
Orange Coast College
2020
Walt Banoczi
Tags
Summary
This article discusses the use of continuous electroencephalography (EEG) monitoring in intensive care units (ICUs). It examines the benefits of cEEG, including seizure detection, assessment of coma, and determination of brain death. The article also reviews indications, technical aspects, and current practices, including the use of QEEG for managing large amounts of data from critical care monitoring.
Full Transcript
The Neurodiagnostic Journal, 60: 231–271, 2020 © 2020 ASET – The Neurodiagnostic Society ISSN: 2164-6821 print / 2375-8627 online DOI: https://doi.org/10.1080/21646821.2020.1824982 ICU-cEEG Monitoring Walt Banoczi, R. EEG/EP T., CNIM, CLTM ES, BVE, FASET...
The Neurodiagnostic Journal, 60: 231–271, 2020 © 2020 ASET – The Neurodiagnostic Society ISSN: 2164-6821 print / 2375-8627 online DOI: https://doi.org/10.1080/21646821.2020.1824982 ICU-cEEG Monitoring Walt Banoczi, R. EEG/EP T., CNIM, CLTM ES, BVE, FASET Professor Emeritus Orange Coast College Costa Mesa, California ABSTRACT. The neurological application of long-term electroencepha lography (EEG) monitoring in the intensive care unit (ICU) has been imple mented in many healthcare institutions. The use of EEG as a monitoring tool in the ICU affords many potential benefits. Uses include the identification of seizures, vasospasm following subarachnoid hemorrhage (SAH), the assess ment of coma and the determination of brain death. Neurologic critical care is focused on recognition and treatment of secondary insults. Often treatment is withheld because these insults are not recognized early enough until an irreversible deficit manifest. Continuous EEG (cEEG) monitoring provides a unique potential to recognize these insults and offers an opportunity for early intervention. Why should we continuously monitor the brain with EEG in the ICU? Nonconvulsive seizures (NCS) are common in comatose patients. Nonconvulsive Status Epilepticus (NCSE) and NCS1 are damaging to brain tissue; thus, rapid control of seizures is essential to preserving brain function. With the increased use of cEEG in critical care areas, the purpose of this paper is to examine the use and benefits of EEG monitoring of ICU patients, review the indications for the use of cEEG and discuss technical issues and concerns when performing cEEG monitoring. This article has been divided into six distinct sections: (1) Seizures, NCS, and NCSE (2) Periodic Discharges2 and Patterns on the Ictal-interictal Continuum, (3) Cerebral Ischemia, SAH, and Delayed Cerebral Ischemia (DCI), (4) Encephalopathy and Coma (5) ECI and Brain Death, and (6) ICU-cEEG Monitoring Techniques. KEY WORDS. Delayed cerebral ischemia, EEG ICU monitoring, neuro-critical care, nonconvulsive seizures, vasospasm. Corresponding Author’s E-mail: [email protected] Received: April 24, 2020. Accepted for publication: September 15, 2020. 231 232 ICU-CEEG MONITORING INTRODUCTION Electroencephalography (EEG) offers the ability to measure brain function in a noninvasive way and in real time. EEG testing benefits patient care in many clinical settings and has many advantages over other testing modalities. Benefits include: l) identification of new neurologic complications in time to intervene and alter out come; 2) identification of new systemic complications allowing prompt interven tion; 3) assessment of prognosis for survival or neurologic recovery; 4) monitoring and regulating therapy, providing feedback regarding its adequacy; and 5) clinical correlations with EEG abnormalities that are well-recognized in the literature. Other advantages of EEG monitoring include portability of monitoring in a variety of locations. In addition, monitoring can be done continuously, even in comatose patients, and does not involve radiation or any other risks associated with invasive procedures. Furthermore, EEG monitoring is quantifiable, which adds numerical precision. (Nuwer 1991). Almost since the first use of EEG in the outpatient setting to help diagnose epilepsies, EEG has also been used in more acute settings in hospitals. With the development of intensive care units (ICU) units dedicated to the resuscitation of patients with cardiac arrest; to treating patients with single or multi-organ system failure; to treating patients with burns; and to managing post-operative surgical patients, the use of EEG in the ICU continues to expand. Hill et al. (2018) in their article “Continuous EEG is Associated with Favorable Hospitalization Outcomes for Critically Ill Patients” reported that continuous EEG (cEEG) was associated with lower mortality in the hospital. Their survey indicated results from 2004 to 2013 that in 7,102,399 critically ill patients, 22,728 received cEEG monitoring. Over this study period only 13,576 of patients with a diagnosis of seizures/ status epilepticus, 2,953 of patients with a diagnosis of SAH or intracerebral hemorrhage and 12,393 patients with a diagnosis of altered consciousness were monitored with cEEG. Mortality in the EEG-monitored cohort was 22.8% vs 27.8% in the cohort without cEEG. The significance of cEEG in the ICU includes (1) detection of nonconvulsive status epilepticus (NCSE) in patients with unexplained mental deterioration (2) assessment of sedative/anesthetic state (3) early detection of delayed ischemia due to subarachnoid hemorrhage (SAH) and (4) assessment of the outcome of patients with post-resuscitation encephalopathy (Kubota et al. 2018). As an expansion of traditional ICUs, there are now neurological and other specialized ICU settings, and the EEG has seen increasing use to help diagnose causes of encephalopathy, coma and unresponsiveness in these units. cEEG has also been used to uncover nonconvulsive seizures (NCS) and NCSE; to provide prognosis after cardiorespiratory arrest in the Cardiac Intensive Care Unit; and to explain unexpected slow recoveries after surgery in the Surgical Intensive Care Unit. These uses have led to increased recognition of occult encephalopathies, NCSE and various coma patterns. ICU-CEEG MONITORING 233 This article will address the challenges of recognizing patterns of encephalopathy, coma and seizures, present recording techniques and best practices, and provide prognostic information on some of the specific EEG patterns that may be seen. Monitoring the brain in real time allows the neurophysiologist/intensivist/neurologist, trained and experienced in clinical EEG and specifically in cEEG (ACNS - The American Clinical Neurophysiology Society 2016a), to make timely changes in treatment that affect the outcome of the patient. QEEG is an excellent tool for efficiently managing the huge amounts of data generated in LTM. QEEG ICU cEEG trends can be used to assist with review of large amounts of data generated by critical care cEEG monitoring. QEEG provides a compressed and simplified view of the raw EEG signals, potentially allowing for evaluation by non-neurophysiologists. The primary goal is to alert the interpreting physician to periods of concern. The raw EEG from the periods of concern can confirm significant events. This could potentially impact patient outcomes by resulting in faster seizure identification and treatment because it has been shown that NCS of longer duration are associated with worse outcomes. Various applications of QEEG in critically ill patients include NCS detection, ischemia detection, vasospasm/delayed cerebral ischemia (DCI) detection, prognosis after cardiac arrest, mon itoring depth of sedation, and monitoring depth of therapeutic burst suppression (LaRoche and Haider 2018). QEEG is sensitive in detecting changes in cerebral blood flow like those seen in vasospasm and delayed cerebral ischemia (DCI) (Figures 1–4). Various QEEG trend preferences include seizure detection, rhythmicity spectrogram, density spectral array (DSA), asymmetry index/spectrogram, alpha/delta ratio (ADR), and relative alpha varia bility (RAV) trends (ASET–The Neurodiagnostic Society 2013; Swisher and Sinha 2016). INDICATIONS FOR CEEG MONITORING Seizures, NCS, and NCSE An electrographic seizure is a sudden, abnormal EEG event defined by a repetitive and evolving pattern with a minimum 2 µV voltage and duration of at least 10 seconds. “Evolving” is defined as an unequivocal evolution in frequency, voltage, morphology or location. Seizure burden can be quantified for clinical purposes using one of two defini tions. Frequency is the number of seizures per hour, or percent of the record with seizures. Another measure is the total summed duration of all the seizures divided by the entire duration of an epoch of interest, or temporal-spatial quantification. Epileptic seizures can occur in a wide variety of situations and with various clinical manifestations. EEG testing is the most useful ICU procedure for determining whether a patient is suffering from seizures and whether the seizures are focal or generalized. This information helps guide the choice of medications most effective for the patient’s seizures. 234 ICU-CEEG MONITORING FIG. 1. (a) 60-year-old female, status-post basilar tip aneurysm clipping. Trend indicates some variability but not normal. (b) For comparison, this is a normal QEEG trend. Note the symmetry and variability over time. ICU-CEEG MONITORING 235 FIG. 2. Following CT scan, trend shows diminished variability and percent alpha at F3. (This is the same patient as 1a). FIG. 3. Patient taken for endovascular therapy and arterial papaverine is administered. (This is the same patient as 1a). 236 ICU-CEEG MONITORING FIG. 4. Trend shows increase in background alpha activity. The patient is clinically more alert and awake. (This is the same patient as 1a). EEG monitoring also can reveal NCS or NCSE. Such subtle degrees of epileptic seizures are a cause of decreased level of consciousness in neurological ICU patients. In more recent large, multi-center studies, 8–68% (median 38%) of 2020 patients in the ICU of 11 facilities presented with seizures during the monitoring (Leppik 2012). In many such patients, decreased level of consciousness is the only outward clinical manifestation of the seizure. Kerrigan (2009) reported in, “EEG in the ICU: Rhythmical and Periodic Patterns,” that 18% of selected “at risk” adult neuro ICU cases had seizures. He also reported that in adult “at risk” patients without a prior history of seizures, 12% presented with NCS and 8% with NCSE (Figure 5). Convulsive seizures usually are obvious to the observer. Occasionally, there is confusion between the patient with epileptic seizures and the patient who is periodi cally moving or jerking for other reasons. Convulsive seizures are difficult to identify or diagnose clinically in patients who are on neuromuscular junction blocking agents because there may be depressed or no subtle clinical signs of the seizure. In these patients, EEG monitoring may provide the only means of detecting the existence of seizures (Banoczi 1995; Leppik 2012) (Figure 6). Drs. LaRoche and Haider stated in their book “Handbook of ICU EEG Monitoring” (2018, pg. 360–361) that “outcome studies for nonconvulsive seizures in the ICU suggest an urgency for diagnosis and treatment that exceed many current standard practices. The standard current practice for reporting is 2–3 times per day ICU-CEEG MONITORING 237 FIG. 5. NCSE characterized by continuous, high amplitude, frontally dominant 2 to 3 HZ dis charges. Reprinted with the permission of ASET-The Neurodiagnostic Society. FIG. 6. This 8-hour total power trend demonstrates several spikes in the waveforms consistent with generalized NCS. 238 ICU-CEEG MONITORING with more frequent review in patients with active seizures. Patients that have had less than 10 hours of NCSE have been associated with an eventual discharge home. Seizures lasting 10–20 hours of NCSE were associated with permanent disability. Seizure activity demonstrating NCSE for greater than 20 hours was associated with a high mortality rate.” ICU seizures are often nonconvulsive and prolonged NCS and NCSE directly increase morbidity and mortality (Hirsch 2004). In critically ill patients with persis tently abnormal mental state following successful treatment of generalized convulsive status epilepticus, NCS & NCSE may still persist. Thus, one of the main purposes of ICU-cEEG monitoring is the diagnosis of NCS and NCSE. NCS is also commonly seen in conditions such as traumatic brain injury (TBI), SAH and intracerebral hemorrhage. When a patient is clinically observed to be having clonic, myoclonic or tonic clonic seizures, the diagnosis is usually not in doubt and an EEG may reflect whether there is any focality to these generalized epileptic seizures. The major challenge, however, comes in the identification of nonconvulsive seizures. cEEG performed on patients in the ICU may detect seizures and status epilepticus in the absence of clinical signs (Hirsch 2004; Kaplan 2006). Unexplained unconsciousness, sometimes accom panied by subtle myoclonus of the face, mouth, orbits, fingers or other parts of the body, episodes of increased tone, head deviation, eye deviation and mutism are all common clinical correlates of nonconvulsive seizures (LaRoche and Haider 2018). According to Kubota et al. (2018, 8) in their article, “Continuous EEG Monitoring in ICU,” “Traumatic Brain Injury (TBI) is associated with a risk of subsequent NCSE, which is being increasingly recognized as harmful. In a retrospective study of TBI patients undergoing cEEG, he found that 18% of patients experienced a seizure during the cEEG monitoring, all of which were subclinical seizures, while 8% developed NCSE.” Critical care continuous EEG should be reviewed as often as logistically and technically feasible and interpreted by neurophysiologist/intensivist/neurologist at least twice daily (i.e., about every 12 hours). If frequent NCS or NCSE are identified, more frequent interpretation should be provided until seizures are controlled. If clinical events are recorded, cEEG should be interpreted as soon as possible after the event to determine whether the events are ictal or nonictal (LaRoche and Haider 2018). Periodic Discharges and Patterns on the Ictal-interictal Continuum The presence of PDs has been associated with poor neurological outcomes and generalized PDs strongly associated with NCS and NCSE (Claassen et al. 2006; Foreman et al. 2012). Therefore, it is important for the Neurodiagnostic Technologist to be knowledgeable of and recognize these patterns. ICU-CEEG MONITORING 239 Periodic discharges (PDs) are stereotyped epileptiform discharges that occur at regularly spaced intervals. They may be spikes, polyspikes, sharp waves, sharply contoured slow waves or a mixture of spikes and slow waves. Typically, they are of high amplitude (100–300 µV) and occur at intervals of 0.3 second to several seconds. Repetition of the waveform patterns may be almost periodic (pseudo-periodic) (Kaplan 2006; LaRoche and Haider 2018). Various types of periodic EEG activity have been described in the neurophysiology literature, including lateralized, bilateral independent and generalized. Lateralized periodic discharges (LPDs) are discharges seen unilaterally occurring periodically or pseudo-periodically. This pattern is thought to arise typically from structural or focal lesions, most frequently ischemic strokes, but may also arise from abscesses, intracranial hemorrhages, brain tumors and focal encephalopathies. This pattern occurs transiently, typically between 2 days and 20 days, and may often co- occur with seizures. LPDs often appear acutely in the setting of recent seizure activity surrounding a structural abnormality. The prognosis, consequently, depends on the underlying etiology. In critical care EEG terminology, modifiers are often used to address additional features of an EEG pattern. The plus (+) modifier represents additional features that make an EEG pattern “more ictal-appearing.” LPDs+ is a rare pattern that appears to be associated with rapid, rhythmic, predominantly frontal discharges and seizures, and is also believed to be a transitional pattern. Rhythmic buildup of LPDs+ is thought to precede seizures. (Hirsch and Brenner 2010). Bilateral independent periodic discharges (BIPDs)3 usually arise from more morbid conditions and hence have a worse prognosis. They have been described after anoxic encephalopathy (28%), central nervous system infections (28%) and with chronic seizure disorders (22%). Almost three-quarters of the patients are comatose when this pattern is seen, and mortality has been reported at 61%. BIPDs are also highly associated with seizures (Kaplan 2006). Generalized periodic discharges (GPDs) have been described in a variety of con ditions, most recently with anoxic-ischemic coma and metabolic encephalopathies. They have also been described with Creutzfeldt-Jakob disease (CJD), subacute sclerosing panencephalitis (SSPE) and herpes simplex encephalitis. Some forms of benzodiazepine- responsive generalized nonconvulsive status epilepticus may assume a GPD form. In the final stages of the progression of convulsive status epilepticus, the EEG may also appear as GPDs. This pattern appears as a periodic sharp, spike, or slow wave often with intervening periods with variable background activity. GPDs typically occur at frequen cies slower than 1 Hz. The prognosis is generally poor but depends on the underlying etiology. When seen with anoxia, CJD and SSPE, the prognosis is usually poor, but with metabolic or toxic encephalopathies or with seizures, there may be a good prognosis. Typically, as the coma deepens, the interval between periodic discharges increases, and the amplitude falls (LaRoche and Haider 2018). 240 ICU-CEEG MONITORING There has been some controversy with regard to whether LPDs, BIPDs and other periodic patterns represent interictal phenomena, a form of “cortical irritability” or signify a more worrisome active ictal discharge with increased neuronal death, increased cerebral profusion and a rise in metabolic consumption. There is no universal consensus, and opinions vary. Most experts believe that LPDs and BIPDs represent interictal discharges, particularly when there is no clinical correlate and when they occur at intervals of every several seconds or more. However, when the discharge frequency varies, increases to faster than one per second and particularly when associated with motor correlates, then they may represent seizure activity (Kaplan 2006; LaRoche and Haider 2018). There are some additional EEG patterns that may be observed in patients being monitored in the ICU. Some of these are considered ictal, some nonictal and some are considered controversial. The term “ictal-interictal continuum” refers to the presence of abnormal periodic activity on scalp EEG variably associated with seizures (Kalamangalam and Phlmann-Eden 2018). This activity is characterized by periodic and/or rhythmic EEG patterns that occur with relatively high frequency in critically ill patients. Further understanding of the “ictal-interictal continuum” is likely to signifi cantly improve the care of the critically ill neurological patient (Hirsch and Brenner 2010; LaRoche and Haider 2018). Patterns included on this continuum are any lateralized rhythmic delta activity (LRDA).4 Brief Ictal/Interictal Rhythmic/Repetitive Discharges [B(I)RDs] is a transient EEG pattern that consists of evolving rhythmic patterns of electrical activity that share many characteristics with seizures but are very brief, with a duration of less than 10 seconds (Yoo et al. 2014). BIRDs are usually seen in the context of an abnormal EEG background and/or confirmed electrographic seizures. The presence of Extreme Delta Brush, which can follow a pattern of well- characterized electroclinical seizures is associated with seizures and status epilepticus in some patients. There appears to be a correlation with N-Methyl-D-Aspartate receptor (NMDAR) encephalitis (Schmitt et al. 2012). NMDA is an amino acid that mimics the neurotransmitter glutamate. NMDAR is a glutamate receptor found in nerve cells. Anti-NMDAR encephalitis can cause among other symptoms rapidly progressive seizures. The severity of the symptoms often requires intensive care monitoring and IV ASDs for refractory status epilepticus (Schutt-Ainé 2018). Stimulus-Induced Rhythmic or Ictal Discharges (SIRPIDs) are a relatively common phenomenon found on prolonged EEG monitoring that captures state changes and stimulation of critically ill patients. Common causes include hypoxic injury, traumatic brain injury and hemorrhage, as well as toxic-metabolic disturbances. Some studies have shown an association between SIRPIDs and the presence of spontaneous electrographic seizures. However, functional imaging (i.e., SPECT) studies have not shown an increase in cerebral blood flow to suggest an active ictal process. Patients with SIRPIDs do not seem to improve with antiepileptic medications. Treatment of SIRPIDs should follow ICU-CEEG MONITORING 241 treatment practices for the underlying pattern rather than aggressive treatment as if for seizures (Johnson et al. 2018). Cerebral Ischemia, Subarachnoid Hemorrhage (SAH), and Delayed Cerebral Ischemia (DCI) Vasospasm is a primary source of neurologic co-morbidity after SAH. The inci dence of vasospasm after SAH is 8–68% with a median of 38% (Leppik 2012). Methods like Transcranial Doppler (TCD) for detecting vasospasm and guiding therapy methods are not used continuously. In contrast, cEEG ICU monitoring can keep track of cerebral activity continuously. When monitoring patients with SAH, Nuwer (1991) observed a marked reduction in relative alpha variability during vasospasm and an increase in EEG variability as the vasospasm resolved. Persistently poor EEG variability and reactivity seemed to be predictors of a poor neurologic outcome after SAH. In SAH patients with quantitative EEG (QEEG) ICU monitoring, we found that decreased relative alpha variability is a marker for altered cerebral function accompanying vasospasm. This QEEG pattern was often present prior to documentation of vasospasm using other clinical means. “Critical care cEEG is suggested as an adjunct method to identify ischemia in at risk patients. EEG monitoring should be continued until the window for vasospasm has passed (day 14) or the patient is considered no longer at risk for vasospasm. The optimal duration of monitoring for ischemia in other patient groups has not been established and should be individualized for the specific clinical situation. A practical guide would be to continue cEEG during the highest risk window for ischemia (e.g., 24–48 hours in a patient with crescendo transient ischemic attack or 24 hours after carotid endarterectomy). The cEEG should be reviewed at least three times daily, whereas for patients at risk for acute ischemic stroke, more frequent review may be necessary, especially while the patient is asleep and clinical symptoms/signs may not be noted” (Herman et al. 2015a, 91–92) (Figure 7). It has been shown that specific pathologic or physiologic EEG patterns do allow accurate prediction of either a poor or good outcome of patients with postanoxic encephalopathy following cardiac arrest, provided that the patterns occurred recently in relation to time since cardiac arrest. Deep coma in the first hours after cardiac arrest does not necessarily preclude full functional recovery, and improvement of brain function is possible even if the EEG is initially isoelectric (Kaplan 2006). In cases of unconsciousness after cardiac arrest, lack of clinical improvement after several days is associated with a poor prognosis if the patient is off sedation. Similarly, neurologic prognosis is particularly good if recovery toward continuous, physiologic EEG rhythms occurs within 12 hours. However, absence of relevant improvement within 24 hours, with persistent isoelectric, low voltage or burst suppression with identical burst patterns is invariably associated with a poor outcome (van Putten & Jofmeijer 2016). 242 ICU-CEEG MONITORING FIG. 7. This 8-hour percent alpha trend demonstrates right frontal ischemia. The EEG signal is the closest available measure of brain function and is exquisitely sensitive to ischemia. EEG monitoring can disclose changes suggestive of impending ischemia before infarction occurs. Studies on patients with aneurysmal SAH provide some evidence that it can be successfully applied to detection of DCI with a good sensitivity (Gaspard 2016). The EEG is also sensitive to almost any disturbance of brain activity due to metabolic disorders, sedation, intoxication, increased ICP, temperature or ischemia, and can potentially be used to monitor cerebral function and perfusion. cEEG creates a window of opportunity during which an intervention can be administered to prevent irreversible injury (Gaspard 2016; van Putten and Jofmeijer 2016). Some studies suggest that cEEG with relatively simple QEEG analysis has good to incredibly good sensitivity with fair to good specificity for early DCI in a broad group of patients with SAH of various severities. Because most studies find that changes in QEEG measures often precede clinical and radiological changes, and that some QEEG (and clinical) changes can be reversed, they also confirm that a window of opportunity exists during which interventions can be implemented before irrever sible brain injury occurs (Gaspard 2016). DCI is the most common and disabling complication among patients admitted to the hospital for aneurysmal SAH and has been reported to occur in 30–40% of these patients. Clinical and radiographic methods often fail to detect DCI early enough to avert irreversible injury. The technique of ICU-CEEG MONITORING 243 inspecting variability of RAV EEG trends is often evaluated in this patient population. An ADR trend has also been effective in predicting DCI: an ADR decrease of 10% below baseline lasting 6 consecutive hours (100% sensitive and 76% specific) or an ADR decrease of at least 50% below baseline lasting 2 or more hours (89% sensitive and 84% specific). The emergence of periodic epileptiform discharges is reported as a potential indicator of ischemia. PDs and electrographic status epilepticus have also been found to be significant predictors of poor subsequent clinical outcome (Muniz et al. 2016). ICU-cEEG monitoring can be exciting when you as a Neurodiagnostic technol ogist discover QEEG trend changes during vasospasm in patients following SAH, often before the TCD is abnormal and/or clinical signs occur, and therefore have direct influence on improving patient outcome by recognizing the changes and notifying the interpreting physician to take appropriate measures. Encephalopathy and Coma Kaplan (2006, 84) observed that “the EEG contains clues that are helpful in determining the nature of an encephalopathy or coma. It also provides clues regarding the patient’s prognosis or response to therapy. Slowing of the EEG background activity is the usual pattern associated with impaired cerebral function. Slowing can either be continuous or intermittent. Patients who have structural or anoxic damage produce continuous slowing on the EEG. Anoxic damage produces continuous gen eralized slowing seen diffusely over the scalp. Structural deficits produce continuous focal slowing seen maximally over the damaged area.” The EEG can help to predict outcome in several neurologic conditions, although it is unclear whether prolonged monitoring is superior to briefer EEG recordings performed at specific times after brain injury. In addition, most EEG parameters used to predict good outcome have a fairly high false-positive rate (i.e., EEG shows favorable pattern, but patient still has poor clinical outcome). Unfavorable prognostic factors include isoelectric pattern, burst suppression pattern, periodic patterns and electrographic seizures. Favorable prognostic features include background continuity, spontaneous variability, reactivity to stimulation and presence of normal sleep patterns. Clinical populations in which EEG may aid in prognosis include severe traumatic brain injury, hypoxic ischemic encephalopathy after cardiac arrest (with or without therapeutic hypothermia) and sub arachnoid hemorrhage (Herman et al. 2015a). “Patients with a metabolic encephalopathy generally show intermittent general ized slowing. The degree of EEG slow activity, whether continuous or intermittent, corresponds in a general way with the degree of decrease in the patient’s level of consciousness. Long-term assessment of EEG background activity can offer an objective physiologic method to assess changes over time and response to any therapeutic treatment” (Kaplan 2006, 84–85). With toxic and metabolic dysfunction, 244 ICU-CEEG MONITORING the EEG changes typically mirror the descending level of consciousness. With moderate to deep coma, patterns may resemble those of sleep in that the picture is dominated by diffuse delta activity, but even in these states there fails to be reactivity to external stimuli with no appearance of lighter sleep architecture such as sleep spindles and vertex sharp waves. Most of the patterns of toxic and metabolic dis turbance are nonspecific in that these disturbances tend to slow background rhythms and produce variable degrees of intrusion of slower theta-delta frequencies. Some patterns may be somewhat suggestive, i.e., high voltage beta activity with benzodia zepine or barbiturate intoxication; Particular patterns have been identified that may have some prognostic significance such as alpha coma patterns, spindle coma pat terns, burst suppression patterns, marked bilateral suppression coma and some types of triphasic waves (TWs) (Hirsch and Brenner 2010; Kaplan 2006) (Figure 8). Kaplan (2006) also stated that polymorphic delta activity (PDA), unless due to a toxic or metabolic disturbance, typically indicates a poor outcome for the patient. A very low voltage, persistent and unreactive PDA in some conditions can be a poor prognostic sign. Such patterns may be seen with severe central nervous system (CNS) infections, after cerebral ischemic and anoxic insults, and following severe diffuse closed head trauma. Intermittent Rhythmic Delta Activity (IRDA) is seen predominantly over anterior head regions in adults. This pattern typically occurs with nonspecific encephalopa thies. There is usually some impairment of consciousness. This pattern may be seen nonspecifically in the elderly. When background activity is present and there is reactivity, the prognosis is good and shows occipital runs of IRDA without structural cause. According to Kaplan (2006, 86), “triphasic waves consist typically of three FIG. 8. Triphasic waves. Reprinted with the permission of ASET-The Neurodiagnostic Society. ICU-CEEG MONITORING 245 phases. The largest phase is surface positive, and it may be preceded and followed by smaller amplitude, negative waves. Nonetheless, many of the morphologies in typical patterns of ‘triphasic wave encephalopathy’ may be biphasic with surface positivity of the initial phase of the biphasic waveform. Occasionally, there may be phase reversals in the fronto-central region. The frequency of these repeating patterns is 1–2 Hz, and they may vary from appearing as single TWs occasionally during the recording, to pairs, triples, brief runs of the activity or all the way to a continuous pattern. TWs may be present in NCS, NCSE or in moderate metabolic coma. When background activity is present and when reactivity to external stimuli can be demonstrated by the technol ogist stimulating the patient, the prognosis is usually good.” Kaplan (2006, 93–94) stated that “there are several patterns that predict a very poor, if any recovery. These patterns are caused by multiple etiologies and are seen in severe and deep coma, and usually represent diffuse brain damage involving cortical and subcortical regions. The prognostic outcome is usually death or a persistent vegetative state.” Alpha coma pattern that signals a poor outcome is one that presents with diffuse alpha frequencies usually unreactive to noxious stimuli even when the Neurodiagnostic technologist manually opens the patient’s eyes or even when they apply painful stimulation. This pattern is often distributed over the anterior head regions and often of relatively low voltage. The usual etiology for this alpha pattern is anoxia after cardiorespiratory arrest. The pattern is usually seen shortly after the onset of coma in adults, and often evolves to other patterns such as low voltage, unreactive or burst suppression patterns. Alpha coma is a sign of poor prognosis for any meaningful neurological recovery. With anoxia secondary to structural abnorm alities of the reticular activating system, and with some head injuries prognosis is more guarded (Kaplan 2006) (Figure 9). FIG. 9. Alpha coma pattern. Note that alpha frequency activity is seen in all the electrodes and is highest in the frontal electrodes. Reprinted with the permission of ASET-The Neurodiagnostic Society. 246 ICU-CEEG MONITORING Beta coma pattern is typically seen in patients with excess or overdoses of benzodiazepines or barbiturates. The most common EEG pattern seen with these drugs is high-frequency beta activity often present in the fronto-central regions. This beta activity is usually less reactive but is also occasionally seen with spindle- like bursts (in the beta range). This pattern generally carries a good prognosis. The presence of high amplitude, diffuse fast activity seen in the EEG of a patient with unexplained coma in the ICU, may suggest an overdose of sedative medications. A burst-suppression pattern is usually seen with anoxic encephalopathy after cardiorespiratory arrest, with toxic CNS depressant drugs, with hypothermia, or is a natural consequence of anesthesia. When used for prognostic purposes, burst- suppression EEG pattern carries a grave prognosis when associated with anoxia or diffuse brain injury if the patient is off sedative drugs (99% do not return to consciousness) (Kaplan 2006). If the patient is on sedation, it may be a normal finding. As noted, the prognosis depends on the etiology. In the above pattern, high amplitude mixed frequency bursts lasting for several seconds are interrupted by flattening of the EEG. The bursts usually occur uniformly across the entire scalp. Anesthesia, sedative overdoses and deep hypothermia can also produce burst- suppression EEG without carrying a poor prognosis (Kaplan 2006) (Figure 10). “A benefit to inducing a pentobarbital coma in a patient is that it reduces ICP by lowering cerebral metabolic demands. The procedure involves the EEG being mon itored. The EEG is used to guide the titration of the barbiturate. The desired EEG pattern is achieved when the nurse gives enough of the barbiturate to achieve and maintain a burst-suppression pattern. It is desirable to maintain an interburst interval in the EEG of between 4 to 10 seconds” (Kaplan 2006, 95–96). FIG. 10. EEG shows burst suppression pattern. ICU-CEEG MONITORING 247 Increasingly common use of prolonged EEG monitoring is to follow the progres sion of treatment in comatose patients. With suppression of status epilepticus, 30% of patients may be refractory to first-line antiseizure drugs (ASDs) even for periods of up to 2 hours warranting the use of anesthetic agents such as midazolam, propofol and/or pentobarbital. The EEG may then be used as a monitoring tool to follow the treatment effect with first-line ASDs (Kaplan 2006). For patients with refractory status epilepticus, cEEG should be used to monitor the efficacy of continuous IV ASDs such as midazolam, propofol or pentobarbital, for seizure suppression, burst suppression or complete sup pression. In conjunction with the clinical examination, to assess level of consciousness in patients requiring IV sedation or pharmacologically induced coma (Kaplan 2006). Herman stated in her article “Consensus Statement on Continuous EEG in Critically ill Adults and Children, part I: Personnel, Technical Specifications, and Clinical Practice” (2015a, 87), that “postanoxic coma after cardiac arrest is one of the most serious acute cerebral conditions and a frequent cause of admission to critical care units. Given substantial improvement of outcome over the recent years, a reliable and timely assessment of clinical evolution and prognosis is essential. In addition to the classic neurologic examination, EEG is increasingly emerging as an important tool to assess cerebral functions noninvasively. Although targeted temperature manage ment and related sedation may delay clinical assessment, EEG provides accurate prognostic information in the early phase of coma.” “Early prognostication of coma after cardiac arrest represents one of the most important challenges in the intensive care unit. The generalized anoxia, ischemia and the subsequent reperfusion after cardiac arrest lead to postanoxic coma in the majority of survivors, where the complete loss of awareness of the environment and the impairment of arousal affects the clinical exploration of cerebral integrity. It is important for Neurodiagnostic Technologists working with these patients to assess and document brainstem reflex examination (i.e., pupillary response) and motor responses to painful stimuli.” (Juan et al. 2015, 465). In the context of postanoxic coma, the most frequently used features to describe EEG signals can be categorized into four main areas: background activity (continuity, symmetry), posterior dominant “alpha rhythm,” reactivity to stimuli, state changes and variability (Juan et al. 2015). Continuity refers to the regularity of the cerebral activity along the duration of the recording.5 Symmetry refers to essentially equal amplitude and frequency between hemispheres and homologous head regions. A marked asymmetry is present if the amplitude is less than 50% and/or the frequency is less than 1 Hz when comparing one hemisphere to another. Reactivity refers to any reproducible change in amplitude or frequency in the EEG signal, related to patient stimulation. The technologist should test and document reactivity as yes, no, or unclear/unknown/not applicable in the test record. Strength and/or nature of stimulus should also be noted as well as the patient reactivity to external stimulations such as noise, eye opening and pain. State changes can occur following stimulation of the 248 ICU-CEEG MONITORING patient or spontaneously while cycling through sleep-related patterns such as sleep spindles and K-complexes. Marked reduction in relative alpha variability is observed during ischemia caused by vasospasm. Increased EEG variability is present as vasos pasm resolves (Hirsch and Brenner 2010; Juan et al. 2015). Despite the effect of lowering body temperature and the sedation associated with therapeutic hypothermia, some EEG patterns during therapeutic hypothermia are already indicative of prognosis. Absent EEG background reactivity and the presence of epileptiform activity seem to be common predictors of poor outcome. Characterization of benign or severe EEG patterns early after cardiac arrest, and even during hypothermia, already provide accurate information concerning outcome. However, to be cautious with early recordings, it is recommended to wait at least 9–12 hours after cardiac arrest to make a determination (Juan et al. 2015). In summary, encephalopathy and coma patterns are frequently encountered in ICUs. These patterns are typically seen in lethargic or obtunded patients from a variety of causes varying from the irreversible to the totally reversible cerebral insult. Generally speaking, ischemic stroke and anoxic ischemia after cardiorespira tory arrest are almost completely irreversible insults. Brain injury produced by head trauma, subdural hemorrhage and some intracranial hemorrhage, in the absence of raised ICP, may be partially, moderately or occasionally, wholly reversible. Finally, electrical disturbances with seizures and status epilepticus, metabolic and some toxic encephalopathies may be totally reversible, carrying with them a good prognosis to return to baseline state. Clearly, the underlying disease state of the patient may carry the principal determining prognosis (Kaplan 2006). Electrocerebral Inactivity (ECI) and Brain Death EEG is commonly used in the ICU for the determination of brain death. In such a setting, EEG can help the clinician make this difficult distinction. Electrocerebral inactivity (ECI) is defined as a record where no EEG activity exceeds 2 µV (ACNS - The American Clinical Neurophysiology Society 2016b; Banoczi 1995). When the EEG shows ECI, in the absence of significant hypothermia (> 90° Fahrenheit or 32.2° degrees Celsius) or large amounts of anesthetic medications, the patient is considered to have suffered an irreversible loss of brain function. This pattern has the worst prognosis, i.e., zero recovery. The American Clinical Neurophysiology Society Guideline 6: Minimum Technical Standards for EEG Recording in Suspected Cerebral Death defines ECI and gives guidelines to be followed when recording EEG in patients suspected of brain death. Some recommendations include using a complete complement of 10–20 electrodes with impedances below 10,000 ohms, montages of electrodes at least 10 cm apart, increasing the sensitivity to 2 µV/mm for at least 30 minutes of the recording, using appropriate filter settings, testing reactivity to intense stimuli (somatosensory, ICU-CEEG MONITORING 249 auditory or visual) and additionally, documenting all medications the patient is currently taking, as well as the patient’s current blood pressure, oxygen saturation and core body temperature. ICU-CEEG MONITORING TECHNIQUES Many technical and strategic lessons can be learned about ICU EEG long-term monitoring from existing literature and from practices used in several other areas of clinical EEG application. These include lessons learned from operating room (OR) monitoring (i.e., intraoperative neurophysiological monitoring or IONM), quantified EEG analysis (i.e., QEEG), routine EEG in the ICU and from long-term monitoring in epilepsy (i.e., LTME). Many of these valuable lessons are directly applicable to ICU long-term cEEG monitoring (Banoczi 1995). Lessons Taken from IONM EEG intraoperative monitoring has a similar goal to monitoring in the ICU. The principal goal is rapid identification of new complications in time to intervene, thus lessen or avoid neurologic deficits to the patient. In the OR and in the ICU, EEG monitoring needs to be specific for the particular patient’s circumstances. Each patient serves as his or her own control, thus establishing his or her own baseline. In both the OR and the ICU, some EEG changes may be abrupt and dramatic, others are slow and gradual, either of which can be a sign of neurologic complications requiring intervention. As described in Chiappa and Hoch (1993), these EEG changes can include an increase in EEG slowing and/or loss of fast activity, asymmetries, epileptiform activity or loss of the entire signal. It is important for Neurodiagnostic technologists to pay attention to these changes, document changes on the record and notify appropriate personnel of the critical test findings. The technologist should also document who they notified and record the time of notification. When such adverse changes occur, the clinical and recording circumstances need to be examined care fully. Sometimes centrally active medications are responsible for abrupt changes, especially sedative medications administered in boluses and the technologist should document the drug and dosage. As Drs. Hirsch and Brenner (2010) state in their book “Atlas of EEG in Critical Care,” sedatives, particularly benzodiazepines and barbiturates, cause slowing as well as excess fast activity, primarily in the beta range. At higher levels, these can cause burst suppression followed by ECI. The burst suppression pattern with barbiturates tends to have bursts of higher amplitude than with benzodiazepines; propofol is probably in between these two in that regard. High doses of almost any centrally acting medication can cause diffuse slowing. Some tend to cause GPDs as well, most notoriously baclofen and lithium. 250 ICU-CEEG MONITORING “In the ICU, some changes are due to ordinary physiologic variability such as the sleep-wake cycle. Non-neurologic changes, such as the presence of scalp swel ling following craniotomy, can also affect the results of EEG monitoring. In the OR, EEG has become a standard monitoring procedure during carotid endarterectomy. Carotid clamping causes EEG changes resulting from a regional cerebral blood flow (rCBF) drop below 18 ml/100 gm/min. Since this rCBF value is near the critical threshold for cerebral impairment, EEG can serve as a valid and valuable monitor. When major ischemic EEG changes occur for 30 minutes, patients are at risk for developing new neurologic deficits. Small degrees of change can be tolerated without resulting in major neurologic deficits” (LaRoche and Haider 2018, 269). This finding has relevance to ischemic changes seen in some ICU patients (i.e., SAH & DCI). The EEG is a valuable monitor in these patients and is very sensitive in identifying and preventing changes prior to development of new neurologic deficits. Lessons from Quantified EEG Analysis In the ICU, automated extraction and trending of quantified EEG (i.e., QEEG) features can assist staff in monitoring EEG. Features most commonly chosen for monitoring are: 1) the percentage of alpha activity (relative alpha), 2) the total power or amplitude, 3) the ratio between alpha and delta activity (i.e., ADR) and 4) the spectral edge or mean frequency (Banoczi and Nuwer 1993). Each of these features can be monitored in channels over several scalp regions. Caution: Such quantified summaries of EEG activity are subject to technical artifacts, including head move ment, muscle activity, swallowing, suctioning, electrocardiogram (ECG) and others. The technologist must document all equipment attached to the patient as well as any clinical activity present. From their earliest work in the ICU, Quinonez and Banoczi (1988), state that a major lesson from QEEG analysis concerns the need to review the actual raw EEG data. Therefore, it is necessary for the raw EEG to be stored during long-term monitoring for later review as needed. Lessons from Routine ICU EEG Testing Analysis of traditional EEG in the ICU has identified several features of these recordings that can be helpful. EEG features such as occasional frontally predominant brief 2/second generalized rhythmic delta activity (if 1–10% of record), pseudo- periodic LPDs, or TWs can help to identify the patient’s physiologic state. Epileptiform activity can also be revealed on the EEG. Patients placed into barbiturate coma benefit from maintenance of a burst-suppression EEG pattern for determining the dose of barbiturates needed for each individual patient. ICU-CEEG MONITORING 251 For patients on long-term EEG monitoring, a common response to adverse EEG changes is to obtain a computerized tomography (CT) or magnetic resonance imaging (MRI) scan. Disc electrodes need to be removed to obtain artifact-free imaging scans or CT/MRI compatible electrodes otherwise should be used. As a compromise, subdermal needle electrodes held in place with collodion-soaked gauze squares are usually not a serious impediment to the reading of the CT scan. If using needle electrodes, they should be single-use and disposable. Their advantage is the speed of application and that they tend to produce stable recordings. Their disadvantages include needle stick risk during application and because they have higher resistance/impedance they have a susceptibility to recording artifacts. (LaRoche and Haider 2018). Lessons from Long-Term Monitoring for Epilepsy Recording experiences in epilepsy monitoring units (EMUs) have shown that EEG electrodes can be left in place continuously for several weeks. Implanted sphenoidal wire electrodes, and intracranial electrodes also yield adequate recordings for weeks at a time. Large volumes of recordings can be obtained when patients are monitored for many days or several weeks. A major problem is sifting through the large volume of data. When all the data is to be reviewed, some labs use fast review techniques to scan the EEG on a video monitor at 10–20 times real speed. Events can be tagged for detailed review of specific sections of EEG data corresponding to the specific events of interest. This requires adequate storage of clock time along with EEG signals. Automated detectors can identify some epileptic seizures but are still quite imperfect. They will also detect many false alarms from muscle artifact, swallowing and other rhythmic artifacts. Alternately, they can be tuned to avoid false identification of non- ictal events but will miss identifying many epileptic seizures. One approach has been to allow the automated seizure detectors to tentatively identify many possible seizures, and then require human visual analysis of the candidate events. This can be accom plished by either reviewing the events on a monitor and/or by quick analysis of automatic software-identified printed candidate events (Banoczi 1995). Lessons from cEEG Monitoring Experience in SAH with Supplemental QEEG Trends In the UCLA neurosurgical ICU, continuous ICU EEG monitoring has been routinely performed using an 8-electrode longitudinal bipolar montage with electrodes placed according to the International 10/20 System (F4–T4, T4–P4, P4–O2, F3–T3, T3–P3, P3–O1). The ACNS guidelines currently recommend all 19 scalp leads be applied and recorded whenever possible. The low-frequency filter was set to 0.3 Hz to better record delta activity. The high-frequency filter was set to 35 Hz to avoid aliasing artifact. 252 ICU-CEEG MONITORING Frequency analysis was performed automatically on each 2 second epoch of EEG. These frequency analysis results were then averaged over 2 minutes. The averaged relative alpha and total power values were printed and displayed as a continuous histogram over each 8–12 hours of EEG monitoring. This color-coded histogram was printed periodically. Frequency band for relative alpha was 6–14 Hz, expressed as a percentage of 1–20 Hz activity. Artifact detection was done by daily review of recorded raw EEG and the corresponding spectral trends. In earlier initial experience with QEEG ICU monitoring in patients with SAH, it was observed that marked reduction in relative alpha variability occurred during vasospasm (ASET – The Neurodiagnostic Society 2013; Vespa et al. 1997) (Figure 11). Technical and Safety Protocols The Neurodiagnostic technologist should select the electrode application method that is most appropriate to the patient’s clinical situation. Different types of electrodes are available for use in the ICU. Critical care monitoring programs should consider FIG. 11. Example of an 8-hour EEG trend recorded from 8 electrodes placed according to the International 10–20 system and arranged in a longitudinal bipolar montage. Frequency filters were set at 0.3 Hz and 35 Hz (a bandpass of 0.3–35 Hz). The LF was set to 0.3 Hz to better record delta activity. The HF was set to 35 Hz to avoid slow frequency activity from aliasing artifacts. (This figure is an example of the technique used in the UCLA neurosurgical ICU). ICU-CEEG MONITORING 253 the electrode cost, ease of use, time for application, imaging compatibility, durability and recording characteristics when selecting the electrodes. Gold or silver disk cup electrodes are typically used for cEEG monitoring and when possible, CT and/or MRI compatible electrodes should be utilized. Neurodiagnostic technologists who perform cEEG in the ICU should be extensively trained and credentialed. Some of their duties include evaluating the quality of the EEG tracing and video (if utilized) and they should be proficient at recognizing artifacts. Once identified, technologists should be able to identify the origin of the artifact and eliminate and/or monitor them as well as properly document them in the recording. Additional artifacts commonly seen in the ICU often come from equipment and patient care maneuvers such as ventilator, suctioning, hemodialysis, infusion pumps, oscillating beds, chest percussion, etc. (Hirsch and Brenner 2010; Tatum 2019). “Technically, ICU monitoring is often obscured by muscle artifact. Unconscious intubated patients can be placed on neuromuscular junction blockade when muscle artifact significantly interferes with the monitoring. Conversely, a patient on neuro muscular blockade agents for respiratory reasons can be followed by EEG long-term monitoring to determine the level of arousal of the patient. Patients are often placed on ventilators, warming beds, infusion pumps and various monitors. These can all create artifacts on the EEG” (LaRoche and Haider 2018, 235–236). “There are several challenges in obtaining access to the patient for EEG. The need for sterility hampers free movement for setting up and obtaining the EEG. There may be a lack of access to the scalp because of open wounds. In some cases, the scalp area is not available because of burns. There might be limited access from bandages. The placement of electrodes may be hindered by open skull and scalp wounds. Timing for patient access may be hindered by competition for EEG recording time from other studies and scans. Quite often, competition for time from nursing care personnel and therapists can delay EEG procedures” (Kaplan 2006, 83). Even when the patient is accessible, the presence of bandages, open wounds, extensive burns, problems of infection, and the need for sterility impose challenges. Minimization of safety concerns related to prolonged monitoring is particularly important. A detailed protocol for the prevention of skin breakdown should be developed (Gaspard 2016). For these types of situations, a technologist must famil iarize him or herself with the protocols and Standard Precautions that must be undertaken (OSHA 2004; CDC 2019). Guidelines are available for proper use of personal protective equipment and infection prevention procedures. Knowledge of current infection prevention practices and recommendations is essential for every Neurodiagnostic technologist, including those working in the ICU (Bonner and Davidson 2020). The Neurodiagnostic technologist should communicate important EEG changes to the EEG/ICU management teams. The technologist should complete a daily EEG impression worksheet/report. Additionally, the technologist is required to perform 254 ICU-CEEG MONITORING daily maintenance of the recording and the cEEG equipment. The quality of the recording should be checked at least twice daily. Clinical and EEG reactivity should be assessed daily (ASET 2011). Suggested Recording Duration Protocols Recordings should begin as soon as feasible according to the condition of the patient and be discontinued after 24–48 hours if no seizures are identified. This period can be extended to 48 hours if sedating medications have been administered and are being weaned. If the patient exhibits suspected NCS, and if no seizures occur, the study can be discontinued after 24–48 hours. In patients with confirmed NCS, the recording can be discontinued 24 hours after the last seizure. When a patient has refractory NCSE, the study can be stopped 24 hours after the administration of IV ASDs. In the patients with NCSE, it is important to monitor them with burst- suppression measures and QEEG seizure identification methods. After traumatic brain injury, the patient must be evaluated for NCS by recording a 24–48-hour period. QEEG seizure identification methods should be utilized including QEEG variability. After cardiopulmonary arrest, the purpose of monitoring is to look for NCS as well as for prognostication. These patients are often placed on a hypothermia protocol. It is acceptable to discontinue the recording 24 hours after the patient becomes normother mic and no seizures have occurred. QEEG seizure identification methods and mea sures of burst suppression can be utilized in these patients. Those patients presenting with a SAH from an aneurysm bursting should be placed on cEEG monitoring for up to 10 days. This covers the peak risk period for DCI after the occurrence of SAH and should be monitored with QEEG techniques to identify ischemia such as occurs with vasospasm and NCS (Herman et al. 2015b; Muniz et al. 2016). Critical Care cEEG Acquisition, Review & Access Critical care cEEG amplifiers, analog-to-digital converters, central processing units, software and monitors should meet ACNS recommended specifications (ACNS - The American Clinical Neurophysiology Society 2016a). Critical care cEEG acquisition computers should have sufficient processing capability to perform simultaneous EEG and video acquisition, QEEG analysis and spike/seizure detection. Ideally, the com puters should have automatic rejection (removal) capability (Herman et al. 2015b). There are several techniques (methods) for artifact reduction. The software can identify most artifacts. Specific channels like ECG, Electromyography (EMG) and Electro-oculogram (EOG), EEG containing the artifact will be rejected. “Artifact regres sion techniques, which are like rejection and subtraction techniques are effective in removing ECG and eye movements” (Tatum 2019, 378). In automated subtraction, segments of EEG recordings are rejected when they exceed a predetermined threshold. ICU-CEEG MONITORING 255 Algorithms such as least mean square use adaptive digital filters and require obtaining a suitable reference signal. The blind source separation (BSS) algorithm is most widely used for artifact reduction. It can use principal component analysis and canonical correlation analysis to identify waveform components. Independent Component Analysis is widely used for artifact reduction and can be combined with other methods for superior results (Tatum 2019). A typical review station should have the following characteristics (Herman et al. 2015b, 100): Monitors should be at least 20 inches with 1200–1600 pixels. Dual monitors can be helpful, especially if QEEG trends are displayed along with the raw EEG. The software should have the ability to display both video and ongoing EEG studies. Nurse and/or technologist monitoring stations should have the capability to simultaneously view video and sometimes EEG and QEEG trends. Automated seizure detection capability. Networking, Remote Access, and Data Storage Equipment In order to quickly identify seizures and ischemia, the EEG data, including trends, should be available for review by designated personnel inside and outside of the hospital. 100 megabits per second networks are necessary for continuous data transfer (Herman et al. 2015b, 101–102). File servers can function as data storage equipment, allowing multiple users to connect to the same data from multiple locations. For the data to be secure, the ICU EEG program should employ virtual private networks (VPNs), which is commonly used. This allows multiple users to access the same data securely from multiple locations (Herman et al. 2015b, 101–102). Advanced Cutting-Edge Techniques and Future Directions for EEG Monitoring in the ICU Some of these advanced techniques involve improved artifact identifications and removal. In discussions with Persyst Development Corporation, I became aware recently that seizure detection algorithms with half the processing time and ten-fold the accuracy have become available. Alarms that indicate the presence of specific EEG/QEEG patterns and multimodal data integration (MMDI) of measures such as EEG, video, ICP, brain oxygenation, cerebral perfusion and CO2 are being utilized. (LaRoche and Haider 2018) (Figures 12–15). 256 ICU-CEEG MONITORING FIG. 12. This 62-year-old male suffered a left frontal-parietal intracerebral hemorrhage (ICH). The patient was obtunded but not showing clinical signs of seizure activity. Using the Moberg CNS ICU monitor, the nurse detected subclinical seizures which allowed prompt treatment of the patient. This case is courtesy of Michelle Hill RN (Ohio Health Riverside Methodist Hospital) and Moberg ICU Solutions (Ambler, PA). Used with permission for educational purposes only. Currently, there are several artifact removal techniques. Some are effective in removing EMG, EOG and ECG. Newer techniques in development will be capable of eliminating challenging artifacts such as patient movements (LaRoche and Haider 2018). BSS, as mentioned above, is a new technique that separates the original signals detected from various sources and subsequently eliminates common types of artifact. Maximum Noise Fraction, a relatively new blind source separation technique, has also demonstrated effectiveness in artifact reduction during EEG recordings (Tatum 2019). Advanced seizure detection software uses neural networks to identify changes in the EEG that represent electrographic seizures. They can also be helpful by identify ing specific artifacts to avoid false positives (Scheuer et al. 2020) (Figures 16–23). The development of more sophisticated alarms is used to identify the presence of certain EEG patterns. They can be set to alert the physicians and staff if the data falls outside a specified range (Hirsch and Brenner 2010). cEEG monitoring is generally performed at large hospitals and academic institu tions with adequate technical resources, although some community hospitals are initiating monitoring. Performing cEEG is resource intensive. Neurodiagnostic ICU-CEEG MONITORING 257 FIG. 13. This patient was a 57-year-old male with multiple contusions, a traumatic subarachnoid hemorrhage, and a subdural hemorrhage after a fall. A trend display was configured with MMDI to show ICP (mean), PbtO2 (Continuously monitors oxygen saturation, noninvasively. Enables decision support for detecting and managing hypoxic events & tissue desaturations) and perfusion (cerebral blood flow). The trended EEG was collected from depth electrodes. Spreading depolarizations appeared in the depth recording. This highlights the importance to concurrently monitor and review brain electrical activity and physiology. This case is courtesy of Brandon Foreman MD (University of Cincinnati) and Moberg ICU Solutions (Ambler, PA). Used with permission for educational purposes only. 258 ICU-CEEG MONITORING FIG. 14. Applications such as the CNS Envision (Moberg ICU Solutions, Ambler, PA) allow the creation of integrated displays that combine raw and processed EEG with video and vital signs, such arterial blood pressure, ICP and brain tissue oxygen (PbtO2). [Demo data used in this example with a volunteer’s permission.]. ICU-CEEG MONITORING FIG. 15. The integrated displays show the recorded physiology alongside the raw and processed EEG to highlight the effects of a dose of Labetalol (green marker). This case is courtesy of Brandon Foreman, MD, University of Cincinnati, and Moberg ICU Solutions, Ambler, PA. Used with permission for educational purposes only. 259 260 ICU-CEEG MONITORING FIG. 16. This is the first seizure recorded in this patient using the Persyst software. This case is courtesy of Persyst Development Corporation (Prescott, Az). Used with permission for educational purposes only. ICU-CEEG MONITORING FIG. 17. This is a split-screen EEG during the previous seizure with artifact reduction “ON” for waveforms and trends. 261 262 ICU-CEEG MONITORING FIG. 18. This is the EEG display during the seizure onset with artifact reduction “OFF.” ICU-CEEG MONITORING FIG. 19. This is a display of the same page with artifact reduction “ON.” 263 264 ICU-CEEG MONITORING FIG. 20. This is an ICU recording showing five seizure detections and the associated trends are clearly visible. This case is also courtesy of Persyst Development Corporation (Prescott, AZ). Used with permission for educational purposes only. ICU-CEEG MONITORING FIG. 21. This is a split-screen recording during the second seizure. 265 266 ICU-CEEG MONITORING FIG. 22. This tracing was obtained during a period of electrode artifact with artifact reduction “OFF” for both EEG waveforms and trends. ICU-CEEG MONITORING FIG. 23. This was recorded with artifact reduction “ON” to reduce muscle, eye blink, lateral eye movement and electrode artifact. This electrode artifact was removed by dynamically disabling the bad channels and indicated by the dashed line. 267 268 ICU-CEEG MONITORING technologists and neurophysiologists are often in short supply. Collaboration with information technology experts is needed for proper networking and remote access and efficient data transmission and storage. Creative solutions to these requirements must be implemented (LaRoche and Haider 2018). CONCLUSION My purpose in writing this paper was to examine the use and benefits of cEEG monitoring of ICU patients, review the indications for the use of cEEG and discuss technical issues and concerns when performing ICU-cEEG monitoring. There are several specific clinical aims in long-term EEG monitoring in the neurologic ICU setting. Monitoring should lead to timely medical or surgical inter vention. This process involves detection of new complications, as well as monitoring treatment of known impairment to see if therapies are being carried out satisfactorily. EEG monitoring also aims to detect subclinical seizures in patients with decreased consciousness, including those NCS associated with stroke, head trauma or other cerebral impairment. EEG monitoring, by measuring the depth of barbiturate- induced burst suppression, allows feedback on the amount of barbiturates needed for an individual patient. EEG monitoring may allow assessment of prognosis for survival or neurologic recovery. Critical care cEEG is used to identify secondary brain injuries such as seizures and ischemia in critically ill patients. There is increasing evidence that these secondary injuries can worsen neurologic outcome, although few prospective studies have yet demonstrated that treatment of EEG-identified changes improves neurologic outcome. The most common indication for cEEG is for identification of NCS and NCSE, with ischemia identification and prognostication as fewer com mon uses. A corresponding decrease in EEG peak frequency from 6.6 to 5.6 Hz that occurs with vasospasm and decreased CBF (cerebral blood flow) has been reported to have a strong correlation with regional CBF measures in chronically brain-injured patients. Trends such as RAV, ADR, total power and spectral edge help to identify and to focus attention on changes in the EEG within large amounts of data. So, what is next for ICU-cEEG monitoring? Continuous digital video-EEG monitoring is the standard of care in neurologic/neurosurgical ICUs and is being utilized in other ICUs as well. Improvements in electrodes, improved artifact rejection techniques, computer networking, QEEG analysis, seizure detection algorithms, alarms and clinical awareness of NCS are well underway. It will soon be practical to offer remote review of urgent EEGs in the ICUs and emergency departments for patients with impaired mental status in most academic centers, and increasingly in more large community hospitals. ICU-CEEG MONITORING 269 RECOMMENDED WEBSITES: www.acns.org www.aset.org www.ilae.org www.ccemrc.org www.moberg.com www.persyst.com NOTES 1. Proposed ACNS 2021 Guideline 14: Standardized Critical Care EEG Terminology: Electrographic Status Epilepticus (ESE) and Electrographic Seizures (ESz). 2. Proposed ACNS 2021 Guideline 14: Standardized Critical Care EEG Terminology: Rhythmic and Periodic Patterns (RPP). 3. Proposed ACNS 2021 Guideline 14: Standardized Critical Care EEG Terminology: Unilateral Independent Discharges (UIPDs). 4. Proposed ACNS 2021 Guideline 14: Standardized Critical Care EEG Terminology: Cyclic alternating pattern of encephalography (CAPE). 5. Proposed ACNS 2021 Guideline 14: Standardized Critical Care EEG Terminology: Highly Epileptiform Bursts, Identical Bursts, Anterior-posterior (AP) gradient and Breach effect. ACKNOWLEDGMENTS I would like to thank my supportive wife, LuAnn Banoczi, for her helpful comments and assistance in the preparation of this manuscript. Without the applica tion of her production skills, this article would not have been satisfactorily completed. I am also very appreciative of the contributions that Moberg ICU Solutions and Persyst Development Corporation provided by allowing me to include their trend displays and case histories. DISCLOSURE STATEMENT No potential conflict of interest was reported by the author. 270 ICU-CEEG MONITORING REFERENCES ACNS - The American Clinical Neurophysiology Society. 2016a. Guideline 4: recording clinical EEG on digital media. [accessed 2020 May 20]. https://journals.lww.com/clinicalneurophys/Fulltext/2016/ 08000/American_Clinical_Neurophysiology_Society.6.aspx. ACNS - The American Clinical Neurophysiology Society. 2016b. Guideline 6: minimum technical stan dards for EEG recording in suspected cerebral Death. [accessed 2020 May 20]. https://journals.lww. com/clinicalneurophys/Fulltext/2016/08000/American_Clinical_Neurophysiology_Society.8.aspx. ASET. 2011. National competency skill standards for performing ICU/cEEG monitoring. [accessed 2020 May 20]. https://www.aset.org/files/public/ICU_National_Competency_Skill_Standards_ Approved_2011.pdf. ASET – The Neurodiagnostic Society. 2013. EEG pattern recognition for the Bedside Caregiver: continuous EEG in pediatric patients. Kansas City (MO): ASET - The Neurodiagnostic Society. Banoczi WR. 1995. Electroneurodiagnostic END intensive care monitoring. Am J EEG Technol. 35 (1):46–57. Banoczi WR, Nuwer MR. 1993. Quantitative trending in the ICU (abstract). Am J EEG Technol. 33(4):307. Bonner AM, Davidson P. 2020. Infection prevention: 2020 review and update for neurodiagnostic technologists. Neurodiagnostic J. 60(1):11–35. Centers for Disease Control (CDC). 2019. Standard precautions. Updated 2018 June 18. [accessed 2019 Dec 24]. https://www.cdc.gov/oralhealth/infectioncontrol/summary-infection-prevention-practices/ standard-precautions.html. Chiappa KH, Hoch D. 1993. Electrophysiologic monitoring. In: Roper A, editor. Neurological and neurosurgical intensive care. New York (NY): Raven Press; p. 129–155. Claassen J, Hirsch LJ, Frontera JA, Fernandez A, Schmidt M, Kapinos G, Wittman J, Connolly ES, Emerson RG, Mayer SA. 2006. Prognostic significance of cEEG monitoring in patients with poor-grade subarachnoid hemorrhage. Neurocrit Care. 4:103–112. Foreman B, Claassen J, Khaled KA, Jirsch J, Alschuler DM, Wittman J, Emerson RG, Hirsch LJ. 2012. Generalized periodic discharges in the critically ill: a case-control study of 200 patients. Neurology. 79 (19):1951–1960. Gaspard N. 2016. Current clinical evidence supporting the use of continuous EEG monitoring for delayed cerebral ischemia detection. J Clin Neurophysiol. 33(3):211–216. Herman ST, Abend NS, Bleck TP, Chapman KE, Drislane FW, Emerson RG, Gerard EE, Hahn CD, Husain AM, Kaplan PW, et al. 2015a. Critical care continuous EEG task force of the American clinical neurophysiology society. Consensus statement on continuous EEG in critically ill adults and children, part 1: indications. J Clin Neurophysiol. 32(2):87–95. Herman ST, Abend NS, Bleck TP, Chapman KE, Drislane FW, Emerson RG, Gerard EE, Hahn CD, Husain AM, Kaplan PW, et al. 2015b. Consensus statement on critically ill adults and children, part II: personnel, technical specifications, and clinical practice. J Clin Neurophysiol. 32(2):96–108. Hill CE, Blank LJ, Thibault D, Davis KA, Dahodwala N, Litt B, Willis AW. 2018. Continuous EEG is associated with favorable hospitalization outcomes for critically ill patients. Neurology. 92(1):e9–e17. Hirsch LJ. 2004. Continuous EEG monitoring in the intensive care unit: an overview. J Clin Neurophysiol. 21:332–340. Hirsch LJ, Brenner RP. 2010. Atlas of EEG in critical care. Hoboken (NJ): Wiley-Blackwell; p. 39, 216; 257; 266–9; 273–4; 276–9. Johnson EL, Kaplan PW, Ritzl EK. 2018. Stimulus-induced rhythmic, periodic, or ictal discharges (SIRPIDs). J Clin Neurophysiol. 35(3):229–233. Juan E, Kaplan PW, Oddo M, Rossetti AO. 2015. EEG as an indicator of cerebral functioning in postanoxic coma. J Clin Neurophysiol. 32(6):465–470. Kalamangalam GP, Phlmann-Eden B. 2018. Ictal-interictal continuum. J Clin Neurophysiol. 35(4):274–278. Kaplan PW. 2006. EEG monitoring in the intensive care unit. Am J END Technol. 46(2):81–97. Kerrigan JF 2009. EEG in the ICU: rhythmical and periodic patterns. ASET 50th Annual Conference, Phoenix, Arizona. August 7, 2009. Kubota Y, Nakamoto H, Egawa S, Kawamata T. 2018. Continuous EEG monitoring in ICU. J Intensive Care. 6:39. ICU-CEEG MONITORING 271 LaRoche SM, Haider HA. 2018. Handbook of ICU EEG monitoring. New York (NY): Springer Publishing (Demos Medical Publishing); p. 13; 32–40; 42–51; 99; 126; 160–9; 180–1; 186–99; 234–8; 256–7; 269; 281–96; 360–1; 381–5. Leppik IE 2012. Nonconvulsive Seizures in the ICU. ASET Webinar Presentation. Minneapolis (MN), August 2, 2012. Muniz CF, Shenoy AV, O’Connor KL, Bechek SC, Boyle EJ, Guanci MM, Tehan TM, Zafar SF, Cole AJ, Patel AB, et al. 2016. Clinical development and implementation of an institutional guideline for prospective EEG monitoring and reporting of delayed cerebral ischemia. J Clin Neurophysiol. 33 (3):217–226. Nuwer MR. 1991. Electrophysiologic monitoring in the neurosurgical-neurological ICU: the UCLA experience and overview of the directions for this field. Los Angeles (CA): American Academy of Neurology (AAN) course handout. Occupational Safety and Health Administration (OSHA). 2004. Personal protective equipment (3151-12R). [accessed 2019 Dec 24]. https://www.osha.gov/Publications/osha3151.pdf. Quinonez D, Banoczi W. 1988. Computerized EEG topographic brain mapping: noninvasive monitoring in the intensive care unit. J West Soc Electrodiag Technol. 39:1–13. Scheuer ML, Wilson SB, Antony A, Ghearing G, Urban A, Bagic AI. 2020. Seizure detection: interreader agreement and detection algorithm assessments using a large dataset. J Clin Neurophysiol. May 27, 2020 - Volume Publish Ahead of Print Issue:1–9. doi:10.1097/WNP.0000000000000709. Schmitt SE, Pargeon K, Frechette ES, Hirsch LJ, Dalmau J, Friedman D. 2012. Extreme delta brushes: a unique EEG pattern in adults with anti-NMDA receptor encephalitis. Neurology. 79(11):1–13. Schutt-Ainé K. 2018. Treatment options for anti-N-methyl-D-aspartate receptor encephalitis. Neurodiagn J. 58(4):226–234. Swisher CB, Sinha SR. 2016. Utilization of quantitative EEG trends for critical care continuous EEG monitoring. J Clin Neurophysiol. 33(6):538–544. Tatum WO. 2019. Atlas of artifacts in clinical neurophysiology. New York (NY): Springer (Demos Medical Publishing); p. 121; 378–85. van Putten MJAM, Jofmeijer J. 2016. EEG monitoring in cerebral ischemia: basic concepts and clinical applications. J Clin Neurophysiol. 33:203–210. Vespa PM, Nuwer MR, Juhász C, Alexander M, Nenov V, Martin N, Becker DP. 1997. Early detection of vasospasm after acute subarachnoid hemorrhage using continuous EEG ICU monitoring. Electroencephalogr Clin Neurophysiol. 103(6):607–615. Yoo JY, Rampal N, Petroff OA, Hirsch LJ, Gaspard N. 2014. Brief potentially ictal rhythmic discharges in critically ill adults. JAMA Neurol. 71(4):454–462.