Heat Transfer (1-67) PDF
Document Details
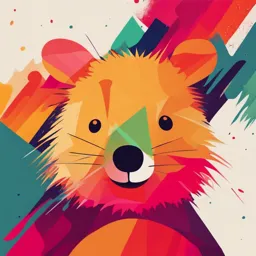
Uploaded by TantalizingWichita1576
King Mongkut's University of Technology Thonburi
Sukanya Phuengjayaem
Tags
Summary
This document is a set of lecture notes on heat transfer, covering principles, applications, and equipment used in bioprocessing. It includes detailed diagrams and examples relevant to the topic.
Full Transcript
Heat transfer BIOLOGY, FA ICRO CU M...
Heat transfer BIOLOGY, FA ICRO CU M LT OF Y T EN OF TM SC Sukanya RI DEPAR IEN CE, KING’S M Phuengjayaem, PhD ONBU O TH NG GY K MIC 321-Basic Bioprocess Engineering UT O UN IVE NOL RSITY OF TECH Objective 1. Know To know the basic principles of heat transfer. 2. Understand To understand the phenomenal of heat transfer in bioprocess. 3. Explain To explain the heat transfer model. 4. Apply To operate and control heat transfer in fermentation process. 2 AGENDA Principal of Heat Heat transfer Heat transfer in Calculate heat transfer in bioreactor sterilization transfer coefficience Conduction Heat transfer equipment Batch Heat Model of heat transfer Convection sterilization of Heat transfer between liquids Radiation fluids Continuous heat Overall Heat Transfer sterilization of Coefficient liquids 3 Principal of Heat transfer Conduction: Conduction: Convection: Radiation: https://jackwestin.com/resources/mcat-content/energy-changes-in-chemical-reactions/heat-transfer-conduction-convection-radiation 4 Cengel, Y. A. et al. (1998) HEAT TRANSFER MECHANISMS CONDUCTION Conduction is the transfer of energy from the more energetic particles of a substance to the adjacent less energetic ones as a result of interactions between the particles. Conduction can take place in solids, liquids, or gases. Heat conduction through a large plane wall of thickness x and area A. 5 Cengel, Y. A. et al. (1998) Direction of heat transfer Heat transfer in the positive direction of a coordinate axis is positive and in the opposite direction it is negative. Therefore, a positive quantity indicates heat transfer in the positive direction and a negative quantity indicates heat transfer in the negative direction. 6 HEAT TRANSFER MECHANISMS Cengel, Y. A. et al. (1998) A system of fixed mass is called a closed system that Under steady conditions and in the absence of any work interactions, involves heat transfer only and no work interactions the conservation of energy relation for a control volume with one across its boundary, the energy balance relation inlet and one exit with negligible changes in kinetic and potential reduces to energies can be expressed as Where Q = the amount of net heat transfer to or from Where the system. 𝑚ሶ = 𝜌𝜗As is the mass flow rate m = Mass 𝑄ሶ = the rate of net heat transfer into or out of the control volume. Cv = specific heat at constant volume Cp = specific heat ∆= Temperature difference As = surface area 𝜌 = density 𝜗 = volume When heat is transferred at a constant rate of 𝑄,ሶ the ∆T= Temperature difference amount of heat transfer during a time interval t can be ሶ determined from Q = 𝑄·t. 7 HEAT TRANSFER MECHANISMS Cengel, Y. A. et al. (1998) The rate of heat conduction through a plane layer which is called Fourier’s law of heat conduction is proportional to the temperature difference after J. Fourier. across the layer and the heat transfer area but is inversely proportional to the thickness of the layer. Here ∆T/ ∆x is the temperature gradient, which is the slope of the temperature curve on a T-x diagram (the rate of change of T with x), at location x. Where the constant of proportionality k is the thermal conductivity of the material, which is a measure of the ability of a material to conduct heat. 8 HEAT TRANSFER MECHANISMS Example 1: Heat conduction The roof of an air heater home is 6 m long, 8 m wide, and 0.25 m thick, and is made of a flat layer of concrete whose thermal conductivity is k 0.8 W/m ·°C. The temperatures of the outer and the inner surfaces of the roof one day are measured to be 10°C and 20°C, respectively, for a period of 10 hours. Determine (a) the rate of heat transfer through the roof that day and (b) the cost of that heat transfer to the homeowner if the cost of electricity is THB 5/kWh. SOLUTION The inner and outer surfaces of the flat concrete roof of home are maintained at specified temperatures during a day. The heat transfer through the roof and its cost that day are to be determined. Assumptions 1 Steady operating conditions exist during the entire day since the surface temperatures of the roof remain constant at the specified values. 2 Constant properties can be used for the roof. Properties The thermal conductivity of the roof is given to be k 0.8 W/m · °C. 9 HEAT TRANSFER MECHANISMS Example 1: Heat conduction Calculation (a) Noting that heat transfer through the roof is by conduction and the area of the roof is A 6 m x 8 m = 48 m2, the steady rate of heat transfer through the roof is determined to be (20−10)℃ = (0.8 W/m·°C)(48 m2) = 1536 w = 1.536 kw 0.25 𝑚 (b) The amount of heat lost through the roof during a 10-hour period and its cost are determined from Q = 𝑄ሶ · t = (1.536 kW)(10 h) = 15.36 kWh Cost (Amount of energy)(Unit cost of energy) = (15.36 kWh)(THB 5/kWh) = 76.8 THB 10 PHYSICAL MECHANISM OF CONDUCTION Cengel, Y. A. et al. (1998) The location of a point is specified as (x, y, z) in rectangular coordinates, as (r, ø, z) in cylindrical coordinates, and as (r,ø,𝜃) in spherical coordinates The various distances and angles involved when describing the location of a point in different coordinate systems. 11 HEAT TRANSFER MECHANISMS Example 2: Heat conduction Dimensions and thermal conductivity of food/beverage container. Inner and outer surface temperatures. FIND: Heat flux through container wall and total heat load. 12 HEAT TRANSFER MECHANISMS Example 2: Heat conduction Assumptions: 1. Steady-state conditions 2. Negligible heat transfer through bottom Wall 3. Uniform surface temperatures and one-dimensional conduction through remaining walls. Given: H = 0.6 m W1 = 0.8 m W2 = 0.6 m K = 0.023 W/m-K L = 0.025 m T1 = 2 °C T2 = 20 °C 13 HEAT TRANSFER MECHANISMS Example 2: Heat conduction Calculation: q = q”Atotal = q” [H(2W1 + 2W2 )+W1 W2] q =16.6 W/m2 [0.6m(1.6m+1.2m)+ (0.8m 0.6m)] = 35.9 W 14 HEAT TRANSFER MECHANISMS Cengel, Y. A. et al. (1998) Convection Convection is the mode of heat transfer between a solid surface and the adjacent liquid or gas that is in motion, and it involves the combined effects of conduction and fluid motion. Heat transfer from a hot The cooling of a boiled egg surface to air by convection. by forced and natural convection. 15 PHYSICAL MECHANISM OF CONVECTION Cengel, Y. A. et al. (1998) Convection Heat transfer through a solid is always by conduction, since the molecules of a solid remain at relatively fixed positions. Heat transfer through a liquid or gas, however, can be by conduction or convection, depending on the presence of any bulk fluid motion. Heat transfer through a fluid is by convection in the presence of bulk fluid motion and by conduction in the absence of it. Therefore, conduction in a fluid can be viewed as the Heat transfer from a hot surface to the limiting case of convection, corresponding to the case of surrounding fluid by convection and conduction. quiescent fluid. 16 PHYSICAL MECHANISM OF CONVECTION Cengel, Y. A. et al. (1998) Convection Convection heat transfer strongly depends on - the fluid properties dynamic viscosity , - thermal conductivity k, - density, 𝜌 - and specific heat Cp, - fluid velocity, 𝜗 It also depends on the geometry and the roughness of the solid surface, in addition to the type of fluid Heat transfer through a fluid sandwiched flow (such as being streamlined or turbulent). between two parallel plates. 17 PHYSICAL MECHANISM OF CONVECTION Cengel, Y. A. et al. (1998) 𝑄ሶ conv= hAs(Ts -T) (W) where h = convection heat transfer coefficient, (W/m2.°C) As = heat transfer surface area, (m2) Ts = temperature of the surface, (°C) T = temperature of the fluid sufficiently far from the surface, (°C) The cooling of a hot block by forced convection. 18 PHYSICAL MECHANISM OF CONVECTION Cengel, Y. A. et al. (1998) We resort to forced convection We turn on the fan on hot We stir our soup and blow on a hot slice whenever we need to increase the summer days to help our body of pizza to make them cool faster. rate of heat transfer. cool more effectively. 19 PHYSICAL MECHANISM OF CONVECTION Cengel, Y. A. et al. (1998) VELOCITY BOUNDARY LAYER The development of the boundary layer for flow over a flat plate, and the different flow regimes. 20 PHYSICAL MECHANISM OF CONVECTION Cengel, Y. A. et al. (1998) The viscosities of liquids and gases The viscosities of liquids decrease with temperature, whereas the viscosities of gases increase with temperature Where 𝜏s = the surface shear stress Cf = the dimensionless friction coefficient ρ = density ϑ = velocity The viscosity of liquids decreases, and the viscosity of gases increases with temperature. 21 PHYSICAL MECHANISM OF CONVECTION Example 3: Convection Width, input power and efficiency of a transmission. Temperature and convection coefficient associated with air flow over the casing. FIND: Surface temperature of casing. 22 PHYSICAL MECHANISM OF CONVECTION Example 3: Convection ASSUMPTIONS: Given: 1. Steady state Pi = 250 hp 2. Uniform convection coefficient Po = ⴄPi and surface temperature hi = 200 W/m2-K 3. Negligible radiation w = 0.3 m Tꚙ = 30 °C 23 PHYSICAL MECHANISM OF CONVECTION Example 3: Convection Calculation: From Newton’s law of cooling where the output power is h Pi and the heat rate is q = Pi - Po = Pi (1-h ) =150 hp746 W/hp 0.07 = 7833W 24 HEAT TRANSFER MECHANISMS Cengel, Y. A. et al. (1998) RADIATION Radiation is the energy emitted by matter in the form of electromagnetic waves (or photons) as a result of the changes in the electronic configurations of the atoms or molecules. Unlike conduction and convection, the transfer of energy by radiation does not require the presence The energy of the sun reaches the earth by radiation. of an intervening medium. 25 HEAT TRANSFER MECHANISMS RADIATION Radiation differs from the other two heat transfer mechanisms in that it does not require the presence of a material medium to take place. In fact, energy transfer by radiation is fastest (at the speed of light) and it suffers no attenuation in a vacuum. Also, radiation transfer occurs in solids as well as liquids and gases. In most practical applications, all three modes of heat transfer occur concurrently at varying degrees. But heat transfer through an evacuated space can occur only by radiation. A hot object in a vacuum chamber loses heat by radiation only. 26 HEAT TRANSFER MECHANISMS Cengel, Y. A. et al. (1998) RADIATION The conduction or convection takes place in the direction of decreasing temperature; that is, from a high-temperature medium to a lower-temperature one. It is interesting that radiation heat transfer can occur between two bodies separated by a medium colder than both bodies. For example, solar radiation reaches the surface of the earth after passing through cold air layers at high altitudes. Also, the radiation absorbing surfaces inside a greenhouse reach high temperatures even when its plastic or glass cover Unlike conduction and convection, heat transfer by remains relatively cool. radiation can occur between two bodies, even when they are separated by a medium colder than both of them. 27 HEAT TRANSFER MECHANISMS THERMAL RADIATION Cengel, Y. A. et al. (1998) Eb(T ) = 𝜎 T 4 (W/m2) Where Eb = blackbody emissive power. 𝜎 = the Stefan–Boltzmann constant (w/m2K4) T = the absolute temperature of the surface in K. The electromagnetic wave spectrum. 28 PHYSICAL MECHANISM OF RADIATION Cengel, Y. A. et al. (1998) Application of Radiation Food is heated or cooked in a microwave oven by Solar radiation is incident on the absorber absorbing the electromagnetic radiation energy surface which is made of aluminum coated with generated by the magnetron of the oven. black chrome. 29 PHYSICAL MECHANISM OF RADIATION Cengel, Y. A. et al. (1998) THERMAL RADIATION Thermal radiation is continuously emitted by all matter whose temperature is above absolute zero. That is, everything around us such as walls, furniture, and our friends constantly emits (and absorbs) radiation. Thermal radiation is also defined as the portion of the electromagnetic spectrum that extends from about 0.1 to 100 m, since the radiation emitted by bodies due to their temperature falls almost entirely into this wavelength range. Thus, thermal radiation includes the entire visible Everything around us constantly and infrared (IR) radiation as well as a portion of emits thermal radiation. the ultraviolet (UV) radiation. 30 Heat transfer in bioreactor https://en.wikipedia.org/wiki/Baffle_(heat_transfer) Applications of heat transfer in bioreactor operation Sterilization Temperature control The first is in situ batch sterilization of liquid The second is cooling water. This is used to bring the medium. In this process, the fermenter vessel temperature back to normal operating conditions. containing medium is heated using steam and Metabolic activity of cells generates a substantial held at the sterilization temperature for a amount of heat in fermenters; this heat must be period of time. removed to avoid temperature increases. Most fermentations take place in the range 30-37 °C; in large-scale operations, cooling water is used to maintain the temperature usually to within 1 ° C. 32 HEAT TRANSFER EQUIPMENT Heat transfer configurations for bioreactors: Doran, P. M. (1995). 1. jacketed vessel; 2. external coil; 3. internal helical coil The fermenter may Coil through which The coil can be operated with high have an external steam or cooling cooling water velocities and the jacket. water is circulated. entire tube surface is exposed to the reactor contents providing a relatively large heat transfer area. 33 HEAT TRANSFER EQUIPMENT Heat transfer configurations for bioreactors: Doran, P. M. (1995). 4. internal baffle-type coil 5. external heat exchanger The coil can be operated with high The external heat exchange unit cooling water velocities and the shown in is independent of the entire tube surface is exposed to reactor, easy to scale up, and can the reactor contents providing a provide greater heat transfer capacity relatively large heat transfer area. than any of the other configurations. 34 HEAT TRANSFER EQUIPMENT Problem of internal structures Doran, P. M. (1995). The coil must be able to withstand the thermal and mechanical stresses generated Internal structures interfere with Film growth of cells on the inside the fermenter during sterilisation and mixing in the vessel and make > internal heat transfer > agitation; the possibility of nonsterile cleaning of the reactor difficult. surfaces. coolant leaking from fractured metal joints in the cooling coil significantly increases the risk of culture contamination. > Because of these problems with internal coils, use of an external jacket is preferable for cell cultures with relatively low cooling requirements. Fermentations with high heat loads may require an internal cooling coil together with an external jacket to achieve the necessary rate of cooling. 35 HEAT TRANSFER EQUIPMENT Doran, P. M. (1995). The temperature of the cooling water rises as it flows through the tube and takes up heat from the fermentation broth. The water temperature increases steadily from the inlet temperature Tci to the outlet temperature Tco. On the other hand, if the contents of the fermenter are well mixed, temperature gradients in the bulk fluid are negligible and the fermenter temperature is uniform at TF. Temperature change in internal coil 36 General Equipment for Heat Transfer Doran, P. M. (1995). Double-Pipe Heat Exchanger - A double-pipe heat exchanger consists of two metal pipes, one inside the other. - One fluid flows through the inner tube while the other fluid flows in the annular space between the pipe walls. When one of the fluids is hotter than the other, heat flows from it through the wall of the inner tube into the other fluid. As a result, the hot fluid becomes cooler and the cold fluid becomes warmer. Double-pipe heat exchanger 37 General Equipment for Heat Transfer Doran, P. M. (1995). Double-Pipe Heat Exchanger The changes in temperature of the two fluids as they flow countercurrently through the pipes. The four terminal temperatures are as follows: Thi is the inlet temperature of the hot fluid, Tho is the outlet temperature of the hot fluid, Tci is the inlet temperature of the cold fluid, and Tco is the outlet temperature of the cold fluid leaving the system. A sign of efficient operation is Tco close to Thi, or Tho close to Tci. Temperature changes for countercurrent flow in a double-pipe heat exchanger. 38 General Equipment for Heat Transfer Doran, P. M. (1995). Double-Pipe Heat Exchanger The temperature curves for cocurrent flow. It is not possible using cocurrent flow to bring the exit temperature of one fluid close to the entrance temperature of the other; instead, the exit temperatures of both streams lie between the two entrance temperatures. Cocurrent flow is not as effective as countercurrent operation because less heat can be transferred; consequently, cocurrent flow is Temperature changes for cocurrent flow in a double-pipe applied less frequently. heat exchanger. 39 Shell-and-Tube Heat Exchangers Single-pass shell-and-tube heat exchanger. Cengel, Y. A. et al. (1998) > The simplest form, called a single- pass shell-and-tube heat exchanger. > Shell-and-tube heat exchangers are used for heating and cooling of all types of fluid. > They have the advantage of containing very large surface areas in a relatively small volume. Single-pass shell-and-tube heat exchanger. 40 Shell-and-Tube Heat Exchangers Doran, P. M. (1995) This Figure represents an end-view of the heat exchanger from inside the header. Open tubes are fitted into the tube sheet so that fluid in the header, which is prevented from entering the main cavity of the exchanger by the tube sheet, must pass into the tubes. The tube-side fluid leaves the exchanger through another header at the outlet. Shell-side fluid enters the internal cavity of the exchanger and flows around the outside of the tubes in a direction that is largely countercurrent to the tube fluid. Heat is exchanged across the tube walls from the hot fluid to Tube sheet for a shell-and-tube heat exchanger. the cold fluid. 41 Shell-and-Tube Heat Exchangers Doran, P. M. (1995) This situation should be avoided because, after the cross, the cold fluid is cooled rather than heated. The solution is an increased number of shell passes or, more practically, provision of another heat exchanger in series with the first. Temperature changes for a double tube-pass heat exchanger. 42 MECHANISMS OF HEAT TRANSFER Doran, P. M. (1995) In most heat transfer equipment, heat is exchanged between fluids separated by a solid wall. Heat transfer through the wall occurs by conduction. In this section we consider equations describing the rate of conduction as a function of operating variables. The rate of heat conduction through the wall is given by Fourier’s law: Where 𝑄 ̇ is the rate of heat transfer, k is the thermal conductivity of the wall, A is the surface area perpendicular to the direction of heat flow, T is temperature, Heat conduction through a flat wall. y is distance measured normal to A. 43 Thermal Conductivities 44 COMBINING THERMAL RESISTANCES IN SERIES Doran, P. M. (1995) Consider the three-layer system with surface area A, layer thicknesses B1, B2, and B3, thermal conductivities k1, k2, and k3, and temperature drops across the layers of ΔT1, ΔT2, and ΔT3. If the layers are in perfect thermal contact so there is no temperature drop across the interfaces, the temperature change across the entire structure is: ΔT = ΔT1 + ΔT2 + ΔT3 where R1, R2, and R3 are the thermal resistances of the individual layers: Heat conduction through three resistances in series. 45 HEAT TRANSFER BETWEEN FLUIDS Doran, P. M. (1995) Thermal Boundary Layers Heat transfer between fluids that are separated by a pipe wall: (a) the longitudinal pipe cross-section identifying a segment of the pipe wall; (b) the magnified detail of the pipe wall segment showing boundary layers and temperature gradients at the wall. The bulk temperature of the hot fluid away from the wall is Th; Tc is the bulk temperature of the cold fluid. Thw and Tcw are the respective temperatures of the hot and cold fluids at the wall. 46 HEAT TRANSFER BETWEEN FLUIDS Doran, P. M. (1995) Individual Heat Transfer Coefficients The rate of heat transfer through each thermal boundary layer in the fluid is given for steady-state conduction: Where h is the individual heat transfer coefficient, A is the area for heat transfer normal to the direction of heat flow, and ΔT is the temperature difference between the wall and the bulk stream. ΔT = Th-Thw for the hot-fluid film; ΔT = Tcw = Tc for the cold-fluid film. 47 OVERALL HEAT TRANSFER COEFFICIENT Doran, P. M. (1995) To calculate the rate of heat transfer in each boundary layer requires knowledge of ΔT for each fluid. This is usually difficult because we do not know Thw and Tcw; it is much easier and more accurate to measure the bulk temperatures of fluids rather than wall Where temperatures. ΔT is the overall temperature difference This problem is overcome by introducing the overall heat between the bulk hot and cold fluids. transfer coefficient, U, for the total heat transfer process The units of U are the same as for h (e.g., through both fluid boundary layers and the wall. U is W m-1 K-1 or Btu h-1 ft-1 F-1). defined by the equation: 48 HEAT TRANSFER BETWEEN FLUIDS Doran, P. M. (1995) Overall Heat Transfer Coefficient The resistance yields an expression for the total resistance to heat transfer, RT The surface area of the wall of a cylinder is equal to the circumference multiplied by the length: A = 2πRL Effect of pipe wall thickness on the surface area for heat transfer. 49 Calculation of Heat Transfer Coefficients Doran, P. M. (1995) Flow in Tubes without Phase Change Individual heat transfer coefficients for flow in pipes and stirred vessels can be evaluated using empirical correlations expressed in terms of dimensionless numbers. The general form of correlations for heat transfer coefficients is: This equation applies for Re > 2100 (turbulent flow) and for fluids with viscosity no greater than 2 mPa s. 50 CALCULATION OF HEAT TRANSFER COEFFICIENTS Flow in Tubes without Phase Change Doran, P. M. (1995) The heat transfer coefficient in stirred vessels When heat is transferred to or from a jacket rather than a coil, the correlation is slightly modified: depends on the degree of agitation and the properties of the fluid. When heat is transferred to or from a helical coil in the vessel, h for the tank side of the coil can be determined using the following equation Where µb = the bulk viscosity µw = the viscosity at the wall For low-viscosity fluids such as water, the viscosity at the wall μw can be assumed equal to the bulk viscosity μb. 51 CALCULATION OF HEAT TRANSFER COEFFICIENTS Example 4 Heat transfer coefficient for a stirred vessel Doran, P. M. (1995) 52 CALCULATION OF HEAT TRANSFER COEFFICIENTS Example 4 Heat transfer coefficient for a stirred vessel Doran, P. M. (1995) To calculate Nu, with μb = μw: Calculating h for this value of Nu The heat transfer coefficient is 1.55 kW/m2 ·°C. 53 LOGARITHMIC-MEAN TEMPERATURE DIFFERENCE Doran, P. M. (1995) When one fluid in the heat exchange system remains at a constant temperature. For the case of a fermenter at constant temperature TF cooled by water in a cooling coil can be simplified to: Flow configuration for a heat exchanger. Where Tci is the inlet temperature of the cooling water. Tco is the outlet temperature of the cooling water. 54 LOGARITHMIC-MEAN TEMPERATURE DIFFERENCE Example 5: Log-mean temperature difference Doran, P. M. (1995) A liquid stream is cooled from 70 °C to 32 °C in a double-pipe heat exchanger. Fluid flowing countercurrently with this stream is heated from 20 °C to 44 °C. Calculate the log-mean temperature difference. At one end of the equipment The log-mean temperature difference is 18 °C. 55 Heat transfer in sterilization Stirred tank bioreactor system Doran, P. M. (1995) 56 HEAT TRANSFER IN STERILIZATION PROCESS Batch Heat Sterilisation of Liquids Doran, P. M. (1995) Depending on the rate of heat transfer from the steam or electrical element, raising the temperature of the medium in large fermenters can take a considerable period of time. Once the holding or sterilisation temperature is reached, the temperature is held constant for time thd. Cooling water in the coils or jacket of the fermenter is then used to reduce the medium temperature to the required level. Variation of temperature with time for batch sterilisation of liquid medium. 57 HEAT TRANSFER IN STERILIZATION PROCESS Doran, P. M. (1995) Let us denote the number of contaminants present in the raw medium N0. During the heating period this number is reduced to N1. At the end of the holding period, the cell number is N2. The final cell number after cooling is Nf. Ideally, Nf is zero: at the end of the sterilization cycle we want no contaminants to be present. However, because absolute sterility would require an infinitely long sterilization time, it is theoretically impossible to achieve. Normally, the target level of contamination is expressed as a fraction of a cell, which is related to the probability of contamination. Reduction in the number of viable cells with time during batch sterilization. 58 HEAT TRANSFER IN STERILIZATION PROCESS Graph of generalized temperature time profiles for the heating and cooling stages of a batch sterilization cycle. 59 HEAT TRANSFER IN STERILIZATION PROCESS Doran, P. M. (1995) Continuous Heat Sterilization of Liquids Raw medium entering the system is first preheated by hot, sterile medium in a heat exchanger > Steam is then injected directly into the medium as it flows through a pipe; as a result, the temperature of the medium rises almost instantaneously to the desired sterilization temperature. > The medium is cooled instantly by flash cooling > Further cooling takes place in the heat exchanger where residual heat is used to preheat incoming medium. > The sterile medium is then ready for use in the fermenter. Continuous sterilising equipment: continuous steam injection with flash cooling 60 HEAT TRANSFER IN STERILIZATION PROCESS Doran, P. M. (1995) Continuous Heat Sterilization of Liquids Advantage > Cost saving Usually use in medium suspension Easy to clean and maintain. Rapid to heat and cool. Disadvantage > Steam injection is dilution of the medium by condensate. Foaming from direct steam injection can also cause problems with operation of the flash cooler. Continuous sterilising equipment: heat transfer using heat exchangers. 61 HEAT TRANSFER IN STERILIZATION PROCESS Doran, P. M. (1995) The rates of heating and cooling in continuous sterilizers are much more rapid than in batch systems Variation of temperature with time in the continuous sterilisers: (a) continuous steam injection with flash cooling; (b) heat transfer using heat exchangers. 62 HEAT TRANSFER IN STERILIZATION PROCESS Berenjian, A. (2019) Where N0 is the number of viable organisms present at the start of the sterilization treatment, Nt is the number of viable organisms present after a treatment period, t minutes. The slope of which equals-k. This kinetic description makes two predictions Plots of the proportion of survivors and the natural logarithm of that appear anomalous: the proportion of survivors in a population of microorganisms 1. An infinite time is required to achieve sterile subjected to a lethal temperature over a time period conditions (ie, Nt = 0). 2. After a certain time, there will be less than one viable cell present. 63 HEAT TRANSFER IN STERILIZATION PROCESS Berenjian, A. (2019) Plots of the Behavior of Mixed Microbial Cultures Subjected to a Lethal Temperature Over a Time Period (a) The effect of a sterilization treatment on a mixed culture consisting of a high proportion of a very sensitive organism (b) The effect of a sterilization treatment on a mixed culture consisting of a high proportion of a relatively resistant organism. 64 Comparison batch and continuous sterilization Advantage of batch sterilization VS Advantage of continuous sterilization 1. Easy to operate and inexpensive 1. Maintaining superiority of medium equipment quality 2. There is a lower risk of contamination, 2. Easy to scale up production as heat transfer occurs in the tank 3. Easy automatic control system during sterilization. 4. Reduce the efficiency of increasing and 3. Simplify manual control decreasing of steam. 4. Easy to have a high solid proportion. 5. Reduced cycle time for sterilization 6. Reduce fermentation tank corrosion 65 References ▪ Berenjian, A. (Ed.). (2019). Essentials in fermentation technology. Springer. ▪ Cengel, Y. A., Klein, S., & Beckman, W. (1998). Heat transfer: a practical approach (Vol. 141). Boston: WBC McGraw-Hill. ▪ Doran, P. M. (1995). Bioprocess engineering principles. Elsevier. ▪ Incropera, F. P., DeWitt, D. P., Bergman, T. L., & Lavine, A. S. (1996). Fundamentals of heat and mass transfer (Vol. 6, p. 116). New York: Wiley. ▪ Stanbury, P. F., Whitaker, A., & Hall, S. J. (2013). Principles of fermentation technology. Elsevier 66 Thank you If you have any question, please feel free to ask me via: Sukanya Phuengjayaem, PhD E mail: [email protected] Sci 5th floor, room SCL513, Department of Microbiology, KMUTT Tel: 02-4708897 MIC 321-Basic Bioprocess Engineering 67