Goals and Objectives Exam 1 PDF
Document Details
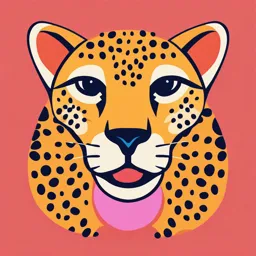
Uploaded by SmartFable2525
Tags
Summary
This document covers various topics related to the foundations of biology, including the origins of the universe and Earth, timescales of life, biomolecules, biochemistry, and cellular structures. It emphasizes the common features of living organisms and explores the cell as the universal building block of life, including different types of cells and their organelles.
Full Transcript
Unit 1 Foundations § Origins of the Universe and Earth: o The Universe was created around 14 billion years ago, basically the big bang theory. From the Big Bang theory, heat and energy, life, and most chemical elements started to appear. The elements st...
Unit 1 Foundations § Origins of the Universe and Earth: o The Universe was created around 14 billion years ago, basically the big bang theory. From the Big Bang theory, heat and energy, life, and most chemical elements started to appear. The elements started to diversify and form in the inorganic (rocks are cooled down inorganic material) and the organic substances (carbon, nitrogen, and other elements). o As the universe started to cool down after the Big Bang theory, it started to form into different areas in the solar system, into stars and planetary systems. § Timescale of life on Earth: o The earth was placed in an optimal place in the universe, with not being so close to the sun and not being too far away, having certain features such as the atmosphere and the ozone layer to protect us humans and other organisms. Having unique and optimal conditions for the development of life, from thermal energy and radiation to revolutions around the sun and self, and the water on Earth is from solid to vapor. § Water has always been on earth since the formation of the land. § The temperature on earth self reduces and fluctuates and shields against the strong exposure to ionizing radiation. § Biomolecules and Biochemistry: o Around 4 billion years ago life arose from simple microorganisms coming together and being able to extract from the environmental chemical compounds and then developing and mutating into getting energy from sunlight, to synthesize more complex biomolecules. o Biochemistry is the study of matter in living organisms, or other words the chemistry of living matter. o Biochemistry = Chemistry of Biology = Physiological Chemistry = Biological Chemistry o Biomolecules and Biochemical Reactions = all chemical substances and processes occurring in living organisms. § Objective of Biochemistry: o To explain and to describe the molecular logic of life all of the molecular level structures, mechanisms, and chemical processes shared by all organisms. § Common Features of Living Organisms: o There is a high degree of chemical complexity and microscopic organization, or in other words a systemically balanced system in using energy to maintain the intrinsic structures/systems in the organism. § From the very long polymers, each having certain characteristics sequences of subunits, unique 3D structure, and highly specific binding partners in the cell. o Ability to extract and produce, transform, and systematically use energy to create and maintain intricate structures and to do mechanical, chemical, osmotic, and electric work. § The idea of plant cells being able to capture sunlight and carbon dioxide and turn them into nutrients and energy for them to grow and produce mammals’ oxygen daily. § In other words, helps the organism to stay alive and counteract the tendency of all matter to decay toward a more disordered state, coming to equilibrium with its surroundings. o The organism’s components have a defined function which is also dynamic and coordinated to work together and regulate interactions without hurting the organism. § The collection of molecules carries out a program, the result of which is to reproduce the program and self-perpetuation of the collection of the molecules. § All of this means LIFE. o The organism has a good sense to detect alterations in its surroundings and to respond to them for the betterment of the organism. § This occurs in the simplest cells to the most complex organisms and structures. § They can adapt to changes in the environment by changing their internal chemistry or their location in the current environment. o Capacity for precise self-replication and self-assembly. This all occurs from the DNA since the whole cell's life and purpose are fully encoded in the DNA. § Many cells in the human body can replicate and create identical daughter cells to adapt to the new environment (survival of the fittest), hence even a lot of animals and mammals give off certain traits to the progeny that comes from the mother/father or both. o Also, cells can change over time by gradual evolution to adapt to the new environmental standards that they are surrounded by. § The Cell as Universal Building Blocks of Life: o All living organisms are made of cells. o Unicellular – single-celled, are the simplest living organisms. o Multicellular – many-celled, are larger organisms, with each different cell, having different functions. § These different cells adopt all of their components for their functions. An all-or-nothing kind of approach. o Cells have some common features but can contain unique components for different organisms which can be seen at the biochemical level. § Cellular Foundations of Life and Biochemistry: o Types of cells / Common and Particular Organelles – § Water is the main component of all of the cells. § They are made from the same major components/ building blocks of life. § Animals Cells – · Unique Structures: o Lysosome – degrades intracellular debris, filled with digestive enzymes that degrade unneeded cellular debris. § Plant Cells – · Unique Structures: o Chloroplast – harvests sunlight and produces ATP and carbohydrates. o Starch Granule – temporarily stores carbohydrate products of photosynthesis. o Thylakoids – sites of light-driven ATP synthesis. o Cell Wall – provides shape and rigidity; protects the cell from osmotic swelling. o Vacuole – degrades and recycles macromolecules and stores metabolites and large quantities of organic acids. o Plasmodesma – provides a path between two plant cells. o Glyoxysome – contains enzymes of the glyoxylate cycle. o Cell Wall – of the adjacent cell. § Common Structures Between Both Cells – · Ribosomes – protein-synthesizing machines, and degradation. · Peroxisome – oxidizes fatty acids. · Cytoskeleton – supports cells, and aids in movement of organelles. · Transport vesicle shuttles lipids and proteins between ER, Golgi, and plasma membrane. · Golgi Complex – processes, packages, and targets proteins to other organelles or for export. Plays a central role in the synthesis and processing of lipids and membrane proteins. · Smooth Endoplasmic reticulum (SER) – site of lipid synthesis and drug metabolism · Nucleus – contains the genes/chromatin, enclosed by a double membrane, called nuclear envelope, making up the eukarya. · Nucleolus – site of ribosomal RNA synthesis. · Rough Endoplasmic Reticulum (RER) – site of much protein synthesis. · Mitochondrion – oxidizes fuels to produce ATP, the site of most energy-extracting reactions of the cell. · Plasma Membrane – separates the cell from the environment and regulates the movement of materials into and out of the cell. Composed of lipid and protein molecules that form a thin, tough, pliable, hydrophobic barrier around the cell, forming a barrier against the inorganic ions in the surrounding area. · Nuclear envelope – segregates chromatin (DNA + Protein) from cytoplasm. · Cytoplasm – composed of an aqueous solution, cytosol, and a variety of suspended particles with specific functions. · Nucleoid – not separated from the cytoplasm in the middle of the cell which is in the bacterial and archaea cells à prokaryotes. o Structural Features – § All cells share a certain structural feature. § Size of Cells – · The smallest cells are defined by the minimum number of biomolecules required to achieve all life features. o Bacterial Mycoplasma – 300 nm in diameter, so a filter of 0.22 is the perfect size to make sure the product of this reaction is sterile. · The upper limit of cell size is probably set by the diffusion rate of solute molecules in an aqueous system. o Oxygen, nutrients, metabolites, and many more. o Around 60 microns. § Shape of Cells – · Determined by various factors: type of cell, the environment in which they live, their function, and role in more complex systems of cells (tissues, organs). § The differences in structural and functional features between different types of cells can be exploited to control their growth/selective killing through the use of various chemical, biochemical, and biological agents (some of them use drugs). o Main Source of Energy – § Organisms differ widely in their source of energy (chemical or photosynthetic) and biosynthetic precursors. § Chemical – · Chemotrophs – energy derived from oxidation of a chemical fuel (food). · Carbon Source o CO2 o Chemoautotrophs – synthesize all of their biomolecules directly. o Hydrogen-, sulfur-, iron-, nitrogen-, and carbon monoxide-oxidizing bacteria. · Organic Compounds o Chemoheterotrophs – require some preformed organic nutrients made by other organisms. o Final electron acceptor § O2 § All animals; most fungi, protists, bacteria, humans. o Final electron acceptor § Not O2 · Organic compounds · Fermentative bacteria such as Lactococcus lactis. § Not O2 · Inorganic compounds and Pseudomonas denitrificans. § Light – · Phototrophs – trap and use sunlight for food and energy. · Carbon source o CO2 § Photoautotrophs § Use H2O to reduce CO2? · Yes à Oxygenic photosynthesis (plants, algae, cyanobacteria) · No à Anoxygenic photosynthesis bacteria (green and purple bacteria) – do not need oxygen · Carbon source o Organic compounds o Photoheterotrophs o Green non-sulfur bacteria, purple non-sulfur bacteria (specialized bacteria) § Chemical Foundations of Life and Biochemistry: o Bulk elements – § Carbon, hydrogen, oxygen, nitrogen, phosphorus, sodium, chloride, potassium, calcium, and sulfur § They are common elements for biological systems, and they are needed every day in large amounts (g/days). Make close to 99% of the mass of cells, and since they are the lightest elements, they make 1, 2, 3, and 4 bonds, and they have the strongest bonds. o Trace elements – § Magnesium, vanadium, chromium, manganese, iron, cobalt, nickel, copper, zinc, selenium, molybdenum, and iodine. § They are present in less than 1% of the mass of the human body and cells, but they have an essential role in human life, due to them being essential to the function of specific proteins, including many enzymes. o Central Role of Carbon – § It is important due to its small size, low electronegativity, and propensity to form covalent bonds with itself or with other elements. § Single bond hybridization is sp3, the double bond is sp2, and the triple bond is sp, which dictates geometry and the molecule's shape, same for other elements also. o Functional Groups – § § Each of these functional groups can be found in all of the chemical compounds, organic and inorganic compounds, they can be used to induce chemical interactions, chemical recognition, and biochemical function. § Depending on the functional groups, the function of biological molecules strongly depends on the 3D structures of the compound. § Due to their complex structures and their folds into their 3D structures, these molecules only fit certain molecules and they can bind. The binding of chiral biomolecules is stereospecific, meaning that only one stereoisomer will be accommodated. o Structure of Biomolecules – § Stereoisomers: are molecules with the same molecular formula and sequence of atoms in the molecule, but with different 2D orientations of their atoms in space. · Geometric Isomers o Cis – same side o Trans – opposite sides § They cannot be interconverted by breaking the bonds holding them in those positions. § They can have different physical and chemical properties. · There could be two almost identical compounds, but since one is cis and the other is trans, one could have a different function, or one could taste sweet and the other bitter. · In other words they can elicit different biological effects. o Maleic Acid (cis) vs. Fumaric acid (trans) o There could be chemicals that can switch structures when they get isomerized by light shining onto the chemical. o Enantiomers – chiral compounds that are mirror images of each other but are not superposable. § They have identical physical properties (except concerning polarized light) and react identically with achiral reagents. o Diastereomers – chiral objects, that are not mirror images of each other and they are not superposable images of each other, usually having more than one chiral center. § They have different physical and chemical properties. · A chiral center is a carbon that has 4 different elements attached to it. · Achiral center is when two or more of the attached elements on the carbon atoms are the same. § Number of stereoisomers: 2n = 4 (n = number of asymmetric carbons) § Physical Foundations in Living Organisms: o Living organisms have to perform energy transduction to accomplish work, to stay alive, and to maintain their structures and dynamic composition, which is different from the surrounding environment. o Molecules are permanently synthesized and broken down; living organisms are in permanent exchange of energy and matter with their surroundings, which means they are open systems. o First Law of Thermodynamics: “total amount of energy in the Universe remains constant, although the form of energy may change, between each organism.” (mechanical, works, heat, chemical, osmotic) o Creating and maintaining order in the living system requires work and energy, therefore living systems perform both catabolic processes, harvesting energy from nutrients, food, and sunlight, and storing it into chemical energy, and anabolic processes, using stored chemical energy to synthesize building blocks and macromolecules. o Living systems exist in a dynamic steady state, maintaining concentrations of nutrients, ions, building blocks, and macromolecules relatively constant and very different from their concentrations in the environment. o In nature systems tend to evolve to complete randomness (infinite entropy); fighting entropy to maintain the dynamic steady state requires work and energy. o Chemical Energy – § It is stored in ATP (chemical currency of energy) and the reduced form of electron carries NADH, FADH2. · Dynamic transformations are used to generate and consume chemical energy to maintain the dynamic steady state, which can be analyzed from the angle of thermodynamics and kinetics. o Thermodynamics – § Analyze how stable one state is vs another, analyzing the relationships between all forms of energy (heat and chemical energy). § Gibbs developed the theory of energy changes during chemical reactions: · ΔG = ΔH – TΔS o ΔG = variation of the free energy content. o ΔH = variation of enthalpy, reflecting the change in the number and type of chemical bonds broken and formed during a chemical reaction. o ΔS = variation of entropy – the change in the system’s randomness. o T = absolute temperature (K). § Thermodynamics analyzes the difference between two states (initial and final) and does not care about the intermediate states, or dynamics of the system: · ΔG < 0 – the reactions occur spontaneously (no need for catalysis, free energy is released (so the energy of the products is lower than the reactants), exergonic process (free energy released)). · ΔG = 0 – the reaction is at equilibrium, with no moving of the reactants to the products. · ΔG > 0 – the reaction requires free energy, meaning it is not spontaneous, it can proceed only in the opposite directions (endergonic process – free energy needed). § The energy coupling link reactions in biology (bioenergetics): chemical coupling of exergonic and endergonic reactions allowing otherwise unfavorable reactions if the overall free energy is < 0. · The high-energy molecule, ATP, reacts directly with the metabolite that needs “activation”. · We can force a reaction to occur by coupling it to a stronger unfavorable reaction to make it occur. § Endergonic cellular reactions are driven by coupling them to exergonic chemical processes through shared chemical intermediates. § Consecutive reactions – the sum of the consecutive ΔG biochemical pathways. They are additive, so if the sum of all of the steps is negative then it is favorable so it occurs spontaneously, meaning it can occur and move in the forward direction or it goes from reactants to products. · The free energy change for ATP hydrolysis is large and negative; phosphorylation is done with ATP instead of phosphate, § Kinetics – analyzing how quickly or slowly species react in living systems. (LOOK AT SLIDES) · A Keq > 0 means the reaction tends to proceed till reactants are almost completely converted into products. § Kinetics and thermodynamics are interconnected through the relationship between equilibrium constant Keq, ΔGo (standard free-energy change), and temperature. · ΔGo = -RTlnKeq · Big and a negative number in the ΔG means it is an exergonic reaction that proceeds forward. o The force that is driving the system towards equilibrium is when nature wants a reaction to occur, it will have to exert an aggressive exergonic reaction until all of the reactants are used up to produce products. § In certain situations when the ΔG < 0, the reaction occurs too slowly for the needs of the biochemical environment, so nature has certain methods to speed up the process. · Higher temperatures – stability of macromolecules is limiting, or in other words, the elements and the macromolecules can easily be denatured. · Higher concentration of reactants – costly as more valuable starting material is needed, in other words, the reactants need to be a lot for this reaction to occur and go to completion. · Change the reaction by coupling to a fast one – universally used by living organisms. · Lower activation barrier by catalysis – compounds that lower the activation energy for that reaction, but not altering the overall ΔG. It is universally used by living organisms. Also, catalysis increases the rate of a chemical reaction. o Enzymatic catalysis offers – acceleration under mild conditions, high specificity, and possibility for regulation. § Nature has a lot of developed bio-catalysis/bio-enzymes, to speed up reactions daily. · Biochemical pathways – are a series of related/consecutive enzymatically catalyzed reactions (each separate reaction is catalyzed by an enzyme). o Either the whole pathway or center parts of the pathway are controlled to regulate levels of metabolites, to start slowing down the whole pathway. · Metabolic pathway – produces energy or valuable materials. · Signal transduction pathway – transmits information. § Genetic and Evolutionary Foundations: o Life arose around 3.5-3.8 billion years ago. o The most remarkable property of living cells and organisms is their ability to reproduce for countless generations with near-perfect fidelity. § A key step towards this ability was the formation of self-replicating molecules. o Biomolecules first arose through chemical evolution à The abiotic (non-biological) origin of organic biomolecules was proved in 1953 by Stanley Miller/Harold Urey: § It proved the formation of 23 amino acids and 7 organosulfur, plus a large number of simple organic compounds – building blocks for more complex ones. · They proved that amino acids/organic compounds came from inorganic compounds. § Hydrothermal vents on the bottom of the oceans may have been the sites of early biogenesis. · Due to the core of the earth heating the water, at least 100o C constantly and also it has high pressure, so life is forming in this area and floating up to the top. o RNA is believed to be the initial macromolecule used for information storage. § Nucleotides are associated with nucleic acids. § It has also appeared before DNA. § RNA can act both as the information carrier and biocatalyst that is located in ribosomes. § Some viruses use RNA as a primary means of genetic information. § A possible RNA scenario of genetic evolution. § DNA, more stable chemically, has replaced RNA as the main storage of genetic information. o The complementary bases in DNA allow for the replication with near-perfect fidelity: § RNA still occupies a central role in the information flow from DNA to proteins encoded, transcription, and translation. · DNA à RNA à Protein o The mRNA into the cytoplasm of the DNA is translated into the proteins over multiple steps in the middle of the above flow. o Natural selection favors some mutations – changes in hereditary instructions allow evolution. § Mutations occur more or less randomly in DNA and RNA. § Mutated polynucleotides may be transcribed and translated into molecular machinery like proteins. § Mutations that give organisms an advantage in a given environment are more likely to be propagated into becoming the best gene for the survival of the fittest. · When two genes share readily detectable sequence similarities à Homologous (proteins encoded: homologs) · Two homologous genes appear in the same organism à paralogous (protein products: paralogs) · Paralogous genes believed to have been derived by gene duplication, followed by gradual changes in the sequence through mutations · Paralogous proteins are similar in sequence, and 3D structure, although may have acquired different functions. o These mutations in center occasions can make the organism survive on two different forms of nutrients and live a long life, due to the environment or the stress factors that it faced throughout the growing stages of the organism. § Two homologous genes found in different species are said to be orthologous and their proteins are orthologs – they usually have the same functions in both organisms. · Molecular anatomy of the genome reveals evolutionary relationships – molecular phylogeny derived from gene sequences is consistent and, in some cases, even more precise than the classical phylogeny based on macroscopic structures. § The evolution of eukaryotes can also be mediated through endosymbiosis. Unit 2 Water § Structure of water: Octet rule: four electron pairs around an oxygen atom in water ○ These electrons are in four sp3 orbitals ○ Two of these pairs covalently link two hydrogen atoms to a central oxygen atom ○ two remaining pairs remain nonbonding (lone pairs) Water geometry is a distorted tetrahedron The electronegativity of the oxygen atom induces a net dipole moment ○ Dipole moment: measure of the separation of positive and negative charges in a molecule, indicating its polarity. ○ Because of the dipole moment, water can serve as both a hydrogen bond donor and acceptor § Influences of life on earth: The solvent of life on Earth ○ water is the most abundant substance in living systems ~ 70% weight in most organisms ○ shaped evolution of life on Earth first living organisms on Earth doubtless arose in an aqueous environment Water continues to play central role Water molecules exhibit attractive forces through hydrogen bonding and dipole-dipole interactions. ○ Water can dissolve and associate ions via ion-dipole interactions. ○ Water has a slight tendency to ionize, which is crucial for the structure and function of biomacromolecules. Water and its ionizable products, H⁺ (H₃O⁺) and OH⁻ ions, influence the properties of all cellular components. ○ These components include proteins, sugars, nucleic acids, and lipids. ○ Water impacts processes such as ionization and self-assembly of biomolecules. § Hydrogen bonding in solid and liquid water: Water is associated through H bonds in solid (ice) and liquid state Water has many different crystal forms; the hexagonal ice is the most common ○ Hexagonal ice forms a regular lattice, and thus has a low entropy ○ Hexagonal ice contains more hydrogen bonds/water molecule Thus, ice has lower density than water Ice floats on top of water When ice melts, some of the hydrogen bonds are broken which increases density Max water density at 4C In liquid state, there is a dynamic structure in which H bonds are made and destroyed simultaneously ○ Flickering clusters of water ○ 1 water molecule associated with ~3.4 other waters at 25C § H Bonding in water: consequences High boiling and melting point ○ 100C boiling ○ 0C melting ○ Due to hydrogen bonding, water requires more energy to change states. High heat of evaporation ○ relatively high due to hydrogen bonding, as additional energy is needed to break these bonds before water molecules can escape into the vapor phase. ○ This property plays a crucial role in temperature regulation in living organisms through processes like sweating and transpiration. § Properties of water High Polarity ○ Hydrogen bonding, dissolution of ions, polar molecules High Specific Heat Capacity ○ Thermal insulation High Heat of Vaporization ○ Sweating cools the body High Dielectric Constant ○ Electrical insulation (charge separation) Maximum Density ○ at 4o C ○ Ice floats Hydrophobic Effects: ○ Micelles, bilayers & amphipathic compounds assemblies, protein folding, enzyme substrate interactions Colligative Properties ○ Depend on the number of solute particles in a solution rather than type of particles Boiling point elevation Adding a solute (like salt) raises boiling point Freezing point depression Dissolving solutes in water lowers it’s freezing point Vapor pressure lowering Adding a solute reduces tendency of water to evaporate Osmotic pressure When a solute is dissolved in water, it creates pressure that drives water to move through a membrane, balancing concentrations in both sides § H-bond formation with polar solutes Hydrogen bonds are formed by many compounds containing highly electronegative elements F, O, N ○ Dissolve many polar compounds Strength ○ typically 4–6 kJ/mol for bonds with neutral atoms ○ 6–10 kJ/mol for bonds with one charged atom Hydrogen bonds are strongest when the bonded molecules are oriented to maximize electrostatic interaction ○ Maximum strength when aligned ○ Ideally the three atoms involved are in a line § Polarity of biomacromolecules Water and biomolecules with functional groups containing O and N are polar and associate through hydrogen bonding and dipole-dipole interactions. ○ Polar biomolecules are soluble in water (hydrophilic, water-compatible). ○ Non-polar molecules, like hydrocarbons, are not very soluble in water (hydrophobic, water-incompatible). Examples of polar biomolecules ○ Glucose ○ Glycine ○ Aspartate ○ Lactate ○ Glycerol Examples of nonpolar biomolecules ○ Typical wax Ampipathic ○ Contains both polar and nonpolar groups ○ Examples Phenylalinine Phosphatidylcholine § Dissolution of inorganic (ionic) compounds in water High dielectric constant of water ○ Water has a high dielectric constant, which means it can reduce the attraction between oppositely charged ions (e.g., Na⁺ and Cl⁻ in salt). ○ The stronger this property, the weaker the attraction between these ions, especially at larger distances (greater than 40 nm). ○ This helps break the ionic bonds in the salt crystal. Ion-dipole interactions ○ When ions (Na⁺ and Cl⁻) are surrounded by water molecules, they experience strong electrostatic interactions with the water. ○ Water molecules are polar, with a partial positive charge near the hydrogen atoms and a partial negative charge near the oxygen atom. ○ These water molecules form ion-dipole interactions with the charged ions, stabilizing them and lowering the energy of the system. Increase in entropy ○ When the salt dissolves, the highly ordered crystal lattice structure of the solid breaks apart, and the ions become dispersed in solution. ○ This process increases the entropy (disorder) of the system, as the dissolved ions are more randomly distributed in water compared to their ordered arrangement in the crystal. § Solubility of gases in water Polar gases (e.g., ammonia, carbon dioxide) dissolve well in water due to favorable interactions like hydrogen bonding or dipole-dipole interactions with water molecules. Nonpolar gases(e.g., oxygen, nitrogen) have low solubility in water because they cannot form strong interactions with water molecules. ○ Some nonpolar gases can still dissolve in water in small amounts due to induced dipole interactions (weak, temporary attractions between gas molecules and water). ○ Maximum amount of oxygen in water is near poles due to low temperature ○ solubility of non-polar gasses in H2O increases with the decrease of temperature Water molecules prefer to interact with each other through hydrogen bonds, making it energetically unfavorable for nonpolar gases to dissolve. Temperature effects ○ As temperature increases, the solubility of gases in water generally decreases, as more thermal energy allows gas molecules to escape into the air. § Hydrophobic effect and Hydrophobic solutes in water Hydrophobic effect ○ the tendency of non-polar compounds to aggregate in aqueous solution and exclude the water molecules ○ The system presents the smallest hydrophobic area to water/aqueous solvent ○ One of main factors behind: protein folding Non-polar side chains aggregate to minimize exposure to water. protein-protein association Hydrophobic regions of proteins promote aggregation. formation of surfactant micelles and lipid membranes Micelle: Nonpolar tails aggregate while polar heads remain in contact with water. Lipids:Amphipathic molecules arrange to shield hydrophobic regions from water. binding of steroid hormones to their receptors Hydrophobic ligands bind to hydrophobic sites in proteins. Low solubility of hydrophobic solutes can be explained by entropy ○ Bulk water has little order, thus high entropy High entropy means liberty to move ○ Water near a hydrophobic solute is forced to adopt a highly ordered state – low entropy ○ Low entropy is not compensated by attractive forces between the non-polar molecules and water ones (of enthalpic nature), therefore the whole process is thermodynamically unfavorable (ΔG > 0), thus hydrophobic solutes have low solubility. Delta S will be negative Delta G will be positive ○ Hydrophobic tail causes lower entropy as water has to slow around the tail ○ Liquid water has an average of 3 water molecules by bonds If all four hydrogen bonds form, ice forms § Origins of hydrophobic effect Amphipathic fatty acids ○ When dispersed in water, the nonpolar tails of the fatty acids are surrounded by ordered water molecules. Decrease in entropy ○ This ordering of water around the nonpolar tails lowers the system’s entropy, creating an unfavorable state. Self-assembly of nonpolar portions ○ Nonpolar parts of the fatty acids aggregate, reducing the number of ordered water molecules. Entropy increases ○ As water molecules are released from the ordered state, they become more random, increasing the system’s entropy. Sequestration of nonpolar groups ○ All nonpolar portions are hidden from water, further increasing entropy. Polar head groups ○ Only the polar head groups remain exposed to water, allowing for energetically favorable hydrogen bonding with water molecules. Formation of micelles ○ This process leads to the formation of structures like micelles, where nonpolar regions are shielded, and polar regions interact with water. § Consequences of hydrophobic effect the molecular shape of amphiphilic molecules influences the self-assemblies formed ○ Micelles Individual units are wedged shaped (cross section of head greater than that of side chain) Fatty acids ○ Phospholipids Individual units are cylindrical (cross section of head equals that of side chain) Bilayer ○ Vesicles Spherical structure made of lipid bilayers Aqueous cavity ○ Maintains structure and prevents collapsing Liposome ”artificial vesicles” (used for drug delivery) e.g. liposomal doxorubicin Myocet® Vesicles have lower energy, which drives process Hydrophobic effect favors amphipathic ligand binding ○ Binding sites in enzymes and receptors are often hydrophobic, containing ordered water molecules of low entropy Such sites can bind hydrophobic substrates and ligands such as steroid hormones ○ Many drugs are designed to take advantage of the hydrophobic effect ○ entropic gain: when hydrophobic substrates bind to hydrophobic sites in enzymes or receptors. Initially, the water molecules around the hydrophobic regions are highly ordered, leading to low entropy. When the hydrophobic substrate binds to the hydrophobic site, these ordered water molecules are released, increasing their randomness and thus the entropy of the system. This entropic gain contributes to the thermodynamic favorability of the binding process. § Non covalent interactions in biomolecules Non covalent interactions ○ Do not involve the sharing of electrons ○ Important in folding protein All reactions occur at once to fold proteins Types of non covalent interactions ○ H-bonds and Ionic (Coulombic) Interactions Electrostatic interactions between permanently charged species, or between the ion and a permanent dipole ○ Dipole Interactions Electrostatic interactions between uncharged, but polar molecules Hydroxy group to hydroxy group ○ van der Waals Interactions Weak interactions between all atoms, regardless of polarity Attractive (dispersion) and repulsive (steric) component ○ Hydrophobic Effect Complex phenomenon associated with the ordering of water molecules around nonpolar substances and entropy decrease in the system § Collagalitive and Non collagative properties of water Colligative properties of water ○ “Tied together” ○ Concentration dependent The effect of solutes on colligative properties of water does not depend on the (chemical) nature of the solute, just on the concentration (number of molecules of solute added to a given amount of water, because concentration of water is lower in solutions than in pure water Example The effect of adding 1 mol of glucose to 5 mols water in terms of CPW is identical to the addition of 1 mol of ethanol to 5 mols water, as we are adding the same amount of foreign molecules and water will have the same molar concentration in the final solution ○ Altered by solutes of all kind ○ Colligative Properties Depend on the number of solute particles in a solution rather than type of particles Boiling point elevation ○ Adding a solute (like salt) raises boiling point Freezing point depression ○ Dissolving solutes in water lowers it’s freezing point Vapor pressure lowering ○ Adding a solute reduces tendency of water to evaporate Osmotic pressure ○ When a solute is dissolved in water, it creates pressure that drives water to move through a membrane, balancing concentrations in both sides ○ Osmotic pressure is force needed to bring it back Osmotic pressure depends only on the number (concentration) of solutes, not on their MW one large biopolymer elicits the same osmotic pressure as a small molecule, therefore large biopolymers (high MW proteins, polysaccharides, nucleic acids) can be stored in large amounts in cells with negligible contribution to total osmotic pressure of the cell; fuel is stored as polysaccharides, not as simple sugars! Stored as polymer to prevent higher osmotic pressure ○ consequence Cytoplasm of cells is a highly concentrated solution of different biomolecules, ions and has a high osmotic pressure (the more biomolecules we add the higher the osmotic pressure) Approxiamately 30% (the rest is water) Non Colligative properties of water ○ Depend on the nature of the solute ○ Non colligative properties Viscosity The resistance of water to flow. Surface tension Water’s ability to resist external forces due to cohesive forces between molecules. Taste Water’s sensory characteristic, though pure water is generally tasteless. Color Water appears colorless in small quantities but can have a slight blue tint in larger volumes due to the absorption of light. § osmotic pressure impact on cells, isotonic, hypertonic, hypotonic Osmotic pressure ○ Osmosis: water movement through a semipermeable membrane driven by differences in osmotic pressure (conc of water in two solutions separated by the membrane) Differences in concentration of solutes inside/outside of cell triggers water movement through membrane via osmosis ○ Osmotic burst constitutes a big issue plasma contains proteins, ions to be isotonic with the cells hydration of a patient is done with isotonic saline solution, not with pure water! ○ Osmotic pressure depends only on the number (concentration) of solutes, not on their MW All MW contribute the same ○ one large biopolymer elicits the same osmotic pressure as a small molecule, therefore large biopolymers (high MW proteins, polysaccharides, nucleic acids) can be stored in large amounts in cells with negligible contribution to total osmotic pressure of the cell; fuel is stored as polysaccharides, not as simple sugars! Stored as polymer to prevent higher osmotic pressure Isotonic ○ No net water movement Hypertonic ○ Water moves out and cell shrinks Hypotonic ○ Water moves in and cell swells § Ionization of water Formula ○ Polar O-H bonds ○ Water molecules have polar O-H bonds that can dissociate heterolytically. Dissociation products ○ The dissociation of water produces a proton (H⁺) and a hydroxide ion (OH⁻). Rapid and reversible ○ The dissociation of water is a quick and reversible process. Low ionization ○ Most water molecules remain un-ionized, resulting in very low electrical conductivity in pure water (resistance: 18 MΩ cm). Equilibrium ○ The dissociation equilibrium is strongly shifted to the left, favoring un-ionized water molecules. Temperature-dependent ○ The extent of water’s dissociation varies with temperature. § Proton hydration and transport Proton hydration ○ Protons do not exist free in solution. ○ They are immediately hydrated to form hydronium (oxonium) ions. A hydronium ion is a water molecule with a proton associated with one of the non-bonding electron pairs. Hydronium ions are solvated by nearby water molecules. ○ The covalent and hydrogen bonds are interchangeable. This allows for an extremely fast mobility of protons in water via “proton hopping.” ○ Protons move through water faster than other ions. This high ionic mobility helps to explain why acid- base reactions are so fast. § Ionic product of water Concentrations of participating species in an equilibrium constant are not independent but are related via the equilibrium constant equilibrium constant ○ ○ Keq can be determined experimentally, it is 1.8 10–16 M at 25C ○ H2O can be determined from water density, it is 55.5 M Ionic product of water ○ ○ In pure water ○ § pH What is pH ○ Definition: Negative logarithm of the hydrogen ion concentration ○ Simplifies equations ○ The pH and pOH must always add to 14 ○ In neutral solution, [H+]=[OH-] and the pH is 7 Equation ○ Insert § Henderson–Hasselbalch Equation Definition ○ The Henderson–Hasselbalch equation relates the pH of a solution to the pKa (acid dissociation constant) and the ratio of the concentrations of the conjugate base and acid. Use ○ This equation is used to calculate the pH of a buffer solution based on the concentrations of an acid and its conjugate base. Order of equations ○ Start with disassociation equation ○ ○ equilibrium expression for the acid disassociation constant (Ka) ○ ○ Rearrange to solve for [H+]: ○ ○ Take the negative log of both sides ○ ○ Recognize that -log[H+] is the pH and -log Ka is the pKa ○ ○ Final equation ○ pH=pKa + log (conjugate base/acid) § pH Scale Definition ○ The pH scale measures the acidity or basicity of a solution, based on the concentration of hydrogen ions (H⁺). Range ○ The pH scale typically ranges from 0 to 14. ○ Acidic solutions pH < 7 (high concentration of H⁺ ions). ○ Neutral solution pH = 7 (pure water or neutral substances). ○ Basic (alkaline) solutions pH > 7 (low concentration of H⁺ ions). Logarithmic scale ○ Each unit change in pH represents a tenfold change in H⁺ ion concentration. Example: A solution with pH 3 is ten times more acidic than a solution with pH 4. Formula ○ [pH] = -log[H+] ○ [H+] is the molar concentration of hydrogen ions. pOH ○ Measures the concentration of hydroxide ions (OH⁻). ○ pH+pOH= 14 Biological relevance: Maintaining proper pH is crucial for biological processes (e.g., enzyme activity, cellular function). Most biological systems operate around neutral pH (7.2–7.4). § Dissociation of weak electrolytes in water Principle ○ Weak electrolytes dissociate only partially in water ○ Extent of disassociation is determined by the acid disassociation constant Ka ○ We can calculate the pH if the Ka is known but some algebra is needed Example ○ Simplification ○ § Acidity § Weak acids § titration curves Titration is used to determine the amount of acid present in solution, using a strong base (NaOH) of known concentration ○ pH is measured in parallel (via pH electrode, etc) ○ pKa is determined Buffering regions ○ 1 unit up and down Conjugate based Formula ○ § buffers and their impact in cells and organisms Buffers are mixtures of weak acids and their anions (conjugate base) Buffers resist change in pH ○ At pH = pKa, there is a 50:50 mixture of acid and anion forms of the compound ○ Buffering capacity of acid/anion system is the greatest at pH = pKa ○ Buffering capacity is lost when the pH differs from pKa by more than 1 pH unit Acetic Acid-Acetate as a Buffer System Cells and organisms maintain a specific and constant cytosolic pH, usually near 7 ○ phosphate-based buffers are the main ones used intracellularly, doubled by protein buffer The particular R groups of important amino acids influence protein activity. They also contribute to the ability of proteins to act as buffers. § acidosis/alkalosis Normal blood pH is maintained between 7.35 and 7.45 through the bicarbonate buffer system ○ Small change (by even.03) can send someone into a coma ○ The lungs regulate the amount of CO2 in the blood ○ the kidneys regulate the bicarbonate. Acidosis ○ the hydrogen ions are increased while pH and bicarbonate ions are decreased. A greater number of hydrogen ions are present in the blood than can be absorbed by the buffer systems ○ When the pH decreases to below 7.35 an acidotic condition is present ○ Two types of acidosis Respiratory acidosis: Lungs can’t excrete CO2 (hypoventilation) Examples ○ emphysema, chronic bronchitis, asthma, severe pneumonia, cardiac arrest Metabolic acidosis can result in stimulation of chemoreceptors and so increase alveolar ventilation, leading to respiratory compensation, otherwise known as Kussmaul breathing (deep and labored breathing) a specific type of hyperventilation. This is common with diabetic Alkalosis ○ results when the pH is above 7.45 (e.g. in hyperventilation due to stress, anxiety). ○ This condition results when the buffer base (bicarbonate ions) is greater than normal and the concentration of hydrogen ions are decreased ○ Two types of alkalosis Respiratory alkalosis Alveolar hyperventilation ○ Can be caused by anxiety, hysteria and stress, fever and large doses of aspirin, stimulates the respiratory center in the brainstem, meningitis and pregnancy ○ Respiratory alkalosis is often produced iatrogenically during mechanical ventilation of patients ○ Lungs excrete too much CO2 Metabolic alkalosis most commonly occurs with profuse vomiting which depletes H+ ions leading to an increase of bicarbonate in the body. § mechanisms of compensation Respiratory acidosis is compensated by the kidney, in effect causing a metabolic also through an increase in breathing frequency, depth Metabolic alkalosis is compensated by decrease breathing rate, in effect causing acidosis. Renal compensation cause an increase in bicarbonate excretion. Respiratory alkalosis can be compensated by temporary breathing in a paper bag alkalosis and respiratory § Drug ionization and pH dependence of drug absorption The pH in the GI tract ranges from 1.5 in the stomach to 7.6 in the small intestine. Ionizable drugs are absorbed at different locations along the GI tract, depending on their pKa and the local pH. ○ Ionized drugs cannot pass through the lipid bilayer and, therefore, are less likely to be absorbed. ○ § optimum pH for enzyme activity Different enzymes have specific optimum pH levels for maximum activity. Enzymes in the GI tract operate at different locations where the pH varies, helping to process food efficiently at each stage. ○ Unit 3 amino acids, peptides, and proteins § structure of amino acids Building Blocks of Protein ○ Proteins are linear heteropolymers of alpha-amino acids ○ Proteins mediate virtually every process that takes place in a cell ○ Amino acids have properties that are well-suited to carry out a variety of biological functions Capacity to polymerize Amino acids have the ability to link together via peptide bonds, forming long chains called polypeptides. This polymerization is the foundation for building proteins, which are essential for nearly every biological process in the body. Useful acid-base properties Each amino acid contains both an amino group (basic) and a carboxyl group (acidic), giving it unique acid-base properties. ○ These properties allow amino acids to buffer changes in pH, which is vital for maintaining the stability of proteins and enzymes in different environments. Diverse physical properties Amino acids vary in size, polarity, and hydrophobicity, contributing to their diverse physical properties. ○ These differences influence the 3D structure and function of proteins, determining whether they are water-soluble or part of membrane structures. Diverse chemical functionality Amino acids contain different side chains (R-groups) that provide a range of chemical functionalities. ○ These side chains can participate in various biochemical reactions, such as enzyme catalysis, molecular recognition, and signaling pathways. Most Alpha-amino acids share common structural features and are chiral ○ The alpha-carbon always has four substituents and is tetrahedral ○ All amino acids (except proline) have: An acidic carbonyl group (COO-) A basic amino group (H3N+) an alpha-hydrogen connected to the alpha-carbon a fourth substituent (R), unique In glycine, the fourth substituent is also hydrogen R group can be quite complex; nomenclature: Proteins only contain L amino acids ○ the two stereoisomers (enantiomers) of alanine ○ ○ L/D represent the relative configuration ○ of amino acids to L- and D-glyceraldehyde: If relationship object is mirror image, it is enatimoers ○ L/D represent the configuration of the four substituents around the chiral carbon, and are not related with the sense of rotation of polarized light! § properties of amino acids Properties of amino acids ○ Acid-base properties: Amino acids have both an amino group (-NH2) and a carboxyl group (-COOH), which can accept or donate protons, respectively. This dual nature gives them amphoteric properties, allowing them to act as buffers and maintain pH balance in biological systems. ○ Chirality: Most amino acids (except glycine) have a chiral carbon atom, meaning they exist in two mirror-image forms (L- and D-isomers). In biological systems, L-amino acids are predominantly used to build proteins. ○ Zwitterionic form: At physiological pH (~7.4), amino acids exist in a zwitterionic form, where the amino group is protonated (-NH3+) and the carboxyl group is deprotonated (-COO−), making them electrically neutral but still carrying both positive and negative charges. ○ Hydrophobic and hydrophilic properties: The side chains (R-groups) of amino acids can be hydrophobic (nonpolar) or hydrophilic (polar), which influences how they interact with water and other molecules. Hydrophobic amino acids tend to be found in the interior of proteins, while hydrophilic ones are often on the exterior. ○ Ability to form peptide bonds: Amino acids can link together through peptide bonds, forming polypeptides or proteins. This bond forms between the carboxyl group of one amino acid and the amino group of another, releasing a molecule of water. ○ Chemical reactivity: The side chains of amino acids can participate in various chemical reactions. For example, cysteine contains a thiol group (-SH) that can form disulfide bonds, while serine and threonine have hydroxyl groups that are important in phosphorylation reactions. ○ Optical activity: Due to their chiral nature, amino acids can rotate plane-polarized light, a property known as optical activity. The direction and degree of rotation depend on the specific structure of the amino acid. Amino acid NH3+ more basic than their counterpart Amino acid COOH more acidic than their counterpart Average Molecular weight of all 20 amino acids is about 128 ○ BUT there is a greater prevalence of low MW AAs in most proteins so the average MW of protein AAs is closer to 128 If we subtract 18 for water released upon formation of peptide bond, the average MW an amino acid residue = 110. Thus if we know the MW of a protein we can divide by 110 to get the approximate number of amino acids in that particular case § Names of Amino Acids Non polar amino acids, name, and symbols ○ Nonpolar Aliphatic R groups Glycine Gly G Image Alanine Ala A Image Proline Pro P Image Valine Val V Image Leucine Leu L Image Isoleucine Ile I Image Methionine Met M Image ○ Aromatic R groups Phenylalanine (non polar) Phe F Image Tyrosine Tyr Y Image Tryptophan Trip W Image Polar amino acids, name, and symbols ○ Uncharged R groups Serine Ser S Image Threonine Thr T Image Cysteine Cys C Cysteine can form disulfide bonds. Image Asparagine Asn N Image Glutamine Gln Q Image ○ Positively charged R groups (polar, basic side chains, usually protonated at physiologic pH = 7.4) Lysine Lys K Image Histidine His H Image Arginine Arg R Image ○ Negatively charged R groups (polar, acidic side chains, usually fully ionized and negatively charged at physiologic pH = 7.4) Aspartate Asp D Image Glutamate Glu E Image Uncommon amino acids found in proteins ○ In the structure of collagen (fibrous protein in connective tissue) 4-Hydroxyproline Formula 5-hydroxylysin Formula ○ In the structure of myosin (contractile protein of muscles) 6-N-methyllysine Formula ○ In the structure of prothrombin (blood- clothing protein), Ca2+ binding proteins Gamma-Carboxyglutamate Formula ○ In the structure of elastin (fibrous protein in connective tissue) Desmosine ○ In the structure of some proteins Selenocysteine Formula ○ Found in Urea Cycle Ornithine One less methylene group compared to lysine! Formula Citrulline Formula Other important bio active compounds derived from amino acids ○ Aminobutryic acid GABA Main inhibitory transmitter in CNS Formula ○ Dihydroxy phenylalanine DOPA Precursor to catecholamines Dopamine Epinephrine Norepinephrine Formula ○ Serotonin Monoamine neurotransmitter CNS and GI tract Formula ○ Thyroxine T4 Thyroid hormone Formula § Ionization of Amino Acids Ionization of Amino Acids ○ At acidic pH, the carboxyl group is protonated and the amino acid is in the cationic form. ○ At neutral pH, the carboxyl group is deprotonated but the amino group is protonated. The net charge is zero; such ions are called Zwitterions. Zwitterions=2 ○ At alkaline pH, the amino group is neutral –NH2 and the amino acid is in the anionic form. ○ Example ○ § Amphoteric Nature of Amino Acids Definition ○ Amino acids are amphoteric molecules, meaning they can act as both acids and bases depending on the pH of the environment. This property is due to the presence of both an amino group (-NH₂), which can accept protons, and a carboxyl group (-COOH), which can donate protons. Step-by-Step Process ○ 1. Basic Structure of Amino Acids: Contains an amino group (-NH₂) and a carboxyl group (-COOH). At a neutral pH, the carboxyl group donates a proton (H⁺), becoming negatively charged (COO⁻), while the amino group accepts a proton, becoming positively charged (NH₃⁺). ○ 2. Amino Acids as Acids At a high pH (alkaline conditions), the amino acid acts as an acid. The carboxyl group (-COOH) loses a proton, resulting in a negatively charged carboxylate ion (-COO⁻). ○ 3. Amino Acids as Bases: At a low pH (acidic conditions), the amino acid acts as a base. The amino group (-NH₂) gains a proton, resulting in a positively charged ammonium ion (-NH₃⁺). ○ 4. Zwitterion Formation: In neutral pH (around physiological pH), amino acids exist as zwitterions. The carboxyl group is deprotonated (-COO⁻), and the amino group is protonated (-NH₃⁺), giving no overall charge but both positive and negative charges within the molecule. ○ 5. Isoelectric Point (pI): The pH at which an amino acid exists in its zwitterionic form, with no net electrical charge. At the isoelectric point, the amino acid is least soluble in water and will not migrate in an electric field. ○ 6. Behavior in Acidic and Basic Environments: In acidic conditions (pH < pI), the amino acid carries a net positive charge. In basic conditions (pH > pI), the amino acid carries a net negative charge. ○ 7. Relevance in Protein Structure and Function: The amphoteric nature of amino acids affects protein folding, structure, and interaction with other molecules, crucial in biological processes. § Amino acids can act as buffers in solution Amino acids with uncharged side chains, such as glycine, have two pKa values: ○ the pKa of the alpha-carboxyl group is 2.34 ○ the pKa of the alpha-amino group is 9.6 It can act as a buffer in two pH regimes: ○ PI=(pK1+pK2)/2 § Amino acids carry a net charge of zero at a specific pH (the pI) Zwitterions predominate at pH values between the pKa values of the amino and carboxyl groups For amino acids without ionizable side chains, the Isoelectric Point (equivalence point, pI) is ○ PI=(pK1+pK2)/2 At this point, the net charge is zero ○ AA is least soluble in water ○ AA does not migrate in electric field § Chemical environment in amino acids affects their pKa values Due to simultaneous presence in amino acid structure of both COOH and NH2 groups: ○ Alpha+carboxy group is much more acidic than in carboxylic acids ○ Alpha-amino group is slightly less basic than in amines ○ § Ionizable side chains can show up in titration curves Ionizable side chains can be also titrated ○ Titration curves are now more complex ○ pKa values are discernable if two pKa values are more than two pH units apart ○ ○ To figure out the PK2, find the zwitterion (neutral charge). PK1 is to the left of the zwitterion § General calculation of the pI when the side chain is ionizable How to calculate ○ Identify species that carries a net zero charge ○ Identify pKa value that defines the acid strength of this zwitterion ○ Identify pKa value that defines the base strength of this zwitterion ○ Take the average of these two pKa values ○ Example § Formation of Peptides and Proteins Peptides are short chains formed by the condensation of amino acids. ○ Peptides are “small” compared to proteins. ○ Proteins have a molecular weight (Mw) greater than 10 kDa, whereas peptides are smaller. Peptide Bond Formation ○ A peptide bond forms through a condensation reaction between the carboxyl group (-COOH) of one amino acid and the amino group (-NH₂) of another. This process releases a molecule of water (H₂O). Energy Requirement ○ Peptide bond formation requires energy because the equilibrium favors hydrolysis (breaking down of the peptide bond). ○ Synthesis of peptides and proteins in living organisms requires energy input (e.g., ATP). Peptide Hydrolysis ○ Though the equilibrium favors hydrolysis, the rate of hydrolysis is extremely slow, making peptide bonds kinetically stable. Without a catalyst, such as enzymes (e.g., proteases), a peptide bond in an aqueous environment would remain intact for over 1,000 years. § Peptide nomenclature Numbering (and naming) starts from the amino terminus § Ionization of Peptides Peptide Ionization: ○ Peptides can ionize due to the presence of ionizable groups, such as amino groups (-NH₂), carboxyl groups (-COOH), and sometimes ionizable side chains in certain amino acids (e.g., lysine, histidine, glutamic acid). Ionizable Groups: ○ N-terminus (amino group, -NH₂): Can be protonated to form -NH₃⁺, contributing a positive charge. ○ C-terminus (carboxyl group, -COOH): Can lose a proton to form -COO⁻, contributing a negative charge. ○ Side chains: Certain amino acids (e.g., glutamic acid, lysine) have side chains that can either gain or lose protons, influencing the overall charge of the peptide. pH Dependence: ○ The ionization state of a peptide depends on the pH of the environment: ○ Low pH (acidic conditions): Peptides tend to be protonated, with both N- and C-terminus groups likely to carry positive charges. ○ High pH (basic conditions): Peptides tend to deprotonate, with carboxyl groups carrying negative charges. Isoelectric Point (pI): ○ The isoelectric point is the pH at which the peptide carries no net charge. ○ At the pI, the peptide is least soluble in water and will not migrate in an electric field. Charge Calculation: ○ The overall charge of a peptide at a given pH can be calculated by considering the pKa values of its ionizable groups. ○ Below the pI, the peptide will have a net positive charge; above the pI, it will have a net negative charge. Titration of Peptides: ○ During titration, ionizable groups in a peptide gain or lose protons at specific pH values, affecting the charge and reactivity of the peptide. ○ Titration curves can show the pKa values of the N-terminus, C-terminus, and any ionizable side chains. Relevance to Protein Function: ○ The ionization state of peptides influences their structure, solubility, and interaction with other molecules, playing a crucial role in biochemical processes and protein folding. § Conjugated Proteins Some proteins contain other chemical compounds besides amino acids ○ cofactors functional non-amino acid component e.g. metal ions or organic molecules (coenzymes) ○ coenzymes organic cofactors e.g. NAD+ in lactate dehydrogenase ○ prosthetic groups covalently attached cofactors e.g. heme in myoglobin ○ Other modifications Classes of conjugated proteins ○ Lipoproteins prosthetic group: lipid Example: β1-Lipoprotein of blood ○ Glycoproteins prosthetic group: Carbohydrates Example: Immunoglobulin G ○ Phosphoproteins Prosthetic group: Phosphate groups Examples: Casein of milk ○ Hemoproteins prosthetic group: Heme (iron porphyrin) Examples: Hemoglobin ○ Flavoproteins Prosthetic group: Flavin nucleotides Examples: Succinate dehydrogenase ○ Metalloproteins Prosthetic groups: Iron,Zinc,Calcium, Molybdenum, Copper Examples: Ferritin, Alcohol dehydrogenase, Calmodulin, Dinitrogenase, Plastocyanin § Separation and purification of proteins Source of proteins diverse (tissue, individual cells) → lysis to generate a crude extract ○ Separation of proteins from crude extracts relies on differences in physical and chemical properties: Charge Size Affinity for a ligand Solubility Hydrophobicity Thermal stability ○ Chromatography is commonly used for preparative separation § Electrophoresis for Amino Acid or Protein Analysis Separation in analytical scale is commonly done by electrophoresis ○ Electric field pulls amino acids or proteins according to their charge ○ Gel matrix hinders mobility of proteins according to their size and shape An amino acid placed in an environment with a pH below its pI will have a net (+) charge and move toward the negative electrode (cathode), ie pI = 9.7 in a pH of 7.0. ○ (At a pH of 9.7, this amino acid will not migrate toward either electrode, while at pH of 13 it will have a net (-) charge Key rule to remember ○ if working pH > pI, then protein or amino acid charge is negatively charged ○ if working pH < pI, then protein or amino acid charge is positively charged Steps of electrophoresis ○ Sample Preparation: Amino acid or protein samples, along with molecular weight (MW) markers and controls (purified proteins), are prepared in a suitable buffer. The samples are then loaded into the wells of a gel matrix, commonly polyacrylamide in SDS-PAGE for proteins, or agarose for DNA/RNA. ○ Application of Electric Field: An electric field is applied ac