GE-109 Satellite Geodesy Topic 8 PDF
Document Details
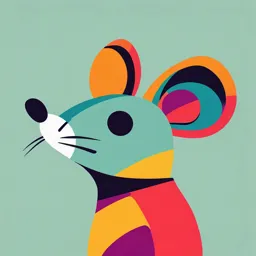
Uploaded by EasyCotangent3334
Caraga State University
Engr. Jaymond T. Verzosa
Tags
Summary
This document provides lecture notes on satellite gravimetry, covering topics such as satellite missions (CHAMP, GRACE, and GOCE), satellite tracking methods, and applications to solving Earth's gravity field. The document also details historical accounts and expected outcomes for the lecture.
Full Transcript
GE 109 – Satellite Geodesy Topic 8: Satellite Gravimetry Lecturer: Engr. Jaymond T. Verzosa Department of Geodetic Engineering College of Engineering and Geosciences Caraga State University Authors/Contributors* This material was prepared/contributed by the following faculty members: Name...
GE 109 – Satellite Geodesy Topic 8: Satellite Gravimetry Lecturer: Engr. Jaymond T. Verzosa Department of Geodetic Engineering College of Engineering and Geosciences Caraga State University Authors/Contributors* This material was prepared/contributed by the following faculty members: Name Type of Contribution Date of Contribution/Revision Kendel P. Bolanio Established (First Version) 11/22/2021 *If this material was edited, revised or updated, kindly fill-up the table with required details accordingly. Copyright Notice Copyright © 2020-2021 by All Authors/Contributors and Department of Geodetic Engineering, College of Engineering and Geosciences, Caraga State University, Butuan City. All rights reserved. Unless otherwise indicated, all materials on these module are copyrighted by the entities indicated above. No part of this module maybe reproduced, stored in a retrieval system, or transmitted in any form or by any means, without either the prior written permission or authorization of Authors/Contributors and the Department of Geodetic Engineering, College of Engineering and Geo-Sciences, Caraga State University, Butuan City. IMPORTANT: The PowerPoint file shall never be distributed to other parties (including students). Only a PDF of this file shall be distributed to students of the course only. Distribution of the PDF to other parties except students should have prior permission or authorization of Authors/Contributors and the DGE of CEGS, CSU. Presentation Outline History Gravity Field of the Earth Satellite Missions: CHAMP, GRACE, and GOCE High-Low, Low-Low Satellite-to-Satellite Tracking, Gradiometry Applications to Solution of Earth’s Gravity Field Expected Outcomes At the end of this lecture, the students would be able to: Discuss the historical accounts for satellite gravimetry; Enumerate and explain the different satellite gravimetric missions; Introduce various satellite tracking methods; Introduce the brief concept of gradiometry; and Discuss the applications of satellite gravimetry to the Earth's gravity field. Part 1 History Gravity Field of the Earth Satellite Missions: CHAMP, GRACE, and GOCE High-Low, Low-Low Satellite-to-Satellite Tracking, Gradiometry Applications to Solution of Earth’s Gravity Field Pioneering Phase of Satellite Gravimetry Today, Helmert’s classical definition of geodesy (Helmert, 1880) could perhaps be reformulated as follows: ▪ "Geodesy is the science of determining the geometric and gravimetric figure of the Earth and its orientation and how these properties change over time". The determination and analysis of the temporal changes of the geometric and gravimetric figure of the Earth and the Earth orientation are moving into the center of geodetic research. Satellite gravimetry – determination of the Earth’s gravitational field October 4, 1957 Source: Flechtner et al., 2021. Satellite Gravimetry: A Review of Its Realization. Surveys in Geophysics (2021) 42:1029-1074 Very First Developments 4 October 1957 – With the entry into the space age, Sputnik-1 was put into orbit by the Soviet Union — satellite gravimetry also began. 3 November 1957 – Already from the very sparse radio signals of Sputnik-1 and Sputnik-2, it was possible to determine the flattening of the Earth, much more accurately than from the preceding 150 years of classical geodetic triangulation networks on Earth. 1969 – A milestone in the development of satellite gravimetry was the Williamstown conference. ▪ Formulate a future program entitled “Solid Earth and Ocean Physics— Applications of Space and Astronomic Techniques” October 4, 1957 Source: Flechtner et al., 2021. Satellite Gravimetry: A Review of Its Realization. Surveys in Geophysics (2021) 42:1029-1074 Gravity Field Modelling in the 1970s to 1990s In the following years, more and more satellites became available for the reconstruction of the gravity field. ▪ 1975 – Starlette ▪ 1976 – LAGEOS 1 ▪ 1986 – AJISAI ▪ 1989 – ETALON-1 and ETALON-2 ▪ 1992 – LAGEOS 2 ▪ 1993 – STELLA ▪ 1995 – GFZ-1 ▪ 2012 – LARES October 4, 1957 Source: Flechtner et al., 2021. Satellite Gravimetry: A Review of Its Realization. Surveys in Geophysics (2021) 42:1029-1074 Gravity Field Modelling in the 1970s to 1990s Procedure Arrangement Quality Path Accuracy Advantage Disadvantage Camera Satellite against 1–2” 1–2” First exact Refraction star background (10 m) Monitoring system SLR Two-way distance 0.5 cm 2 cm Very accurate Depending on weather station distribution Radar S-Band Two-way 1m 5m Weather Single Frequency distance/change 0.3 cm/s 1 cm/s independent System (ionosphere) TRANET Satellite to Ground 0.2 cm/s 0.7 cm/s Good Inaccurate Clocks Distance Station distribution Ionosphere DORIS One-way range 0.4 mm/s 0.5 mm/s Good station Only one ground (ground-to- distribution station at a time satellite) High accuracy Only a few Weather satellites independent Source: Flechtner et al., 2021. Satellite Gravimetry: A Review of Its Realization. Surveys in Geophysics (2021) 42:1029-1074 Gravity Field Modelling in the 1970s to 1990s Procedure Arrangement Quality Path Accuracy Advantage Disadvantage PRARE Two-way 2–3 cm 3–4 cm High accuracy Only a few range/range rate 0.1 mm/s 0.2 mm/s Weather satellites (Satellite— independent Limited number of Ground—Satellite) stations user stations simultaneously Satellite central data provision TDRSS Two-/four-way 1 m (biased) 2m Global coverage Large orbit errors range/range-rate 0.8 mm/s Good accuracy Weak link with reference frame GNSS Pseudorange/phase 1–2 cm 1–2 cm High accuracy Striping for low-low SST high-low (GNSS to Satellite) Continuous orbit SST tracking Source: Flechtner et al., 2021. Satellite Gravimetry: A Review of Its Realization. Surveys in Geophysics (2021) 42:1029-1074 Gravity Field Modelling in the 1970s to 1990s Procedure Arrangement Quality Path Accuracy Advantage Disadvantage Altimetry Two-way range 1–2 cm 2 cm Precise scanning of Modelling near the (Satellite to sea) Ice and oceans coast SST low-low Pseudorange 1 μm 1–2 cm Excellent accuracy Striping (Satellite to Time resolution Satellite) Gradiometry Gravity Gradients 10 mE 1–2 cm High spatial Very complex resolution system Source: Flechtner et al., 2021. Satellite Gravimetry: A Review of Its Realization. Surveys in Geophysics (2021) 42:1029-1074 What is “Gravity” in Fact? The term "gravity" has usually three meanings in geodesy as the weight of a body, the intensity of the gravity field and the acceleration of the free fall. Gravity force acting on a body in static equilibrium at the earth’s surface is defined in a reference system rotating with the earth usually as the resultant 𝐹Ԧ 𝑔 of the Newtonian (or gravitational) mass attraction 𝐹Ԧ 𝑁 between the earth's masses and the body and of the centrifugal force 𝐹Ԧ 𝑅 of the earth's rotation, as 𝑙Ԧ Ԧ Ԧ Ԧ 𝐹 𝑔 = 𝐹 𝑁 + 𝐹 𝑅 = 𝐺 න 3 𝑑𝑚 + 𝜔2 𝑝Ԧ 𝑚 𝑙 Where: G → Newton's gravitational constant (the gravitational force acting between two unit masses from the unit distance) 𝑙Ԧ → the distance vector between the body and the mass elements dm of the earth body 𝜔 → the angular velocity of the earth's rotation 𝑝Ԧ → the distance of the body from the spin axis of the earth m → the mass of the body. October 4, 1957 Source: Rummel, R. and Hipkin, R., 1989. Gravity, Gradiometry, and Gravimetry, Symposium No. 103, International Association of Geodesy Symposia Test Mass in Free Fall Gravitation, the story of the falling apple October 4, 1957 Source: R Westfall: The life of Isaac Newton, Cambridge Univ Press, 1999 [Compare page 51 and 305] Test Mass in Free Fall The size of 𝑥0 ; 𝑡0 gravity from measuring position and time 𝑥1 ; 𝑡1 𝑥2 ; 𝑡2 October 4, 1957 Source: R Westfall: The life of Isaac Newton, Cambridge Univ Press, 1999 [Compare page 51 and 305] Test Mass in Free Fall Absolute Gravimeter FG5 at TU Hannover October 4, 1957 Source: Rummel, R. Lecture 3 - Satellite Gravimetry. 5th ESA Earth Observation Summer School. Part 2 History Gravity Field of the Earth Satellite Missions: CHAMP, GRACE, and GOCE High-Low, Low-Low Satellite-to-Satellite Tracking, Gradiometry Applications to Solution of Earth’s Gravity Field October 4, 1957 October 4, 1957 October 4, 1957 Gravity Field of the Earth (in terms of Gravity Anomalies) Deviation of the gravitational acceleration w.r.t. the gravity on the reference ellipsoidOctober (simplified): The values are between –100 4, 1957 (black) to +100 (white) millionths of the mean gravitational acceleration on the Earth‘s surface (mgal). Individual values are up to three times larger Gravity Field of the Earth (in terms of Geoidal Height) Geographical distribution of the height differences between the Geoid* and the reference October ellipsoid (“bulges” and 4, 1957 “depressions”): The values are between –110 m (dark blue) up to + 90 m (dark red). *Geoid = Equipotential surface of the Earth gravity field which coincides with the undisturbed sea surface) Gravity Field of the Earth (in terms of Geoidal Height) Reasons for the fine structure resp. deviations of the gravity field: - Various mass / density inhomogeneities in the Earth interior (mantle, lithosphere …) and near resp. on the surface - Continental and ocean bottom topography - Ocean currents Geographical distribution of the height differences between the Geoid* and the reference October ellipsoid (“bulges” and 4, 1957 “depressions”): The values are between –110 m (dark blue) up to + 90 m (dark red). *Geoid = Equipotential surface of the Earth gravity field which coincides with the undisturbed sea surface) Gravity Field of the Earth The most common mathematical representation of global gravity field models: ▪ Expressed as expansion (superposition) of spherical harmonic functions ▪ Spherical harmonic functions = three-dimensional spatial waves of spherical symmetry ∞ 𝑙 𝑙 𝐺𝑀 𝑅 𝑉 𝑟, 𝜑, 𝜆 = 𝐶00 + 𝐶𝑙𝑚 𝑃𝑙𝑚 𝑠𝑖𝑛𝜑 𝑐𝑜𝑠𝑚𝜆 + 𝑆𝑙𝑚 𝑃𝑙𝑚 𝑠𝑖𝑛𝜑 𝑠𝑖𝑛𝑚𝜆 𝑟 𝑟 𝑙=1 𝑚=0 ▪ Gravity field determination means estimation of spherical harmonic coefficients Clm and Slm for the gravity field of the Earth ▪ A “Gravity field model” is a data set of spherical harmonic coefficients generated from measurements, which expresses the Earth gravity field in a certain accuracy October 4, 1957 Source: Foerste, C. et al., 2016. On the principles of satellite-based Gravity Field Determination with special focus on the Satellite Laser Ranging technique. 20th International Workshop on Laser Ranging, Potsdam, Gravity Field of the Earth Composition of a gravity field model (gravity anomalies) Example for the successive superposition of spherical harmonics EGM96 (Lemoine et al. 1998) , maximum degree/order 360 → spatial resolution of 50 km at the Earth‘s surface October 4, 1957 October 4, 1957 Gravity Field Determination from Space by using Satellite Basic principle: Evaluation of satellite orbit perturbations ▪ Applied since the launch of the first satellites (1957) Photo-optical satellite tracking = Azimuth/Elevation angle measurements w.r.t. the starry sky ▪ Improved accuracy by Laser tracking systems since ~ 1980 = Satellite Laser Ranging (SLR) ▪ Important “Quantum jump” in the accuracy has been achieved by continuous orbit tracking using GNSS, since the launches of CHAMP (2000 - 2010) and GRACE (seit 2002) = Position measurements (in principle), a few second sampling October 4, 1957 Source: Foerste, C. et al., 2016. On the principles of satellite-based Gravity Field Determination with special focus on the Satellite Laser Ranging technique. 20th International Workshop on Laser Ranging, Potsdam, Gravity Field Determination from Space by using Satellite Gravity field determination using satellites (by evaluation of orbit perturbations) means solving the equation of motion on the basis of precise orbit determination: where fg = Gravitational forces (contains the spher. harm. coefficients) fng = Non-gravitational forces femp = Unknown residual forces October 4, 1957 Source: Foerste, C. et al., 2016. On the principles of satellite-based Gravity Field Determination with special focus on the Satellite Laser Ranging technique. 20th International Workshop on Laser Ranging, Potsdam, Gravity Field Determination from Space by using Satellite Common numeric principles for solving the equation of motion: ▪ Numerical integration (Dynamic approach) ▪ Kinematic orbit determination and taking the obtained positions/velocities as observations for specific algorithms like: o Energy balance approach o Reduced dynamic approach o Short arc approach Estimation of the spherical harmonic coefficients: ▪ Obtained from least squares adjustment ▪ Based on the functional dependency of the satellite orbit from the spherical harmonic coefficients ▪ Includes estimation of empirical parameters to consider the unknown residual forces October 4, 1957 Source: Foerste, C. et al., 2016. On the principles of satellite-based Gravity Field Determination with special focus on the Satellite Laser Ranging technique. 20th International Workshop on Laser Ranging, Potsdam, Part 3 History Gravity Field of the Earth Satellite Missions: CHAMP, GRACE, and GOCE High-Low, Low-Low Satellite-to-Satellite Tracking, Gradiometry Applications to Solution of Earth’s Gravity Field Gravity Satellite Missions Famous recent gravity satellite missions: ▪ CHAMP (2000 – 2010) ▪ GRACE (since 2002) ▪ GOCE (2009 – 2013) October 4, 1957 Source: Foerste, C. et al., 2016. On the principles of satellite-based Gravity Field Determination with special focus on the Satellite Laser Ranging technique. 20th International Workshop on Laser Ranging, Potsdam, The Satellite Mission CHAMP (CHAMP = CHAllenging Minisatellite Payload) Continuous GPS-measurements to the high-altitude GPS satellites (GPS-SST) Measuring of the non-gravitative forces by a 3D accelerometer Additional “classical” SLR tracking from ground October 4, 1957 Allows for much more precise determination of the fine structure of the gravity field than from ground tracking only 2000 - 2010 The Satellite Mission CHAMP A Product of German Reunification Activities German Research Center for Geosciences (GFZ = Deutsches GeoForschungsZentrum Potsdam) – a large-scale research facility established in the course of Germany’s reunification in 1992 German Agency for Space Affairs (DARA)—later integrated into the German Aerospace Center (DLR = Deutsches Zentrum für Luft- und Raumfahrt) – propose a geopotential mission with state-of-the-art observation technology Specifications of the small satellite approach were: fast realization, low costs, ambitious mission objectives. This gravity satellite was named due to the unique combination of novel instruments for the simultaneous detection of gravity and magnetic field, as well as for the sounding of the atmosphere and ionosphere. Source: Flechtner et al., 2021. Satellite Gravimetry: A Review of Its Realization. Surveys in Geophysics (2021) 42:1029-1074 The Satellite Mission CHAMP CHAMP Satellite GeoForschungsZentrum Potsdam Mission life time: 2000 – 2010 October 4, 1957 The Satellite Mission CHAMP Mission Objectives, Satellite Design and Measurement Principle The primary mission goal of the CHAMP mission was a significantly improved determination of the long-wave components (> 800 km) of both the Earth’s gravity and geomagnetic field using innovative instrumentation on board the satellite. Secondary mission goal was the use of the on-board GPS instrumentation for the first operational use of radio occultation technology for remote sensing of the atmosphere and ionosphere. In order to optimize the aerodynamic behaviour and the magnetic field observation environment, the satellite was built as a relatively heavy trapezoidal body, measuring 430 cm × 75 cm × 162 cm (l/h/w), with a 404 cm long fold-out boom in flight direction. The satellite weighed, including two tanks with 34 kg cold gas for attitude control and orbital maneuvers, 522 kg at the beginning of the mission. Source: Flechtner et al., 2021. Satellite Gravimetry: A Review of Its Realization. Surveys in Geophysics (2021) 42:1029-1074 The Satellite Mission CHAMP Mission Objectives, Satellite Design and Measurement Principle Source: Flechtner et al., 2021. Satellite Gravimetry: A Review of Its Realization. Surveys in Geophysics (2021) 42:1029-1074 The Satellite Mission CHAMP Launch, Mission History and Data Provision CHAMP orbit altitude changes 7/2000–7/2010 Source: Flechtner et al., 2021. Satellite Gravimetry: A Review of Its Realization. Surveys in Geophysics (2021) 42:1029-1074 The Satellite Mission CHAMP Characteristics of CHAMP CHAMP CHAllenging Minisatellite Mission Main instrument for gravity Low Earth orbiting satellite with onboard geodetic GPS receiver for high–low SST field recovery Other instruments Digital ion drift meter, overhauser magnetometer, fluxgate magnetometer (for magnetic field recovery) Orbit determination Spaceborne geodetic GPS receiver Orbit control Laser retro reflector Orientation in space Four stellar sensor systems Measurement of non- Electrostatic STAR accelerometer gravitational accelerations Mission duration 15.7.2000–19.9.2010 Orbit height Descending from an altitude of 454 km after launch to 260 km in July 2010, after raising the orbit height twice in July and December 2002 and in addition once in July 2006 and July 2009 in order to maximize mission duration Orbit eccentricity Quasi-circular to frozen orbit Source: Flechtner et al., 2021. Satellite Gravimetry: A Review of Its Realization. Surveys in Geophysics (2021) 42:1029-1074 The Satellite Mission CHAMP Special Features of the CHAMP Mission for Gravity Field Modelling It was the first time that operational and scientific data was collected on board of a geoscientific long-term mission in low orbit, almost continuously (approx. 98 per cent) every second and that all measured quantities were provided with a uniform and accurate (< 1 ms) time stamp. This information was fed into a network of ground system components, realized for the first time in Germany for a geoscientific mission, for the ongoing control of satellite functions and the ongoing monitoring, processing and provision of instrument data and scientific reference products to interested research groups. The various components were developed and operated throughout the entire mission period by (1) the DLR/ GSOC in Oberpfaffenhofen for the satellite operation, (2) the GFZ Potsdam for the scientific data processing, archiving and distribution system, and (3) the DLR/DFD branch office Neustrelitz for raw data archiving and processing. Source: Flechtner et al., 2021. Satellite Gravimetry: A Review of Its Realization. Surveys in Geophysics (2021) 42:1029-1074 The Satellite Mission CHAMP Special Features of the CHAMP Mission for Gravity Field Modelling In addition to the DLR Receiving and Commanding Station Weilheim and the DLR/DFD Receiving Station Neustrelitz, the development and remote operation of a data receiving station on Spitsbergen and a globally distributed network of near- real-time GPS ground stations was promoted by the GFZ for the CHAMP mission. The multi-year continuous data sequence of GPS BlackJack on-board receiver and STAR accelerometer at one-second intervals provided by the CHAMP mission has enabled the continuous determination of exact kinematic satellite positions and the exposure of the purely gravitational signal in the satellite orbit, and thus for the first time the application of so-called two-step or in-situ procedures. Just a few months of continuous high-low-tracking of CHAMP yielded a global gravity field that was superior to the cumulative effort of the 4 decades before. Source: Flechtner et al., 2021. Satellite Gravimetry: A Review of Its Realization. Surveys in Geophysics (2021) 42:1029-1074 The Satellite Mission CHAMP Special Features of the CHAMP Mission for Gravity Field Modelling Gravity field model from 860 days of CHAMP data Source: Flechtner et al., 2021. Satellite Gravimetry: A Review of Its Realization. Surveys in Geophysics (2021) 42:1029-1074 The Satellite Mission GRACE (GRACE = Gravity Recovery and Climate Experiment) Two CHAMP-type satellites (launched in 2002) in an altitude of about 500 km are flying one after another in a distance of about 200 km In addition to GNSS and SLR tracking: October 4, 1957 Ultra precise relative range and range-rate measurements between the satellites (Microwave Ranging System of a few Micrometer accuracy) The Satellite Mission GRACE (GRACE = Gravity Recovery and Climate Experiment) Measuring of differential orbit pertubations between the satellites Higher accuracy in gravity field determination than with single satellites like CHAMP GRACE is sensitive for large scale temporal variations in the gravity field andOctober enables4, 1957 monitoring of mass redistributions on the Earth surface (glacier melting, ground water storage variations…) GRACE Inter-Satellite Ranging Accuracy = few μm (few tenth the diameter of human hairs) October 4, 1957 The GRACE Tandem Mission GRACE NASA + DLR mission (in orbit since 2002) Gravitation from very precise measurement (1μm) of changes of inter satellite distance of two satellites following each other in the same orbit (200 km) October 4, 1957 The GRACE Tandem Mission GRACE: Mission Objectives, Satellites and Measurement Principle GRACE was a satellite mission specifically designed to measure the temporal variations of the Earth’s gravitational field. The main scientific goal was to monitor globally integrated mass changes in the geosphere, which are associated with climate-relevant processes, over a measurement period of several years. The overall system consisting of two identical satellites and the on-board instruments was therefore designed to obtain monthly mean images of the gravitational field, the accuracy of which in this wavelength range should exceed the knowledge gained from the CHAMP mission by a factor of 100 to 1000. The secondary mission objective was the use of GPS radio occultation measurements to obtain density and temperature profiles in the upper atmosphere. Source: Flechtner et al., 2021. Satellite Gravimetry: A Review of Its Realization. Surveys in Geophysics (2021) 42:1029-1074 The GRACE Tandem Mission GRACE mission concept (HAIRS = high accuracy inter-satellite ranging system) Source: Flechtner et al., 2021. Satellite Gravimetry: A Review of Its Realization. Surveys in Geophysics (2021) 42:1029-1074 The GRACE Tandem Mission Distance change in micro-metre between the satellites during an overflight over the Himalayas on 3 May 2003 Source: Flechtner et al., 2021. Satellite Gravimetry: A Review of Its Realization. Surveys in Geophysics (2021) 42:1029-1074 The GRACE Tandem Mission Structure of the GRACE satellites Source: Flechtner et al., 2021. Satellite Gravimetry: A Review of Its Realization. Surveys in Geophysics (2021) 42:1029-1074 The GRACE Tandem Mission Launch, Mission History and Data Products The two GRACE satellites were launched on 17 March 2002 from the Plesetsk Cosmodrome in northern Russia with a ROCKOT launch vehicle, a converted Russian intercontinental rocket SS-19 with a maneuverable BREEZE-KM upper stage. During the 15-year mission period, which was three times longer than originally planned, all instruments on board the GRACE satellites delivered almost continuously measurement and control data for satellite operation and monitoring of the instruments as well as for scientific evaluation. In September 2017, due to the age-related failure of a large number of battery cells on GRACE-2 and outgoing fuel, tandem operation had to be discontinued. Source: Flechtner et al., 2021. Satellite Gravimetry: A Review of Its Realization. Surveys in Geophysics (2021) 42:1029-1074 The GRACE Tandem Mission GRACE orbit altitude change between March 2002 and September 2017 Source: Flechtner et al., 2021. Satellite Gravimetry: A Review of Its Realization. Surveys in Geophysics (2021) 42:1029-1074 The GRACE Tandem Mission Launch, Mission History and Data Products All measurement data obtained onboard GRACE were processed and archived in the GRACE Science Data System (SDS), jointly operated by JPL, CSR and GFZ, to form so-called level-0 (original raw observations) to level-2 (monthly gravity field models in terms of spherical harmonic coefficients) GRACE products. The archiving of the products and supporting documents was performed in the JPL Physical Oceanography Distributed Active Archive Center (PODAAC) and the Information System and Data Center (ISDC) operated at the GFZ. In September 2017, due to the age-related failure of a large number of battery cells on GRACE-2 and outgoing fuel, tandem operation had to be discontinued. Source: Flechtner et al., 2021. Satellite Gravimetry: A Review of Its Realization. Surveys in Geophysics (2021) 42:1029-1074 The GRACE Tandem Mission Statistics of worldwide registered users in the ISDC in early 2018 (left) and annual GRACE publications (right) Source: Flechtner et al., 2021. Satellite Gravimetry: A Review of Its Realization. Surveys in Geophysics (2021) 42:1029-1074 The GRACE Tandem Mission Characteristics of GRACE GRACE Gravity Recovery and Climate Experiment Main instrument for Low Earth orbiting identical satellite pair with K-Band inter-satellite ranging system gravity field recovery HAIRS, low–low SST Other instruments Spaceborne geodetic GPS receiver Separation distance of 220 ± 50 km satellite pair Orbit determination Spaceborne geodetic GPS receiver Orbit control Laser retro reflector Orientation in space Two stellar sensor systems Measurement of non- Electrostatic Super-STAR accelerometer gravitational accelerations Mission duration 17.3.2002–24.12.2017 Source: Flechtner et al., 2021. Satellite Gravimetry: A Review of Its Realization. Surveys in Geophysics (2021) 42:1029-1074 The GRACE Tandem Mission Characteristics of GRACE GRACE Gravity Recovery and Climate Experiment Orbit height Descending from an altitude of 500 km after launch to 345 km in September 2017 Orbit inclination 89° (quasi-polar) Orbit eccentricity quasi-circular Source: Flechtner et al., 2021. Satellite Gravimetry: A Review of Its Realization. Surveys in Geophysics (2021) 42:1029-1074 The GRACE Tandem Mission Time-Variable Gravity Field Models The most important evaluation goal of the GRACE mission was the calculation and rapid provision of time-variable gravity field models. These time series of the three evaluation teams, which are important for further interpretation and evaluation, were provided in the form of so-called GRACE Level 2 products, i.e. as a set of spherical harmonic coefficients describing the Earth’s gravity potential for a certain time period and a spatial resolution. Time series of monthly gravity fields are available in the versions RL01 to RL06. Source: Flechtner et al., 2021. Satellite Gravimetry: A Review of Its Realization. Surveys in Geophysics (2021) 42:1029-1074 The GRACE Tandem Mission Gravity field anomalies expressed in terms of equivalent water height (EWH) with unit cm and DDK3 filtered for the month 2003/08 for all GFZ GRACE releases so far: RL01 (top left), RL02 (top middle), RL03 (top right), RL04 (bottom left), RL05 (bottom middle), and RL06 (bottom right). Source: Flechtner et al., 2021. Satellite Gravimetry: A Review of Its Realization. Surveys in Geophysics (2021) 42:1029-1074 The GRACE Tandem Mission Climate-Relevant Applications Groundwater Monitoring ▪ smallest mass changes observed with GRACE can now help to document the overexploitation of groundwater resources Flood Events and Crisis Management ▪ Calculated daily solutions in near-real time (< 2 days) and derived moisture indicators that are needed to predict the origin and development of flood events in large river systems Polar Ice Sheets ▪ How fast the total mass of ice sheets changes in these areas in order to better understand the fluctuations in sea level worldwide Source: Flechtner et al., 2021. Satellite Gravimetry: A Review of Its Realization. Surveys in Geophysics (2021) 42:1029-1074 The GRACE Tandem Mission Climate-Relevant Applications High-Mountain Glaciers ▪ GRACE data also indicate the mass loss of glaciers in many high mountain regions of the world. ▪ This loss of water is accompanied by a threat to the water supply of the areas downstream of the mountains and the danger of glacial lake outburst floods (GLOFs). Sea Level and Ocean Dynamics ▪ GRACE data can be used to find out whether the (temperature-related) expansion of water, or melting ice, or the influx of water from land has a greater effect on these changes Source: Flechtner et al., 2021. Satellite Gravimetry: A Review of Its Realization. Surveys in Geophysics (2021) 42:1029-1074 The GRACE Tandem Mission GRACE: measures tiny changes of gravitational acceleration Source: Rummel, R. Lecture 3 - Satellite Gravimetry. 5th ESA Earth Observation Summer School. The GRACE Tandem Mission GRACE measures temporal gravitational changes example: seasonal changes of continental hydrology Source: Rummel, R. Lecture 3 - Satellite Gravimetry. 5th ESA Earth Observation Summer School. October 4, 1957 The Satellite Mission GOCE The GOCE Measurement Principle: The acceleration differences along each axis can be taken as second derivatives of the gravitational potential (gravity gradient) in the respective direction. This measurement principle means the direct measurement of a functional of the Earth gravity field (instead of the indirect gravity measurements via evaluation of orbit perturbations). Remark: ▪ From theoretical point of view: A free-floating accelerometer proofmass outside the satellite‘s center of mass can be seen as a small satellite flying in a slightly deviating orbit ▪ Satellite gravity gradiometry is nothing else than measuring differential orbit perturbations (but on a different scale as GRACE) Source: Foerste, C. et al., 2016. On the principles of satellite-based Gravity Field Determination with special focus on the Satellite Laser Ranging technique. 20th International Workshop on Laser Ranging, Potsdam, The Satellite Mission GOCE GOCE: Mission Objectives and Principle GOCE was the first satellite of the European Space Agency ESA’s "Living Planet" Earth science program. It was launched on 17 March 2009 by a Russian launch vehicle from Plesetsk. The aim of this mission was to measure the gravitational field of the Earth as detailed and accurate as possible. The scientific objectives are primarily in the fields of oceanography and geophysics. GOCE’s mission objective was to measure the global Earth’s gravitational field in unprecedented detail. Source: Flechtner et al., 2021. Satellite Gravimetry: A Review of Its Realization. Surveys in Geophysics (2021) 42:1029-1074 The Satellite Mission GOCE GOCE: Mission Objectives and Principle The inner workings of the satellite GOCE—in the centre the gravitational gradiometer, immediately to the right the star sensors and in the next segment to the right the GPS receiver of European design (Source: ESA) Source: Flechtner et al., 2021. Satellite Gravimetry: A Review of Its Realization. Surveys in Geophysics (2021) 42:1029-1074 The Satellite Mission GOCE Characteristics of GOCE GOCE Gravity and steady-state Ocean Circulation Explorer Main instrument Triaxial gravitational gradiometer Other instruments: Orbit determination Spaceborne geodetic GPS receiver Orbit control Laser reflector Orientation in space Three star-sensors Compensation for air Ion engines resistance Mission duration 17.3.2009–11.11.2013 Orbit height 255 km Starting on 1.8.2012 lowering of the orbit -height in 4 steps by 9 km, 6 km, 5 km and 11 km to a height of 224 km Orbit inclination 96.7° (sun-synchronized) Orbit eccentricity Quasi-circular Source: Flechtner et al., 2021. Satellite Gravimetry: A Review of Its Realization. Surveys in Geophysics (2021) 42:1029-1074 The Satellite Mission GOCE Characteristics of gravity field models DIR5 and TIM5 DIR5 TIM5 Max. degree of spherical 300 280 harmonic series development Degree/order (d/o) Data volume 01.11.09–20.10.13 01.11.09–20.10.13 Gravity Gradients Vxx, Vyy, Vzz, Vxz Vxx, Vyy, Vzz, Vxz Filter method Bandpass ARMA per data segment GOCE SST (GPS) Short Arc Method (d/o 150) GRACE SST (K-Band) Years 2003–2012 GRGS RL03 (d/o) 130) LAGEOS 1 & 2 (SLR) 1985 – 2010 Regularization Spherical cap related to GRACE & Kaula (for a segment near the LAGEOS Kaula for (d/o > 180) zonal coefficients and for d/o > 200) Source: Flechtner et al., 2021. Satellite Gravimetry: A Review of Its Realization. Surveys in Geophysics (2021) 42:1029-1074 The Satellite Mission GOCE Overview of the ESA GOCE gravity field models of the DIR, TIM and SPW series Source: Flechtner et al., 2021. Satellite Gravimetry: A Review of Its Realization. Surveys in Geophysics (2021) 42:1029-1074 The Satellite Mission GOCE Scientific Results Gravity or geoid anomalies are a measure for the imbalance of the Earth masses in the crust, lithosphere and upper mantle. New models of the Mohorovicic (Moho) discontinuity were derived from the gravity field models of GOCE. ▪ The Moho discontinuity is the boundary surface between the Earth’s crust and the mantle and corresponds to the depth of the isostatic mass balance according to the Airy model. Detailed investigations were carried out for South America, parts of Africa and Asia, and Antarctica. ▪ For these regions, GOCE demonstrated that the gravity data available before GOCE is faulty and incomplete. Source: Flechtner et al., 2021. Satellite Gravimetry: A Review of Its Realization. Surveys in Geophysics (2021) 42:1029-1074 The Satellite Mission GOCE Scientific Results In the central part of the Himalayas and in the northern Indian border zone, GOCE gradient data were used for the first time to determine plausible values for the elastic thickness of the lithosphere. Also new is the now available gravity and geoid model of the Antarctic. The combination of GOCE models with GRACE gravity time series succeeded in increasing their spatial resolution of the monthly solutions. For oceanography, GOCE provided for the first time a detailed global geoid model. ▪ Satellite altimetry, another highly successful geodetic satellite technique, has been providing series of measurements of the actual and mean sea level without interruption for more than 20 years. Source: Flechtner et al., 2021. Satellite Gravimetry: A Review of Its Realization. Surveys in Geophysics (2021) 42:1029-1074 The Satellite Mission GOCE GOCE gravity field A global geoid map based on two months of data Source: Rummel, R. Lecture 3 - Satellite Gravimetry. 5th ESA Earth Observation Summer School. The Satellite Mission GOCE GOCE gravity field Map compiled by M.Studinger, LDEO, Using data from Siegert et al. (2005) and NSIDC Source: Rummel, R. Lecture 3 - Satellite Gravimetry. 5th ESA Earth Observation Summer School. Part 4 History Gravity Field of the Earth Satellite Missions: CHAMP, GRACE, and GOCE High-Low, Low-Low Satellite-to-Satellite Tracking, Gradiometry Applications to Solution of Earth’s Gravity Field Satellite-to-Satellite Tracking and Satellite Gravity Gradiometry According to Newton’s law of gravitation, the attraction between two bodies is proportional to the product of their mases and inversely proportional to the square of the distance between them. If a satellite passes above an Earth’s mass inhomogeneity (or anomaly), its trajectory (orbit) has a perturbation, i.e., the satellite position gets closer or further away from the Earth. The lower the satellite, the higher its sensitivity to the gravitational effect caused by the mass inhomogeneity. Source: https://ggos.org/item/satellite-gravimetry/#learn-this Satellite-to-Satellite Tracking and Satellite Gravity Gradiometry The traditional tracking techniques used for satellite positioning (GNSS, SLR and DORIS) allow the precise orbit determination for satellites with altitudes between 800 km and 20,000 km, which in turn allow the determination of the Earth’s gravity field with a resolution of about 500 to 1000 km (half wavelength). To increase this resolution, dedicated high-resolution gravity-field missions have been developed. The main characteristics of these satellite missions are: ▪ Orbit altitude as low as possible (200 to 500 km), ▪ Uninterrupted tracking over large orbital arcs in three spatial dimensions, and ▪ Separation between gravitational and non-gravitational forces (e.g. atmospheric drag, solar radiation pressure, albedo, thrust, etc.) acting on the satellite. Source: https://ggos.org/item/satellite-gravimetry/#learn-this Satellite-to-Satellite Tracking and Satellite Gravity Gradiometry Over the years, geoscientists have realized the great complexity of the Earth and its environment. The knowledge of the gravity potential and its level (geopotential) surfaces have become an important issue. Various positioning and gravity field determination techniques have been designed be geoengineers. Various measurement principles of the gravity field: ▪ Gravity measurements ▪ Astronomical positioning ▪ Satellite laser ranging ▪ Satellite radar altimetry ▪ Satellite-to-satellite tracking ▪ Satellite gravity gradiometry Source: Freeden, W. et al., Satellite-to-Satellite Tracking and Satellite Gravity Gradiometry (Advanced Techniques for High-Resolution Gravitational Field Determination Satellite-to-Satellite Tracking and Satellite Gravity Gradiometry The main observation principles are: 1. Satellite-to-satellite tracking in the high-low mode (SST-HL) – A low Earth orbiting (LEO) satellite is tracked by high orbiting GNSS satellites, relative to a network of ground stations. – The satellite is a probe within the Earth’s gravity field, which can be precisely tracked without interruption. – The observed 3-D accelerations correspond to the gravity accelerations. – The non-gravitational forces acting on the satellite are measured by accelerometers. – An example of this measuring principle is given by the mission CHAMP (CHAllenging Minisatellite Payload). Source: https://ggos.org/item/satellite-gravimetry/#learn-this Satellite-to-Satellite Tracking and Satellite Gravity Gradiometry Satellite-to-satellite tracking in the high-low mode (SST-HL) mode: – Measurement of accelerations of one Low Earth Orbiting (LEO) satellite – The orbit of the satellite is determined using GNSS positioning. – The differences with respect to a reference (unperturbed) orbit allow the determination of gravity field at a spatial resolution of 400 km for the static component and about 4,000 km for monthly solutions. – The accelerometer records the non- gravitational forces. Source: https://ggos.org/item/satellite-gravimetry/#learn-this Satellite-to-Satellite Tracking and Satellite Gravity Gradiometry The main observation principles are: 2. Satellite-to-satellite tracking in the low-low mode (SST-LL): – Two LEO satellites are placed in the same orbit, separated by several hundred kilometres, and the range (distance) between them is measured with the highest possible accuracy. – Basically, the acceleration difference between the two satellites is measured. – This method provides a very much higher sensitivity than SSL-HL. – Examples of this measuring principle are the GRACE (Gravity Recovery and Climate Experiment) and GRACE Follow-On (GRACE-FO) missions. Source: https://ggos.org/item/satellite-gravimetry/#learn-this Satellite-to-Satellite Tracking and Satellite Gravity Gradiometry The main observation principles are: 2. Satellite-to-satellite tracking in the low-low mode (SST-LL): – The two satellites can be considered as one instrument in which a. Variations in the gravity field cause variations in the range between the two satellites: areas of stronger gravity affect the lead satellite first and accelerate it away from the second satellite, b. Range variations are measured by a high-accuracy microwave link or laser-ranging interferometry, c. The observed range variations are corrected for non-gravitational effects by precise accelerometers. Source: https://ggos.org/item/satellite-gravimetry/#learn-this Satellite-to-Satellite Tracking and Satellite Gravity Gradiometry Satellite-to-satellite tracking in the low-low mode (SST-LL) mode: – Measurement of acceleration differences between two Low Earth Orbiting (LEO) satellites – The orbits of the two satellites are determined using GNSS. – The distance between the two satellites is measured with the highest possible accuracy. – The acceleration differences between the two satellites allow the determination of the gravity field with a spatial resolution of about 170 km for the static component and about 300 km for monthly solutions. Source: https://ggos.org/item/satellite-gravimetry/#learn-this Satellite-to-Satellite Tracking and Satellite Gravity Gradiometry The main observation principles are: 3. Satellite Gravity Gradiometry (SGG): – Acceleration differences in all three dimensions are measured directly within a LEO satellite. – One important advantage, compared to SST-HL and SST-LL, is that non- gravitational accelerations are the same for all measurements inside the satellite and hence vanish by differentiation. – An example of this measuring principle is the mission GOCE (Gravity field and steady-state Ocean Circulation Explorer). Source: https://ggos.org/item/satellite-gravimetry/#learn-this Satellite-to-Satellite Tracking and Satellite Gravity Gradiometry Satellite Gravity Gradiometry (SGG): – In-situ measurement of acceleration differences gradients within one Low Earth Orbiting (LEO) satellites – The satellite orbit is determined using GNSS. – The gravity gradients are measured in all three components. – This differential measurement allows a spatial resolution of 80- 100 km for the static gravity field. Source: https://ggos.org/item/satellite-gravimetry/#learn-this Part 5 History Gravity Field of the Earth Satellite Missions: CHAMP, GRACE, and GOCE High-Low, Low-Low Satellite-to-Satellite Tracking, Gradiometry Applications to Solution of Earth’s Gravity Field Applications to Solution of Earth’s Gravity Field Data provided by satellite gravity field missions are essential for the determination of the Earth’s gravity field, the computation of geoid, the vertical datum unification, and for the establishment of a global unified height system such as the International Height Reference System (IHRS). The dedicated gravity field missions CHAMP, GRACE (and GRACE Follow-On) and GOCE have made to a huge improvement to our knowledge of the Earth’s static and time-variable gravity field. These missions have considerably increased the accuracy of the static gravity field by a factor of at least 100 in terms of resolvable spatial scales compared to pre-CHAMP gravity models, which were mainly determined from satellite laser ranging data. Source: https://ggos.org/item/satellite-gravimetry/#learn-this Applications to Solution of Earth’s Gravity Field Global gravity models based on GOCE data offer accuracies at the 2 cm level in terms of geoid height at a resolution of 80 to 100 km. Based on monthly gravity fields determined from CHAMP and in particular, GRACE data, seasonal variations and trends in the Earth’s gravity field can be monitored, providing unique information about relevant mass transport phenomena like water cycle in larger river basins, the melting of ice sheets in Antarctica and Greenland and the associated sea level change, as well as in the ocean current systems. Static global gravity models and time-variable gravity models are available at the International Center for Global Earth Models (ICGEM). Source: https://ggos.org/item/satellite-gravimetry/#learn-this Applications to Solution of Earth’s Gravity Field International Center for Global Earth Models – The determination of Earth’s global gravity field is one of the main tasks of geodesy: it serves as a reference for geodesy itself and provides essential information about the Earth, its interior and its fluid envelope for all geosciences. – ICGEM is one of the five services coordinated by the International Gravity Field Service (IGFS) of the International Association of Geodesy (IAG). – The primary objective of the ICGEM service is to collect and archive all existing static and temporal global gravity field models and provide an online interactive calculation service for the computation of gravity field functionals freely available to the general public. https://ggos.org/item/icgem/ Applications to Solution of Earth’s Gravity Field ICGEM Objectives: With the initiation of IGFS and the commitment for hosting and financial support by German Research Centre for Geosciences (GFZ), the ICGEM service was established in 2003 and comprehends: – collecting and long-term archiving of existing static global gravity field models, solutions from dedicated shorter time periods, and topographic gravity field models, – making the above-mentioned models available on the web in a standardized format, – assigning Digital Object Identifiers (DOIs) to the models, – a web interface for the calculation of gravity field functionals from the spherical harmonic models on freely selectable grids and user-defined points, – a 3-D interactive visualization of the models (geoid undulations and gravity anomalies), https://ggos.org/item/icgem/ Applications to Solution of Earth’s Gravity Field ICGEM Objectives: With the initiation of IGFS and the commitment for hosting and financial support by German Research Centre for Geosciences (GFZ), the ICGEM service was established in 2003 and comprehends: – quality checks of the static gravity field models via comparisons with other models in the spectral domain and w.r.t. GNSS/levelling-derived geoid undulations, – the visualization of surface spherical harmonics as tutorial, – the theory and formulas of the calculation service documented in GFZ’s Scientific Technical Report STR09/02 – manuals and tutorials for global gravity field modelling and usage of the service and scientific journal papers for educational and reference purposes – the ICGEM web-based gravity field discussion forum for questions on ICGEM, its products, and knowledge exchange. https://ggos.org/item/icgem/ Applications to Solution of Earth’s Gravity Field Visualization (geoid) of a global gravity field model. https://ggos.org/item/icgem/ Applications to Solution of Earth’s Gravity Field The Calculation Service – A web-interface to calculate gravity field functionals from the spherical harmonic models on freely selectable grids or at user-defined points with respect to a reference system of the user’s choice is provided. Example of grid and plot generation: EIGEN-6C4 global gravity anomalies. https://ggos.org/item/icgem/ One example from the results obtained with CHAMP, GRACE and GOCE CHAMP (7 years, 2002…2009) GRACE (6 years, 2002…2008) Resolution ~ 300 km Resolution ~ 150 km mgal Source: Foerste, C. et al., 2016. On the principles of satellite-based Gravity Field Determination with special focus on the Satellite Laser Ranging technique. 20th International Workshop on Laser Ranging, Potsdam, List of recent Satellite-only gravity field models Source: http://icgem.gfz-potsdam.de Applications to Solution of Earth’s Gravity Field Purpose of Satellite-only Gravity Field Models: Precise orbit computation for various Earth observation satellites (e.g. SAR-Interferometry, Radar Altimetry and other remote sensing techniques) Oceanography (e.g. ocean currents) Regional gravity field modelling together with terrestrial data Source: Foerste, C. et al., 2016. On the principles of satellite-based Gravity Field Determination with special focus on the Satellite Laser Ranging technique. 20th International Workshop on Laser Ranging, Potsdam, Source: Foerste, C. et al., 2016. On the principles of satellite-based Gravity Field Determination with special focus on the Satellite Laser Ranging technique. 20th International Workshop on Laser Ranging, Potsdam, List of recent Satellite-only gravity field models The development of EGM2008 set a benchmark in high resolution gravity field modelling. All other models are improvements based on EGM2008 by combination of new satellite data (incl. Altimetry) with the up to now unmatched continental gravity data from EGM2008. Purpose of Combined Gravity Field Models: ▪ Regional and global gravity field modelling (e.g. height system(s)) ▪ Geophysical modelling ▪ Oceanography (e.g. ocean currents) Source: Foerste, C. et al., 2016. On the principles of satellite-based Gravity Field Determination with special focus on the Satellite Laser Ranging technique. 20th International Workshop on Laser Ranging, Potsdam, Questions? Department of Geodetic Engineering College of Engineering and Geosciences Caraga State University, Ampayon, Butuan City