FUNDAMENTALS OF PETROLEUM GEOSCIENCES AND ENGINEERING-BY JUDAH.pdf
Document Details
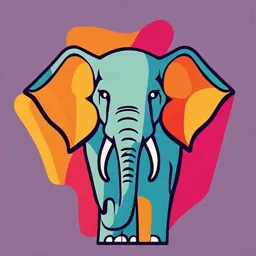
Uploaded by AdorableCircle
2020
Tags
Related
- Official-Gazette-of-the-Petroleum-Industry-Act-2021_PIA_Optimized.pdf
- Petroleum Industry Fiscal Framework: Nigerian Fiscal Regimes
- Petroleum Administration: NNPC PDF
- PIA-2021_Governance and Institutions-Part II (Minister of Petroleum).pdf
- PIA-2021_Petroleum Administration-Part III (Mid and Downstream Petroleum Operations).pdf
- PIA-2021_Petroleum Administration-Part IV (Mid and Downstream Gas Operations).pdf
Full Transcript
Fundamentals of Petroleum Geosciences & Engineering 2020/2021 Academic Year UNIVERSITY OF MINES AND TECHNOLOGY, TARKWA DEPARTMENT OF PETROLEUM GEOSCIENCES AND ENGINEERING FUNDAMENTALS OF PETR...
Fundamentals of Petroleum Geosciences & Engineering 2020/2021 Academic Year UNIVERSITY OF MINES AND TECHNOLOGY, TARKWA DEPARTMENT OF PETROLEUM GEOSCIENCES AND ENGINEERING FUNDAMENTALS OF PETROLEUM GEOSCIENCES AND ENGINEERING (PG 170) LECTURE NOTES Lecturer: Esther Owusu Boateng (Miss) Year One / Semester Two 2020/2021 Compiled by Esther Owusu Boateng Dept. of Petroleum Geosciences and Engineering, UMaT, Tarkwa Page 1 of 91 COMPILED BY JUDAH ANKAMAH Fundamentals of Petroleum Geosciences & Engineering 2020/2021 Academic Year Contents General Information of the Course............................................................................................... 4 Objective................................................................................................................................... 4 Content...................................................................................................................................... 4 Mode of Delivery...................................................................................................................... 4 Reading Material....................................................................................................................... 4 Geosciences.................................................................................................................................. 5 Introduction............................................................................................................................... 5 The Roles of Geoscientists........................................................................................................ 5 Geoscience and the value chain................................................................................................ 6 Exploration........................................................................................................................... 6 Appraisal............................................................................................................................... 6 Development......................................................................................................................... 7 Production............................................................................................................................. 8 Reserve additions and reserves growth................................................................................ 9 Field abandonment and reactivation..................................................................................... 9 GEOSCIENCE ACTIVITY.................................................................................................... 10 Basics of Petroleum Geoscience............................................................................................. 11 Unit Quantities of oil and gas............................................................................................. 12 Numbers of Wells and Fields............................................................................................. 13 Actual reserves discovered and produced.......................................................................... 13 Numbers of basins.............................................................................................................. 15 Rates of additions to reserves............................................................................................. 15 Costs of finding and producing oil and gas........................................................................ 15 Hydrocarbons.............................................................................................................................. 18 Introduction............................................................................................................................. 18 Natural Gases.......................................................................................................................... 19 Hydrocarbon Gases............................................................................................................. 19 Nonhydrocarbon Gases Inert Gases................................................................................... 20 Gas Hydrates........................................................................................................................... 21 Crude Oil................................................................................................................................. 22 Chemistry................................................................................................................................ 22 Paraffins.............................................................................................................................. 23 Naphthenes......................................................................................................................... 23 Aromatics............................................................................................................................ 24 Compiled by Esther Owusu Boateng Dept. of Petroleum Geosciences and Engineering, UMaT, Tarkwa Page 2 of 91 COMPILED BY JUDAH ANKAMAH Fundamentals of Petroleum Geosciences & Engineering 2020/2021 Academic Year Heterocompounds............................................................................................................... 24 Classification........................................................................................................................... 24 Factors that Determine Hydrocarbon Formation........................................................................ 26 Introduction............................................................................................................................. 26 Elements.................................................................................................................................. 27 Source rocks....................................................................................................................... 27 Reservoir rocks................................................................................................................... 42 Traps................................................................................................................................... 47 Seals or cap rocks............................................................................................................... 59 Processes................................................................................................................................. 59 Generation.......................................................................................................................... 59 Migration............................................................................................................................ 59 Accumulation...................................................................................................................... 64 Timing................................................................................................................................ 64 Significance of oil and gas seeps............................................................................................ 65 The Petroleum Play................................................................................................................. 65 The Petroleum Events Chart................................................................................................... 66 Controls on Oil and Gas Occurrence.......................................................................................... 68 Introduction............................................................................................................................. 68 Sedimentary Basins............................................................................................................ 68 Characteristics of Sedimentary Basins............................................................................... 69 Depositional history............................................................................................................ 70 Sedimentary basins and hydrocarbons............................................................................... 71 Basic Concepts and Terms of Sedimentary Basins................................................................. 71 Mechanisms of Basin Formation........................................................................................ 74 Classification of Sedimentary Basins................................................................................. 76 Resources and Reserves: Definition and Tools used for Resource Exploration & Engineering Applications................................................................................................................................ 88 Introduction............................................................................................................................. 88 Classification and Quantification of Reserves........................................................................ 89 Geological methods................................................................................................................ 91 Geophysical methods.............................................................................................................. 91 Geochemical methods............................................................................................................. 91 Other auxiliary methods -....................................................................................................... 91 Compiled by Esther Owusu Boateng Dept. of Petroleum Geosciences and Engineering, UMaT, Tarkwa Page 3 of 91 COMPILED BY JUDAH ANKAMAH Fundamentals of Petroleum Geosciences & Engineering 2020/2021 Academic Year General Information of the Course Objective The course introduces students to the theoretical principles of petroleum geophysics and the steps involved in the use of seismic reflection method for exploring and monitoring petroleum reservoirs. Content Hydrocarbon play concept, composition and origin of petroleum, source rocks, maturation, migration, traps and reservoirs. Outline of exploration and production techniques. The principles related to evaluating potential reservoirs. Introduction to geophysical methods used for resource exploration & engineering applications. Includes basic theory, instrumentation, field techniques, data analysis and case histories. Mode of Delivery Lectures, tutorials, group work and assignments. Reading Material i. Gluyas, J. and Swarbrick, R. (2004), Petroleum Geoscience, Wiley-Blackwell, 376 pp. ii. Keary, P. and Brooks, M. (1991), An Introduction to Geophysical Exploration, Blackwell Scientific Publications, 254 pp. iii. Mussett, A. E. and Khan, M. (2000), Looking into the Earth – An Introduction to Geological Geophysics, Cambridge University Press, Cambridge, United Kingdom, 496 pp. iv. Selley, R. C. and Stephen A. S. (2014), Elements of Petroleum Geology, 3rd Edition, Academic Press, United States, 470 pp. Compiled by Esther Owusu Boateng Dept. of Petroleum Geosciences and Engineering, UMaT, Tarkwa Page 4 of 91 COMPILED BY JUDAH ANKAMAH Fundamentals of Petroleum Geosciences & Engineering 2020/2021 Academic Year Geosciences Introduction Geoscience or Earth Science is a study of the scientific constituents of the earth and its surroundings. This is a branch of science dealing with the physical and chemical constitution of Earth and its atmosphere. Earth science mostly encompasses four main branches of study, the lithosphere, the hydrosphere, the atmosphere, and the biosphere. The Roles of Geoscientists Finding oil and gas – petroleum geoscientists (geophysicists, geologists, and geochemists) work in multidisciplinary teams to decide where to perform seismic imaging (like an ultrasound of the Earth), collect and analyze seismic data, and analyze pre-existing drillhole data from wells to develop a detailed picture of the oil- or gas-rich rocks deep beneath the Earth’s surface. These geoscientists use their knowledge of stratigraphy and sedimentary processes to predict the location and structure of oil- and gas-bearing rocks. Structural geology is used to predict the folding, faulting, and fracturing of rocks in order to interpret the shape of the oil- and gas-rich zones, identify areas where oil and gas may have migrated along faults and fractures, and improve the design of hydraulic fracturing operations. Geochemistry is used to study rock samples and fluids to better understand of the types and amounts of oil and gas present in the rocks. Paleontologists study fossils of ancient organisms, pollen grains, and more to help determine the age of rocks and how they formed. Petroleum geologists work closely with petroleum engineers who must ultimately design how the well will be drilled and prepared for production. Drilling safely and effectively – drilling and preparing a well for production is generally managed by engineers. Geologists help to monitor the drilling by studying rock samples brought up to the surface during the drilling process and analyzing geophysical data obtained by instruments inside the well. Hydrogeologists analyze the risk of ground- and surface water contamination during the drilling and operation of the well, informing decisions about where to position a well and how to reduce the risk from surface and drilling operations. Petroleum Geoscience is intimately linked with making money, indeed profit. The role of the petroleum geoscientist, whether in a state oil company, a massive multinational company, or a small independent company, is to find petroleum (oil and hydrocarbon gas) and help produce it so that it can be sold. Compiled by Esther Owusu Boateng Dept. of Petroleum Geosciences and Engineering, UMaT, Tarkwa Page 5 of 91 COMPILED BY JUDAH ANKAMAH Fundamentals of Petroleum Geosciences & Engineering 2020/2021 Academic Year Geoscience and the value chain The components of the value chain are exploration (commonly divided into frontier exploration and basin exploitation), appraisal, development, production, reserves growth, and field reactivation or abandonment (Figure 1). Geoscientists are employed in each part of this chain. Exploration Almost all the world's petroleum occurs in sedimentary basins—areas that are now, or have at some time in the geologic past, been the sites of sediment accumulation and burial. At the exploration stage the petroleum geoscientist needs to establish as a minimum that the four necessary ingredients are present in the basin: a petroleum source rock, a trap, a seal, and a reservoir. The relative timing of petroleum generation, trap formation, reservoir, and seal deposition also needs to be known. The geoscientist may also need to "know" oil or gas will be produced in the reservoir, since the value of the two can be quite different. In the earliest, frontier, phase of exploration in an unexplored basin, much effort is expended on determining if the basin has petroleum source rocks and whether such source rocks have yielded their petroleum. Naturally, this activity runs in parallel with trying to find the largest structures, those that could contain the greatest quantities of oil and/or gas. If a basin is proved to contain economically viable petroleum pools, then the nature of the work changes to one of basin exploitation. It is during this phase that most of the largest and many of the medium-sized pools are discovered, as the companies involved in exploration begin to understand how, in that particular basin, the petroleum system works. Appraisal Once a petroleum discovery has been made, there can be much uncertainty about its size. The early stages of appraisal are concerned with defining the size and shape of the field and the position of the contacts between fluids (gas/water, oil/water, and possibly gas/oil). Appraisal of a field also starts to reveal the internal architecture of the reservoir and whether the field is divided into compartments, one isolated from another, how reservoir quality (porosity and permeability) varies spatially, and whether the reservoir is layered. At the same time, data are also generated on the variation in petroleum composition across the field. These data and other commercial criteria are then applied to determine whether the field will return sufficient profit when brought into production. Today, a typical field appraisal involves Compiled by Esther Owusu Boateng Dept. of Petroleum Geosciences and Engineering, UMaT, Tarkwa Page 6 of 91 COMPILED BY JUDAH ANKAMAH Fundamentals of Petroleum Geosciences & Engineering 2020/2021 Academic Year the acquisition of a dense grid of seismic data and the drilling of delineation wells. The quality and size of the seismic survey are determined by a number of factors, which include the structural and stratigraphic complexity, the estimated size of the discovery, the environment (marine surveys are much less expensive than land or land/sea transition surveys), the location, and the relative cost of wells versus seismic. The wells drilled during appraisal commonly carry large data acquisition programs. Often, core will be cut from the reservoir section. Some of the core may be preserved at reservoir conditions. Core is used by geoscientists, petrophysicists, and reservoir engineers to determine rock and fluid properties. Such data are used to build a reservoir model and calibrate wells to rock properties to seismic data. The reservoir intervals in wells are also subject to extensive wireline logging, to determine rock types, fluid types, fluid distribution, and seismic properties. Figure 1:Geoscience and the value chain. a) Frontier exploration, (b) Exploration and exploitation (c) Discovery and appraisal and (d) Development and production. Development Information gathered at the appraisal stage is used to construct a development plan—how many wells are needed, where they should be drilled, and what platform facilities are required—and the development plan is executed. The approach to development varies from offshore to onshore and from country to country. However, a very common plan nowadays is to drill the production Compiled by Esther Owusu Boateng Dept. of Petroleum Geosciences and Engineering, UMaT, Tarkwa Page 7 of 91 COMPILED BY JUDAH ANKAMAH Fundamentals of Petroleum Geosciences & Engineering 2020/2021 Academic Year wells while the production facilities are being built. This has the advantage that when the completed facilities are erected on site, the wells can be tied back very quickly, and the field brought onto plateau production almost immediately. The core of the development plan is the reservoir model. The model is used to simulate production of petroleum from the accumulation using computer modeling packages. Each model commonly comprises many tens of thousands of cells. Each cell represents a portion of the reservoir. It is populated with reservoir properties such as net to gross, porosity, permeability, permeability anisotropy, pressure, and the like. Faults and other potentially impermeable barriers are woven through the array of cells. Cells may also have simulated wells, points at which petroleum is extracted from the model or water and/or gas introduced. When the reservoir simulation is running, petroleum is extracted from those cells that contain production wells. Changes in pressure and fluid content are transmitted between cells as the production continues. It is common to run models several times, and the results are used to optimize well location and offtake rate. Reservoir models are commonly conditioned to static data, such as a geologic description of the trap shape, the reservoir architecture, the petrophysical properties, the gas to oil ratio, and the petroleum compressibility. They are also conditioned to any dynamic data that might be available, such as well flow test results. Geologic data continues to be collected and interpreted during the development process. It is used to help refine the reservoir model and thus the way in which petroleum is produced from the field. Production Until the onset of production, the reservoir description has rested almost wholly on "static" data. As production begins, so "dynamic" data are generated. Such data include temporal changes in reservoir pressure and relative and absolute production rates of petroleum and water. These data yield a much greater insight into the internal architecture of the field and, used properly, they can help to improve the reservoir description and so improve the rate and quantity of petroleum production. During the production phase it is probable that the reservoir model will be rebuilt a number of times. First, the reservoir model will begin to fail to predict the performance of the reservoir. Secondly, as production continues a production history will be recorded. This history can be used to assess the success or otherwise of the early models by "history matching" the reservoir model to the real production data. In most oilfields, man needs to help nature when it comes to getting the oil out. Most common amongst secondary recovery processes is the injection of water into the aquifer underlying the Compiled by Esther Owusu Boateng Dept. of Petroleum Geosciences and Engineering, UMaT, Tarkwa Page 8 of 91 COMPILED BY JUDAH ANKAMAH Fundamentals of Petroleum Geosciences & Engineering 2020/2021 Academic Year oil and/or injection of gas (usually methane) into the gas cap or top of the reservoir. For example, in the Beryl Field (UK North Sea) water and gas have been injected to maintain reservoir pressure and sweep oil to the producing wells (Karasek et al. 2003). A large gas cap was created. In a later stage of field development, the secondary gas cap will be produced. Here again, the reservoir description skills of the geoscientist are important. He or she will work with the reservoir engineer to design the best locations for water or gas injection wells. Reserve additions and reserves growth As the rate of petroleum production from a field declines, expensive production equipment becomes underused. As a consequence, work will be undertaken to improve the percentage of petroleum that can be won from the field (reserves additions). Simultaneously, efforts will be made to discover, appraise, and develop additional pools of petroleum that lie near to the original development (reserves growth). At this stage in the value chain, small pools of petroleum can become economically attractive, because the incremental cost of developing them is low if the production facilities exist only a few kilometers away. A geoscientist working on reserves growth can expect to see the whole range of activity from exploration through to production on a highly compressed timescale. Field abandonment and reactivation In most situations, a field will be abandoned when the production rate drops below a predetermined economic limit or, alternatively, the production facilities and wells require investment (repairs) that are deemed not economically viable. There is a tendency for the costs of operating to be reduced with the passage of time for most fields and hence abandonment postponed. However, sooner or later a field will be abandoned. But abandonment may not be the end of the story. Many oilfields are abandoned when the oil remaining in the ground is at least double that which has been produced. The technology available to describe, drill into, and produce the oil today (or in the future) may be that much better than was available during the original life of the field. In consequence, the abandoned field may be selected for reactivation. The appraisal of an abandoned field for rehabilitation is commonly the job of a multidisciplinary team that includes geoscientists and engineers. Compiled by Esther Owusu Boateng Dept. of Petroleum Geosciences and Engineering, UMaT, Tarkwa Page 9 of 91 COMPILED BY JUDAH ANKAMAH Fundamentals of Petroleum Geosciences & Engineering 2020/2021 Academic Year GEOSCIENCE ACTIVITY The specific geoscience activities that are used to support exploration through to abandonment are similar to those used by all geologists and geophysicists, whether working in academia or in industry. The history of geology, at least from the days of William Smith in the 18th century, can be characterized using a series of maps. The primary product from deciphering the stratigraphic column across the globe is the geologic map. Geoscientists working in the oil industry today also spend much time making maps. Such maps can be used to illustrate structure at a range of scales from basin geometry to the geometry of individual traps. Maps are also generated for specific properties, such as net reservoir thickness or porosity. The data used to generate maps comes from several sources. Outcrop mapping on the Earth's surface is not a common activity today, but it still occurs in some parts of the world as a cheap screening process, or where seismic acquisition is difficult. Seismic data provide the main components for map-making in most oil companies. Where seismic data are lacking, maps can be made from well data alone. In order to create maps, there is a range of description and interpretation activities. These include core and outcrop description, structural and stratigraphic work on seismic and well data, paleontological analysis, geochemical analysis of rock and fluid, and a range of petrographic studies. There is one activity that a petroleum geoscientist undertakes which does not have an adequate counterpart in academia. To find and produce commercial quantities of petroleum, wells must be drilled. In most instances it is a petroleum geoscientist, or a team of geoscientists, who will choose the well location. The choice of location will be supported by a prediction of what the well will encounter. This usually takes the form of a predicted stratigraphic column for the well, together with a more detailed description of the target horizon. The detailed description of the target is likely to include the expected rock type, porosity and permeability, whether the well is likely to encounter oil or gas, and whether there is an expectation of penetrating a fluid contact. These data will have been used to estimate the reserves likely to be encountered, and the reserves estimate will justify the expenditure and risk. The success of the well will be judged against the prediction. Although it is a given that petroleum geoscience is about finding oil and gas, a well result that is more positive than predicted is likely to be "forgiven" more quickly than a well that fails to find the petroleum anticipated. Compiled by Esther Owusu Boateng Dept. of Petroleum Geosciences and Engineering, UMaT, Tarkwa Page 10 of 91 COMPILED BY JUDAH ANKAMAH Fundamentals of Petroleum Geosciences & Engineering 2020/2021 Academic Year Basics of Petroleum Geoscience Petroleum (rock oil) is a naturally occurring complex of hydrocarbons widely distributed in the sedimentary rocks of the earth's crust. Though its strict meaning requires that the hydrocarbons occur in liquid form, the simpler compounds among them are gaseous under normal conditions and the more complex ones are commonly found as solids. In the absence of any other widely understood term, "petroleum" should be used for the spectrum of solid, liquid, and gaseous members of the common series. The liquid members constitute crude oil until artificial refinement modifies their original compositions. The gaseous members constitute natural gas. The solid members are variously called asphalt, bitumen, or tar. Neither oil, nor gas, nor solid hydrocarbon satisfies the definition of a mineral, and they should not be called minerals by geologists. Commercially exploitable petroleum occurs underground in the subsurface. The rock containing it is a reservoir rock, or simply a reservoir. The feature of the rock that restrains the fluid petroleum from moving out of the reservoir is called a trap. Petroleum becomes commercially exploitable when it is naturally gathered into a pool, which is a single, discrete accumulation of oil or gas in a single reservoir with a single trap. Several pools may lie in a vertical succession within a single area, or they may lie side by side or overlap laterally so as to constitute a really continuous accumulation called a field. A three-dimensional geological entity containing a number of oil- or gas fields is a sedimentary basin, or simply a basin. A geographical region containing petroleum fields having some geologic characteristics in common is a province. A province may be synonymous with a basin, or it may embrace several basins sharing clear similarities but separated by barren, non-basinal tracts. A geographic concentration of fields within a province or a basin is a district. A small area within a basin, province, or district, which may contain oil or gas but has not yet been proved to do so is a prospect. A larger area within which the drilling of prospects has established success and pointed the way for further drilling provides a play. Oil and gas occurring under these conditions are said to be conventional. They are discovered and exploited by drilling boreholes, or boring drill-holes, into the ground. A hole which yields Compiled by Esther Owusu Boateng Dept. of Petroleum Geosciences and Engineering, UMaT, Tarkwa Page 11 of 91 COMPILED BY JUDAH ANKAMAH Fundamentals of Petroleum Geosciences & Engineering 2020/2021 Academic Year any fluid is a well. A well drilled in search of a new accumulation of oil or gas is an exploratory or wildcat well. If it is successful, it constitutes a discovery. If a discovery well shows promise of being commercial it is completed as a producing well. If a well yields no recoverable oil or gas, it is a dry hole (despite the fact that it may yield a lot of water). A dry hole is abandoned. The process of recording the data derivable from the drilling of a well (whether the recording is done mechanically or as a wholly human operation) is called logging. Oil may occur in natural forms having such high viscosities and densities that they are no longer fluid. Similarly, natural gas in the presence of water may be subjected to such low temperature that it forms a clathrate and becomes effectively a solid. Petroleum which is not sufficiently either liquid or gaseous to be extracted, transported, and used in this conventional manner is said to be unconventional petroleum (or fuel), and to constitute an unconventional resource occurring in unconventional deposits. The term "unconventional" now refers simply to naturally occurring oil or gas deposits of potentially commercial significance not recoverable by conventional drilling methods. Unconventional petroleum resources are of vast extent, but the ready availability of conventional resources has prevented their exploitation until recent years except on a local scale. The search for new sources of petroleum constitutes exploration. The sources discovered by successful exploration become reserves, which are portions of the total resource that have been shown to be accessible and recoverable under current economic and technological conditions. Because no recovery technique can extract all the oil and gas from a field, the reserves of the field are only a fraction of the in-place oil, which the field actually contains. The process of recovering the reserves, by drilling wells within a field and operating them successfully is called development. The technique for exploration is not a function of geology alone but of geophysics also. Petroleum geophysics is so dominated by its seismic branch that the term seismic has almost become synonymous with geophysics for the petroleum industry. Unit Quantities of oil and gas Quantities of oil have traditionally been expressed in barrels. One barrel contains 42 US gallons or 35 Imperial gallons. Many countries including UK and USSR express oil quantities not in terms of volume, but weight: in either tons or metric tonnes of 1000kg. Thus the relation between a tonne and a barrel is not constant but depends on the relative density (specific gravity) of the Compiled by Esther Owusu Boateng Dept. of Petroleum Geosciences and Engineering, UMaT, Tarkwa Page 12 of 91 COMPILED BY JUDAH ANKAMAH Fundamentals of Petroleum Geosciences & Engineering 2020/2021 Academic Year oil. One tonne of average crude oil is 7.33 US barrels (for heavy oil it is 6.8 or less and for light crude 7.6 or more). Quantities of natural gas have always been expressed in terms of volume: cubic feet in North America and cubic meters in most European countries. One barrel of light oil is equivalent to 6,000 or 6,500 cubic feet of natural gas (1 m3 of light oil = 1,000-1,120 m3 of gas). Numbers of Wells and Fields By late 1982, the oil and gas industry had drilled 3.6 million wells. More than 2.8 of these had been drilled in the US alone. The USA has 600,000 producing wells. The whole of the Middle East including Egypt and Syria fewer than 7,000 and Western Europe fewer than 6,000. Typical oil fields in the Persian Gulf produce more than 1000 m3 daily, the average US well produces less than 3 m3 a day. Of the 3.6 million wells that have been drilled, only about 33,000 oil and gas fields were discovered. Of this figure many are small fields that have long been abandoned. Actual reserves discovered and produced. To 1984, the total recoverable, conventional oil discovered in the world was about 2.0 x 1011 m3 (1.2 X 1012 barrels). About 42 percent had been produced, leaving some 1 X 1011 m3 (0.7 x 1012 barrels) as proven, recoverable reserves. The total recoverable natural gas discovered is impossible of estimation because great quantities of it were flared or wasted before its discoverers recognized its value as a fuel in its own right. The cumulative conventional production of gas to 1984 has been about 36 x 1012 m3 (nearly 1300 tcf (trillion cubic feet)). Remaining reserves were then a little less than 100 x 1012 m3, but very much more will certainly be discovered in the future. Table 1.1 provides some vital statistics regarding petroleum production. Table 1: Figures on world’s oil and gas CATEGORY PERCENTAGE COUNTRIES Cumulative oil production to 27 USA and Persian Gulf 1984 15 Soviet Union Compiled by Esther Owusu Boateng Dept. of Petroleum Geosciences and Engineering, UMaT, Tarkwa Page 13 of 91 COMPILED BY JUDAH ANKAMAH Fundamentals of Petroleum Geosciences & Engineering 2020/2021 Academic Year 8 Venezuela 11.5 Canada, Mexico, Libya, Nigeria, and Indonesia Cumulative conventional gas 60 North America production 20 Soviet Union & Eastern Europe Current annual oil production 54 Soviet Union, the USA, Saudi (1984) Arabia, and Mexico (3.2 X 109 m3) 75 USA, the Soviet Union, Canada, and the Netherlands. The USA alone provided more than 50 percent of world oil production Current annual gas until 1950 and much more than production (1984) 1.6 x 1012 50 percent of world gas m3 (55 tcf) production until 1970. Even as this is written, Texas has produced more oil than Saudi Arabia. 55 Saudi Arabia, Kuwait, Iran, Iraq, and Abu Dhabi Remaining known oil reserves 13 Soviet Union and China 8 Mexico 40 Soviet Union 16 Iran Known gas reserves 19 USA, Algeria, Saudi Arabia, and Canada Compiled by Esther Owusu Boateng Dept. of Petroleum Geosciences and Engineering, UMaT, Tarkwa Page 14 of 91 COMPILED BY JUDAH ANKAMAH Fundamentals of Petroleum Geosciences & Engineering 2020/2021 Academic Year Numbers of basins Oil and gas fields, and their output and reserve figures, are conventionally tabulated by country because it is to national treasuries that much of their wealth is contributed. For geologists, oil and gas are better assigned to geological than to political entities. The geological entities from which petroleum is derived are sedimentary basins. Many productive basins lie of course wholly within the boundaries of single countries; many more extend from a single nation's territory on to that nation's continental shelf. Other basins, however, are shared by more than one country; the unique Persian Gulf Basin is shared by 12 producing countries. There are about 700 readily distinguishable basins in the world, and finer subdivision could no doubt increase this number considerably. It has been said that China alone possesses 200 sedimentary basins. Of the world's 700, half have been intensively or partially explored for oil and gas; the remaining 300-400 are essentially unexplored as 1984. About 150 basins are (or have been) commercially productive. Many of these basins are of no more than trivial significance. The highly unequal distribution of reserves between the basins, and between the individual fields in them, is so important that it needs extended explanation. Rates of additions to reserves The number of "ordinary" oilfields discovered each year continues to increase year by year (even in North America). Discoveries of giant fields (those having recoverable reserves of 500 x 10 6 barrels (8 x 107 m3) or more are becoming scarcer, however, and discoveries of super giants (5 x 109 barrels or 8 x l08 m3) have been declining since the 1960s. By far the greatest number of giant oilfields was discovered between 1950 and 1970, especially during the decade of 1955-64, called the heyday of exploration in the Middle East and North Africa. Costs of finding and producing oil and gas. Until the end of the 1960s, the petroleum industry's concern about the costs of finding and producing their products was concentrated on drilling costs. These were quoted as costs per foot (or meter) of hole drilled, and the figures were of the order of US $30-50 m-1. These costs have escalated enormously since the early 1970s, for three elementary reasons. Unit costs of all operations have risen everywhere; Compiled by Esther Owusu Boateng Dept. of Petroleum Geosciences and Engineering, UMaT, Tarkwa Page 15 of 91 COMPILED BY JUDAH ANKAMAH Fundamentals of Petroleum Geosciences & Engineering 2020/2021 Academic Year Exploratory wells are now drilled much deeper than formerly, and much deeper fields are exploited; finally, Drilling and production have been extended into deep waters offshore and into other regions of operational difficulty in high latitudes. Deep drilling costs exceed $300 m-1 and are commonly more than twice that amount. The total cost of drilling the world's wells is now in the realm of $20 X 109 - 50 X 109 annually. Drilling wells, of course, is not the only cost of finding oil or gas. Pre-drill exploration, including both geologic and seismic surveys, has been increasing in cost at about 15 percent per year for a decade or more. In 1981, seismic surveys on land in the non-communist world cost about $3 X 109 annually; marine surveys cost an additional $0.75 x 109. Total seismic costs, including processing of the data, are between $4 x 109 and $5 X 109 annually; one-third of these costs is incurred in the USA. The industry's attention has consequently been shifted from the costs of drilling to the costs of finding oil (or gas). So long as these costs were of the order of $1 per barrel ($6 m -3 approximately), they were of little concern. In the Middle East, the cost of finding oil was measured in cents per barrel. By 1981, the average cost of finding oil in the non-communist world had become almost $9 per barrel ($55 m-3), and this figure can only become larger. Costs of producing oil and gas have risen proportionately. The operation of a single producing well, years after its field has been discovered, runs from hundreds of dollars monthly in the continental USA to tens of thousands monthly in deep waters or polar regions. Costs of production are further complicated by laws governing allowable rates of production. These considerations go beyond the immediate concern of the geologists (until they become members of management committees) With outlays of these magnitudes, however, it is obvious that concentration on the costs of exploration, of drilling wells, of operating wells once drilled, or of any downstream operations (transporting and refining the oil, for example) is futile, though each item is of course of critical individual concern to the industry (and to the consumer). The industry's quantitative fiscal concern is now best expressed, therefore, in terms of the cost per unit of daily production. This cost represents the total capital and operating costs required for the discovery and production of the oil or gas, divided by the volume of production achievable or allowable under the physical, legal, and political conditions governing the operation. The costs considered are technical costs only, and do not include royalties or taxes (though these have to Compiled by Esther Owusu Boateng Dept. of Petroleum Geosciences and Engineering, UMaT, Tarkwa Page 16 of 91 COMPILED BY JUDAH ANKAMAH Fundamentals of Petroleum Geosciences & Engineering 2020/2021 Academic Year be included in the larger, overall "costs of doing business"). The actual figures, of course, vary from region to region for the physical conditions, and from jurisdiction to jurisdiction for the legal and political conditions; they also, again of course, vary with time (though invariably increasing). At the beginning of the 1980s, however, we may consider sources of oil as falling into four unit-cost categories (with costs in 1980 US dollars): (a) Low-cost oil, such as that from the Persian Gulf, Indonesia, or Nigeria. This averages perhaps $3,000 per daily barrel ($2 x 104 m-3 of daily production). In simple terms, the achievement and maintenance of a production rate of 100,000 m3 per day (about 600,000 barrels per day) requires investment of two billion dollars ($2 x 109). (b) Medium-cost oil: Costs average, say, $9,000 per daily barrel. The larger fields in the North Sea or southeastern Mexico, and fields in the deep interiors of continents other than North America or Europe, fall into this category. (c) High-cost oil: Costs average $18,000 per daily. barrel or more. Arctic oil, and oil from the smaller fields in the North Sea or off eastern Canada, will be in this category. Those with difficult individual problems, such as a recalcitrant reservoir rock, are in the $30 000 per daily barrel range. (d) Excessive-cost oil: Most unconventional oil sources require still higher costs per unit of output. The Athabasca tar sands, for example, will require investments variously estimated at $20,000 to more than $50,000 per daily barrel of output. Shale oil may cost between $50,000 and $100,000 per daily barrel. Gas production may be similarly categorized. Gas from the continental USA, western Canada, or onshore western Europe is low-cost gas. Gas from the eastern Canadian offshore is high-cost gas. Gas from the Bering Sea would be of excessive cost. The development of oil or gas resources in any remote, hostile, or deep-water region is conceivable only if output is to be large; a minimum of 50,000 m3 per day in the case of oil, or of 5 X 107 m3 per day in the case of gas. Minimum outlays are therefore in the billions of dollars. There is general recognition that these huge costs will approximately double by the year 2000. Compiled by Esther Owusu Boateng Dept. of Petroleum Geosciences and Engineering, UMaT, Tarkwa Page 17 of 91 COMPILED BY JUDAH ANKAMAH Fundamentals of Petroleum Geosciences & Engineering 2020/2021 Academic Year Hydrocarbons Introduction Petroleum exploration is largely concerned with the search for oil and gas, two of the chemically and physically diverse group of compounds termed the hydrocarbons. Physically, hydrocarbons grade from gases, via liquids and plastic substances, to solids. The hydrocarbon gases are classified as dry gas or wet gas according to the amount of liquid vapour that they contain. A dry gas may be arbitrarily defined as one with less than 0.1 gal/1000 ft3 of condensate; chemically, dry gas is largely methane. A wet gas is one with over 0.3 gal/1000 ft3 of condensate; chemically, these gases contain ethane, butane, and propane. Gases are also described as sweet or sour, based on the absence or presence, respectively, of hydrogen sulphide. Condensates are hydrocarbons that are gaseous in the subsurface but condense to liquid when they are cooled at the surface. Liquid hydrocarbons are termed oil, crude oil, or just crude. The plastic hydrocarbons include asphalt and related substances. Solid hydrocarbons include coal and kerogen. Gas hydrates are ice crystals with peculiarly structured atomic lattices, which contain molecules of methane and other gases. The earth's atmosphere is composed of "natural gas." In the oil industry, however, natural gas is defined as "a mixture of hydrocarbons and varying quantities of nonhydrocarbons that exists either in the gaseous phase or in solution with crude oil in natural underground reservoirs." Natural gas is sub-classified into: Dissolved gas - is in solution in crude oil in the reservoir. Associated gas - is commonly known as gas cap, gas overlies and is in contact with crude oil in the reservoir. Non-associated gas is in reservoirs that do not contain significant quantities of crude oil. Natural gas liquids, or NGLs, are the portions of the reservoir gas that are liquefied at the surface in lease operations, field facilities, or gas-processing plants. Natural gas liquids include, but are not limited to, ethane, pro-pane, butane, pentane, natural gasoline, and condensate. Basically, natural gases encountered in the subsurface can be classified into two groups: those of organic origin and those of inorganic origin (Table 2). Table 2: Natural gases and their dominant modes of formation. Compiled by Esther Owusu Boateng Dept. of Petroleum Geosciences and Engineering, UMaT, Tarkwa Page 18 of 91 COMPILED BY JUDAH ANKAMAH Fundamentals of Petroleum Geosciences & Engineering 2020/2021 Academic Year Natural Gases Hydrocarbon Gases. The major constituents of natural gas are the hydrocarbons of the paraffin series and contain those straight-chain hydrocarbons having the general formula CnH2n+2. The heavier members of the series decline in abundance with increasing molecular weight. Methane is the most abundant; ethane, butane, and propane are quite common; and paraffins with a molecular weight greater than pentane are the least common. Methane (CH4) is also known as marsh gas and is a colourless, flammable gas, which is produced (along with other fluids) by the destructive distillation of coal. It is the first member of the paraffin series. It is chemically non-reactive, sparingly soluble in water, and lighter than air (0.554 relative density). Methane is known to occur as a by-product of bacterial decay of organic matter at normal temperatures and pressures. This biogenic methane has considerable potential as a source, of energy and generated by the maturation of manure. Biogenic methane is commonly formed in the shallow subsurface by the bacterial decay of organic-rich sediments. As the burial depth and temperature increase, however, this process diminishes, and the bacterial action is extinguished. The methane encountered in deep reservoirs is produced by thermal maturation of organic matter. This process will be discussed in detail later under maturation of kerogen. The other major hydrocarbons that occur in natural gas are ethane, propane, butane, and occasionally pentane. Unlike methane these heavier members of the paraffin series do not form biogenically. They are produced only by the thermal maturation of organic matter. If they are Compiled by Esther Owusu Boateng Dept. of Petroleum Geosciences and Engineering, UMaT, Tarkwa Page 19 of 91 COMPILED BY JUDAH ANKAMAH Fundamentals of Petroleum Geosciences & Engineering 2020/2021 Academic Year recorded by a gas detector during the drilling of a well, it often indicates proximity to a significant hydrocarbon reservoir or source rock. Nonhydrocarbon Gases Inert Gases Helium is a common minor accessory in many natural gases, and traces of argon and radon have also been found in the subsurface. Except for helium these gases are of no economic significance. Helium occurs in the atmosphere at 5 ppm and has also been recorded in mines, hot springs, and fumaroles. It has been found in oil field gases in amounts of up to 8 percent. Helium is known to be produced by the decay of various radioactive elements, principally uranium, thorium, and radium. Based on these data, the helium found in natural gas is widely believed to have emanated from deep-seated basement rocks, especially granite. Although the actual rate of production is slow and steady, the expulsion of gas into the overlying sediment cover may occur rapidly when the basement is subjected to thermal activity or fracturing by crustal arching. Nitrogen is another nonhydrocarbon gas that frequently occurs naturally in the earth's crust. It is commonly associated both with the inert gases just described and with hydrocarbons. Several pieces of information suggest that the major source of nitrogen is basement, or more specifically, igneous rock. Taken all together, these various lines of evidence suggest that nitrogen natural gases are predominantly of inorganic origin, although organic processes may be significant generators of atmospheric and shallow nitrogen. Furthermore, some atmospheric nitrogen may have been trapped in sediments during deposition, occurring now as connate gas. Free hydrogen gas rarely occurs in the subsurface, partly because of its reactivity and partly because of its mobility. Nonetheless, one or two instances are known. Hydrogen is commonly dissolved in subsurface waters and in petroleum as traces. Subsurface hydrogen is probably produced by the thermal maturation of organic matter. Carbon dioxide (CO2) is often found as a minor accessory in hydrocarbon natural gases and is associated with nitrogen and helium. Natural gas accumulations in which carbon dioxide is the major constituent are common in areas of extensive volcanic activity. Both organic and inorganic processes can undoubtedly generate significant amounts of carbon dioxide in the earth's crust. It may also be generated where igneous intrusive metamorphose carbonate sediments. Permeable limestones and dolomites can also yield carbon dioxide when they are invaded and leached by acid waters of either meteoric or connate origin. Carbon dioxide Compiled by Esther Owusu Boateng Dept. of Petroleum Geosciences and Engineering, UMaT, Tarkwa Page 20 of 91 COMPILED BY JUDAH ANKAMAH Fundamentals of Petroleum Geosciences & Engineering 2020/2021 Academic Year is a normal product of the thermal maturation of kerogen, generally being expelled in advance of the petroleum. Hydrogen sulphide (H2S) occurs in the subsurface both as free gas and, because of its high solubility, in solution with oil and brine. It is a poisonous, evil-smelling gas, whose presence causes operational problems in both oil and gas fields. It is highly corrosive to steel, quickly attacking production pipes, valves, and flowlines. Gas or oil containing significant traces of hydrogen sulphide are referred to as sour. In contrast, sweet, refers to oil or gas without hydrogen sulphide. Small amounts of hydrogen sulphide are economically deleterious in oil or gas because a washing plant must be installed to remove them: both to prevent corrosion and to render the residual gas safe for domestic combustion. Extensive reserves of sour gas can be turned to an advantage, however, since it may be processed as a source of free sulphur. Hydrogen sulphide is commonly expelled together with sulphur dioxide from volcanic eruptions. Sour gas generally occurs in hydrocarbon provinces where large amounts of evaporites are present. It is also frequently associated with carbonates, generally reefal, and lead-zinc sulfide ore bodies. Gas Hydrates Gas hydrates are compounds of frozen water that contain gas molecules. The ice molecules themselves are referred to as clathrates. Physically, hydrates look similar to white, powdery snow and have two types of unit structure. The small structure holds up to 8 methane molecules within 46 water molecules. This clathrate may contain not only methane but also ethane, hydrogen sulphide, and carbon dioxide. The larger clathrate consists of unit cells with 136 water molecules. This clathrate can hold the larger hydrocarbon molecules of the pentanes and n-butanes. Gas hydrates occur only in very specific pressure-temperature conditions. They are stable at high pressures and low temperatures. Gas hydrates occur in shallow arctic sediments and in deep oceanic deposits. Gas hydrates in arctic permafrost have been described from Alaska and Siberia and occur between about 750 to 3500 m. Hydrates have been found in the sediments of many of the oceans around the world. Gas hydrates have generally been attributed to a shallow biogenic origin. Clathrates can hold six times as much gas as can an open, free, gas-filled pore system. Unfortunately, gas hydrates present considerable production problems, which have yet to be overcome. These problems are due, in part, to the low permeability of the reservoir and, in part, Compiled by Esther Owusu Boateng Dept. of Petroleum Geosciences and Engineering, UMaT, Tarkwa Page 21 of 91 COMPILED BY JUDAH ANKAMAH Fundamentals of Petroleum Geosciences & Engineering 2020/2021 Academic Year to chemical problems concerning the release of gas from the crystals. Clathrate deposits may be of indirect economic significance, however, by acting as cap rocks. Because of their low permeability, they form seals that prevent the upward movement of free gas. Crude Oil Crude oil is defined as "a mixture of hydrocarbons that existed in the liquid phase in natural underground reservoirs and remains liquid at atmospheric pressure after passing through surface separating facilities". In appearance crude oils vary from straw yellow, green, and brown to dark brown or black in colour. Oils are naturally oily in texture and have widely varying viscosities. Oil on the surface tend to be more viscous than oils in warm subsurface reservoirs. Surface viscosity values range from 1.4 to 19,400 centistokes and vary with temperature, age and depth of the oil. Most oils are lighter than water. Although the density of oil may be measured as the difference between its specific gravity and that of water, it is often expressed in gravity units defined by the American Petroleum Institute according to the following formula: 0 API = [141.5 / (specific gravity 60°F /60°F)] - 131.5 where 60°F /60°F is the specific gravity of the oil at 60°F compared with that of water at 60°F. Note that API degrees are inversely proportional to density. Thus, light oils have API gravities of over 40° (0.83 specific gravity), whereas heavy oils have API gravities of less than 10° (1.0 specific gravity). Heavy oils are thus defined as those oils that are denser than water. Oil viscosity and API gravity are generally inversely proportional to one another. Chemistry In terms of elemental chemistry, oil consists largely of carbon and hydrogen with minor amounts of oxygen, nitrogen, and sulphur. Oil also contains traces of vanadium, nickel, and other elements. Although the elemental composition of oils is relatively straightforward, there may be an immense number of molecular compounds. Elemental composition of crude oils by weight % is given below. Table 3: Elemental composition of crude oils by weight % is given below. Element Minimum Maximum Carbon 82.2 87.1 Compiled by Esther Owusu Boateng Dept. of Petroleum Geosciences and Engineering, UMaT, Tarkwa Page 22 of 91 COMPILED BY JUDAH ANKAMAH Fundamentals of Petroleum Geosciences & Engineering 2020/2021 Academic Year Hydrogen 11.8 14.7 Sulphur 0.1 5.5 Oxygen 0.1 4.5 Nitrogen 0.1 1.5 Other Trace 0.1 No two oils are identical either in the compounds contained or in the various proportions present. However, certain compositional trends are related to the age, depth, source, and geographical location of the oil. For convenience, the compounds found in oil may be divided into two major groups: the hydrocarbons, which contain three major subgroups, and the heterocompounds, which contain other elements. Paraffins The paraffins, often called alkanes, are saturated hydrocarbons, with a general formula CnH2n+2. For values of n < 5 the paraffins are gaseous at normal temperatures and pressures (methane, ethane, propane, and butane). For values of n = 5 (pentane, C5HI2) through to n = 15 (pentadecane, C15H32) the paraffins are liquid at normal temperatures and pressures; and For values of n > 15 they grade from viscous liquids to solid waxes. Two types of paraffin molecules are present within the series, both having similar atomic compositions (isomers), which increase in molecular weight along the series by the addition of CH2 molecules. One series consists of straight-chain molecules, the other of branched-chain molecules. The paraffins occur abundantly in crude oil, the normal straight-chain varieties dominating over the branched-chain structures, Individual members of the series have been recorded up to C78Hl58. For a given molecular weight the normal paraffins have higher boiling points than do equivalent weight isoparaffins. The paraffins are the major constituents of hydrocarbon gases. They are also quantitatively significant in light gasoline and kerosene oils, making up to 30 percent and 25 percent of the oil, respectively. With increasing boiling point the paraffin fraction of oils gradually decreases. Naphthenes The second major group of hydrocarbons found in crude oils is the naphthenes, or cycloalkanes. This group has a general formula CnH2n. Like the paraffins they occur in a homologous series Compiled by Esther Owusu Boateng Dept. of Petroleum Geosciences and Engineering, UMaT, Tarkwa Page 23 of 91 COMPILED BY JUDAH ANKAMAH Fundamentals of Petroleum Geosciences & Engineering 2020/2021 Academic Year consisting of five- and six- membered carbon rings termed the cyclopentanes and cyclohexanes, respectively. Unlike the paraffins all the naphthenes are liquid at normal temperatures and pressures. They make up about 40 percent of both light and heavy crude oils. Aromatics Aromatic compounds are the third major group of hydrocarbons commonly found in crude oil. Their molecular structure is based on a ring of six carbon atoms. The simplest member of the family is benzene (C6H6), whose structure is shown in Figure 2.9. One major series of the aromatic compounds is formed by substituting hydrogen atoms by alkane (CnH2n+2) molecules. This alkyl benzene series includes ethyl benzene (C6H5, C2H5) and toluene (C6H5CH3). Another series is formed by straight- or branched-chain carbon rings. The aromatic hydrocarbons include asphaltic compounds. These compounds are divided into the resins, which are soluble in n- pentane, and the asphaltenes, which are not. The aromatic hydrocarbons are liquid at normal temperatures and pressures (the boiling point of benzene is 80.5°C). They are present in relatively minor amounts (about 10 percent) in light oils but increase in quantity with decreasing API gravity to over 30 percent in heavy oils. Toluene is the most common aromatic component of crude oil, followed by the xylenes and benzene. Heterocompounds Crude oil contains many different heterocompounds that contain elements other than hydrogen and carbon. The principal ones are oxygen, nitrogen, and sulphur, together with rare metal atoms, commonly nickel and vanadium. Classification Many schemes have been proposed to classify the various types of crude oils. Broadly speaking, the classifications fall into two categories: those proposed by chemical engineers interested in refining crude oil and hose devised by geologists and geochemists as an aid to understanding the source, maturation, history, or other geological parameters of crude oil occurrence. The first type of classification is concerned with the quantities of the various hydrocarbons present in a crude and their physical properties, such as viscosity and boiling point. Schemes of interest to geologists are concerned with the molecular structures of oils because these may be keys to their source and geological history. A more recent scheme by Tissot and Welte (1978) is based on the ratio between paraffins, naphthenes, and aromatics, including asphaltic compounds (Table 4 and Compiled by Esther Owusu Boateng Dept. of Petroleum Geosciences and Engineering, UMaT, Tarkwa Page 24 of 91 COMPILED BY JUDAH ANKAMAH Fundamentals of Petroleum Geosciences & Engineering 2020/2021 Academic Year Figure 2). The great advantage of this classification is that it can also be used to demonstrate the maturation and degradation paths of oil in the subsurface. Figure 2: Ternary diagram showing the classification of oils proposed by Tissot and Welte (1978). Table 4: Classification of crude Compiled by Esther Owusu Boateng Dept. of Petroleum Geosciences and Engineering, UMaT, Tarkwa Page 25 of 91 COMPILED BY JUDAH ANKAMAH Fundamentals of Petroleum Geosciences & Engineering 2020/2021 Academic Year Factors that Determine Hydrocarbon Formation Introduction Petroleum generation is explained via two sets of observations: geological and chemical. Geological facts Major accumulations of hydrocarbon characteristically occur in sedimentary rocks although continental and deep marine sediments are also petroliferous. There are numerous examples of hydrocarbon accumulations in sandstone and limestone reservoirs, which are totally enclosed above, below and laterally by impermeable rocks. Other geological occurrences. (Commercial accumulations of hydrocarbon have been found in basement rocks). Chemical facts Crude oils differ from recent shallow hydrocarbons. They are normally even-numbered carbon chains (modern hydrocarbons are generally odd- numbered chains) They contain more than 50% light hydrocarbons which are rare or absent in modern sediments. Oil and gas fields are economically viable concentrations of hydrocarbons, the formation of which is controlled by several factors referred to as elements and processes. Petroleum system analysis is the study of how these elements and processes interact to create hydrocarbon-bearing provinces. This is the case for “conventional” accumulations, i.e. those where the hydrocarbons are stored in voids in certain types of rocks in oil and gas fields. Oil and gas fields are commercially significant concentrations of hydrocarbons. Every oil and gas accumulation is the result of the following fundamental elements and processes: Element: Source rock, Reservoir, Traps and, Seal Compiled by Esther Owusu Boateng Dept. of Petroleum Geosciences and Engineering, UMaT, Tarkwa Page 26 of 91 COMPILED BY JUDAH ANKAMAH Fundamentals of Petroleum Geosciences & Engineering 2020/2021 Academic Year Processes: generation, migration and, accumulation. Generation takes place in source rocks. The hydrocarbons then migrate from the source beds and accumulate in permeable formations known as reservoir rocks. Accumulation requires the presence of a trap in the reservoir and, in order to prevent further upward hydrocarbon movement, the reservoir must be covered by an impermeable cover called a seal or cap rock. Source, reservoir, trap and seal are the parameters that control the occurrence of oil and gas accumulations (fields). These parameters are geologically controlled. Elements Source rocks A source rock is a fine-grained sediment that in its natural setting has generated and released sufficient hydrocarbons to form a commercial accumulation of oil and gas. Source rocks are clay or carbonate organic-rich muds deposited under low-energy, anoxic conditions. To function as a source rock, the sediment must contain a minimum of 2% by weight of organic matter. The single most important factor in the generation of hydrocarbons in a source rock is temperature. The action of heat on the organic matter (kerogen) contained in the sediment leads to the formation of oil and gas. For temperature to rise to the required level, the source rock must be buried and attain what is known as the minimum level of maturity. A source rock is a rock containing sufficient hydrocarbon-prone organic matter to generate oil and gas. Source Rocks and Generation of Petroleum In conventional oil and gas fields the hydrocarbons are not indigenous to the reservoir. They are generated from the organic matter in the source rock and migrate into the reservoir. Source rocks are very fine-grained, dark-coloured sediments such as shale or carbonate mudstone. The dark colour is due to the presence of the organic matter. Figures 3 and 4 show an exposure of an organic rich shale. The preservation of the organic matter in the source rock is therefore a key factor and this leads to a discussion of the conditions necessary for the deposition of organic-rich sediments. The requirements are: Compiled by Esther Owusu Boateng Dept. of Petroleum Geosciences and Engineering, UMaT, Tarkwa Page 27 of 91 COMPILED BY JUDAH ANKAMAH Fundamentals of Petroleum Geosciences & Engineering 2020/2021 Academic Year a subaqueous environment. oxic surface waters to support abundant organic life, from which the hydrocarbons are ultimately derived; and stagnant sea-bed conditions, leading to the development of an anoxic water–sediment interface. This is essential, otherwise the organic matter becomes oxidised into CO2 and water and will not be preserved. Figure 5 illustrates the ideal conditions for the preservation of the organic matter and Figure 6 depicts the later stages of the generation, migration and accumulation processes. For stagnant sea-bed conditions to develop, an enclosed environment with restricted circulation is the primary requirement. Identifying and studying modern analogues of such environments are useful in the understanding of sites of ancient source-rock deposition. Such modern analogues include the Mediterranean and Black Seas; both are silled, enclosed basins with restricted access to open waters. Also, evaporation at the eastern end of the basin increases the concentration and density of the water, which sinks and aids the circulation process. Consequently, the Mediterranean is an oxic basin, which means that it has poor organic matter preservation potential. It is not, therefore, a suitable analogue for ancient sites of source-rock deposition.Organic-rich sediments are accumulating here now and the anoxic conditions favour the preservation of the organic matter, making the Black Sea a suitable analogue for ancient sites where source-type rocks were deposited. Figure 7 shows the development of anoxic basins in the central Atlantic following the Late Cretaceous breakup of Africa and South America. Figure 3: Dead or inactive seep. Bitumen-impregnated sandstone, northern Greenland (after Henriksen, 2008). Compiled by Esther Owusu Boateng Dept. of Petroleum Geosciences and Engineering, UMaT, Tarkwa Page 28 of 91 COMPILED BY JUDAH ANKAMAH Fundamentals of Petroleum Geosciences & Engineering 2020/2021 Academic Year Figure 4: Exposure of an organic-rich shale: Lower Jurassic, Yorkshire coast, NE England (photo by M. Ala). Figure 5: Illustration of ideal conditions for the deposition and preservation of organic matter (modified and redrawn from UKOOA). Figure 6: Illustration of geological and thermal effects on a source rock resulting in the generation, migration and entrapment of hydrocarbons (copyright Schlumberger, after AI-Hajeri et al., 2009, used with permission). Compiled by Esther Owusu Boateng Dept. of Petroleum Geosciences and Engineering, UMaT, Tarkwa Page 29 of 91 COMPILED BY JUDAH ANKAMAH Fundamentals of Petroleum Geosciences & Engineering 2020/2021 Academic Year Figure 7: The Black Sea is a present-day anoxic basin with good organic matter preservation potential. Figure 8: Core section, showing the occurrence of organic matter in bands in a mudstone, offshore Vietnam (after Petersen et al., 2014). *TOC: total organic content *HI: hydrogen index, indicative of the amount of hydrogen in the organic matter. *High HI indicates greater oil generation potential. The organic matter often occurs in bands in sediments, as shown in Figure 8. The darker the colour the higher the total organic content, abbreviated to TOC. It is estimated that 10–20% of petroleum is formed directly from the hydrocarbons produced by living organisms. The remaining 80–90% of oil and gas is formed by the thermal alteration of kerogen, the organic matter in sedimentary rocks that is insoluble in organic solvents. The most important factor in the generation of hydrocarbons from kerogen is temperature. For temperature to rise to the required level, the source rock must be buried. As the temperature increases, the kerogen matures. With increasing maturity, the colour of the kerogen changes from yellow to brown, to dark brown and finally black. The changes in colour and the corresponding kerogen maturity Compiled by Esther Owusu Boateng Dept. of Petroleum Geosciences and Engineering, UMaT, Tarkwa Page 30 of 91 COMPILED BY JUDAH ANKAMAH Fundamentals of Petroleum Geosciences & Engineering 2020/2021 Academic Year levels are illustrated in Figure 9. Most authorities agree that the threshold temperature for the onset of maturity is 75−80◦C. Initially, heavy oil is generated followed by light oil, then “wet” gas (oil and gas together) and finally dry gas (Figure 10). The oil- and gas-generation phases of the process are referred to respectively as the oil and gas windows, with an overlapping segment representing wet gas formation. Hydrocarbon generation ceases once the hydrogen content of the kerogen is exhausted. Immature kerogen, Palaeocene shale, Zagros basin, SW Iran (after Ala et al., 1980). Slightly darker than the sample above, but still immature kerogen, Upper Cretaceous shale, Zagros basin, SW Iran (after Ala et al., 1980). Mature kerogen, Lower Cretaceous (Albian) shale, Zagros basin, SW Iran (after Ala et al., 1980). Highly mature kerogen, Lower Cretaceous (Neocomian) shale, Zagros basin, SW Iran (after Ala et al., 1980). Figure 9: Changes in the colour of kerogen with increasing maturity. Compiled by Esther Owusu Boateng Dept. of Petroleum Geosciences and Engineering, UMaT, Tarkwa Page 31 of 91 COMPILED BY JUDAH ANKAMAH Fundamentals of Petroleum Geosciences & Engineering 2020/2021 Academic Year Figure 10: Influence of temperature and depth on hydrocarbon generation, assuming an average geothermal gradient of 30◦ C/km (after Henriksen, 2008). Origin of Petroleum It is now widely accepted that petroleum is formed by the thermal maturation of organic matter. The amount of carbon in the earth’s crust has been estimated to weigh 2.651020g. About 82 % of this carbon is locked up as CO3 in limestone and dolomites. About 18% occur as organic carbon in coal, oil and gas. In certain circumstances organic matter may be buried in sediments and preserved and they may be modified into coal, oil and gas. The major groups of chemicals that occur in organic matter are proteins, carbohydrates, lipids and lignin. The first three are found in plants and animals and variously contain the element hydrogen, carbon, oxygen, nitrogen with some sulphur and phosphorus. Productivity and Preservation of Organic Matter The amount of organic matter buried in sediments is related to the ratio of organic productivity and destruction. Generally, organic matter is destroyed on the earth’s surface and only minor amounts are preserved. The deposition of an organic-rich sediment is favoured by a high rate of production of organic matter and a high preservation potential. These two factors will be discussed in the context of: (a) Marine environments and (b) Continental environments. Organic Productivity and Processes in Marine Environment Compiled by Esther Owusu Boateng Dept. of Petroleum Geosciences and Engineering, UMaT, Tarkwa Page 32 of 91 COMPILED BY JUDAH ANKAMAH Fundamentals of Petroleum Geosciences & Engineering 2020/2021 Academic Year In the sea and on land, all organic matter was originally formed by photosynthesis. The photosynthesizers in the sea are pelagic phytoplankton and benthic algae. Biological productivity of these plants is related to both physical and chemical parameters: Physical - (temperature and light are most important) The amount of light is dependent on depth, latitude and turbidity of the water. Organic productivity is highest in the shallow photic zone and decreases with increasing water depth and decreasing light and temperature. Chemical conditions, which favor organic productivity, include abundance of phosphates and nitrates. These chemicals are essential for the growth of plants and animals. Oxygen is also essential for the animals that form later links in the food chain. Preservation of organic matter is favoured by: Anaerobic bottom conditions and a rapid sedimentation rate and the presence of stratification within the waters from which sedimentation occur creating anoxic conditions. Organic Productivity and Preservation in Continental Environments Only swamp deposits are important because sediments with or without organic matter are unlikely to be preserved in upland environments. Types of kerogen Formation of Kerogen Organic matter generated and preserved undergoes changes. In a steadily subsiding sedimentary basin, the temperature and pressure of the sediments increase. Three main phases of organic matter evolution are recognized (Figure 11). a) Diagenesis - occurs at shallow depths at near-normal temperatures and pressures. It includes both biogenic decay, which is aided by bacteria and abiogenic reactions. Methane, carbon dioxide and water are given off by the organic matter leaving behind a complex hydrocarbon called kerogen. Diagenesis of organic matter results in reduction of its oxygen content, leaving the hydrocarbon ratio largely unaltered. (b) Catagenesis occurs in the deeper subsurface as burial continues and temperature and pressure increase. Petroleum is released from kerogen first as oil and later gas. The hydrogen-carbon ratio declines with no significant change in the oxygen-carbon ratio. Compiled by Esther Owusu Boateng Dept. of Petroleum Geosciences and Engineering, UMaT, Tarkwa Page 33 of 91 COMPILED BY JUDAH ANKAMAH Fundamentals of Petroleum Geosciences & Engineering 2020/2021 Academic Year (c) Metagenesis occurs at high temperatures and pressures like metamorphism. The last hydrocarbons, normally methane is expelled. The H-C ratio declines until only carbon is left in the form of graphite. Porosity and permeability are now negligible. Figure 11: Formation of progressively lighter hydrocarbons with increasing temperature and depth (after Marshak, 2005). Chemistry of Kerogen Kerogen is the intermediate product formed by diagenetic transformation of organic matter (OM) originally enclosed in sediments that gives rise to petroleum. It is a complex mixture of high molecular weight organic materials whose composition depends on the source and environment of the OM. Chemically, kerogen consists of carbon, hydrogen with minor amounts of nitrogen and sulphur with a general composition of the form (C12H12ON0.16)x. It is insoluble in normal petroleum solvents such as carbon bisulphide. This insolubility distinguishes it from bitumen. Kerogen is commonly categorised into four types and these are described briefly below. Type I is derived mainly from algae. It is commonly lacustrine in origin (deposited in fresh water lakes) and has high oil-generation potential. Type I kerogen is essentially algal in origin and has a higher proportion of H relative to O than other types of kerogen. Lipids are the dominant compounds in this kerogen, with derivatives of oils, fats and waxes (H/C 1.65) Compiled by Esther Owusu Boateng Dept. of Petroleum Geosciences and Engineering, UMaT, Tarkwa Page 34 of 91 COMPILED BY JUDAH ANKAMAH Fundamentals of Petroleum Geosciences & Engineering 2020/2021 Academic Year Type II is derived from marine organic matter consisting of animal and plant material. Its oil- generation potential is lower than that of type I but it is still a very important source of hydrocarbons. This is the most common kerogen worldwide. Middle East and northern North Sea source rocks contain type II kerogen. Type II or liptinic kerogen is of intermediate composition and rich in aliphatic compounds (i.e. carbon atoms are in long chains). The original matter consisted of algal detritus in addition to zooplankton and phytoplankton (H/C>1). Type III is derived primarily from humic (terrestrial plant material) organic matter and is gas- prone. It is the dominant kerogen type in deltaic petroleum provinces. Type III or humic kerogen is low in aliphatic compounds but rich in aromatic ones (i.e. cyclic hydrocarbons e.g. benzene). Humic kerogen is produced from lignin of the higher woody plants which if buried as peat undergoes diagenesis to coal (H/C< 0.84). This type of kerogen normally generates gas and little, if any oil. Type IV may have been derived from any source. It is usually recycled, oxidised and largely inert (very little oil and gas generation potential). These different kerogen types follow different maturation pathways. The main changes in the chemical composition of kerogen with increasing maturity are reductions in their H/C and O/C ratios. Measurements of the H/C and O/C ratios are used in the van Krevelen diagram to (a) characterise the kerogen and (b) determine its level of maturity. Figure 12 presents a generic van Krevelen diagram, indicating the general scheme of kerogen evolution and the principal products generated during each stage. Diagenesis refers to the changes occurring at relatively low temperatures while catagenesis is applied to the alterations taking place at elevated temperatures. Oil and gas are formed during the catagenetic phase. Metagenesis encompasses the changes when temperature rises above 225◦C, resulting in the formation of graphite. Figure 4.18 presents an example of a van Krevelen diagram for various kerogen types and their level of maturity. Regional source-rock maturity studies are useful in outlining prospective areas for oil and gas field exploration. Figure 4.19 shows that the overwhelming majority of the hydrocarbon accumulations in the northern North Sea occur in the Viking and Central Grabens, where the Upper Jurassic Kimmeridge clay source rock has reached maturity. In these areas the drilling success ratio has been 1:3, but it drops to 1:30 where the source rock remains immature. From the above it is important to identify the: Compiled by Esther Owusu Boateng Dept. of Petroleum Geosciences and Engineering, UMaT, Tarkwa Page 35 of 91 COMPILED BY JUDAH ANKAMAH Fundamentals of Petroleum Geosciences & Engineering 2020/2021 Academic Year Nature of the organic matter in a source rock to assess its potential for generating hydrocarbons. Quantity of kerogen necessary to generate significant amounts of oil and gas suitable for commercial production. 1500 ppm total organic carbon (TOC) is sometimes taken as the minimum requirement for further exploration of a source rock. Figure 12: A generic van Krevelen diagram, showing the general scheme of kerogen evolution and the principal products generated during each stage. Maturation of Kerogen Kerogen matures and gives off oil and gas during catagenesis. When kerogen is immature, no petroleum is generated, but with increasing maturity, first oil and then gas are expelled. An over- matured kerogen has neither oil nor gas. Figure 13 shows the maturation paths for different types of kerogen. Significant oil generation occurs between 60-120oC and significant gas generation between 120- 225 oC. Above 225 oC kerogen is inert and after expelling hydrocarbons, only graphite remains. The reaction rate generally doubles for each 10oC rise. Petroleum may therefore be generated from old, cool source rocks or young hot ones. The rate of maturation is dependent on temperature, time, and possible pressure. Compiled by Esther Owusu Boateng Dept. of Petroleum Geosciences and Engineering, UMaT, Tarkwa Page 36 of 91 COMPILED BY JUDAH ANKAMAH Fundamentals of Petroleum Geosciences & Engineering 2020/2021 Academic Year The two commonly used maturation indices are: The thermal maturation index (TTI) TTI is calculated from a formula that integrates temperature with the time spent in each temperature (100C increments) as a source rock is buried. A burial curve is used to establish the length of time spent in each temperature increment. Figure 13: Graph showing maturation paths of the three different types of kerogen. The level of organic maturation (LOM). LOM is based on the assumption that reaction rate doubles for each 100C increment of temperature. Oil generation occurs between LOM values of 7 and 13, and gas between 13 and 18. Both TTI and LOM should be used with caution because they assume that geothermal gradient is constant through time, which is seldom true. Estimating the time of hydrocarbon generation The van Krevelen diagram provides information on the type and level of maturity of kerogens but does not indicate the time of the onset of maturity and hence the start of hydrocarbon generation. The positions of the oil and gas windows in terms of time and depth in a given area can be determined by constructing burial history curves, which involve plotting depth of burial against geological time. Figure 14 presents a burial history curve based on data from a well in the Gulf of Suez basin. The object of the exercise is to determine when the source rock, which is of Santonian (Upper Cretaceous) age and contains type II kerogen, became mature and entered the oil window. Compiled by Esther Owusu Boateng Dept. of Petroleum Geosciences and Engineering, UMaT, Tarkwa Page 37 of 91 COMPILED BY JUDAH ANKAMAH Fundamentals of Petroleum Geosciences & Engineering 2020/2021 Academic Year The results show that maturity was reached and oil generation began in the Middle Miocene, about 9 million years ago, at a temperature of about 95◦C and depth of 2,290 m. It ended in the Pliocene, about 2.5 million years ago, at a temperature of around 135◦C. It should be noted, however, that the integration of time and temperature with depth is based on complex equations that involve calculations of a parameter known as the time–temperature index (TTI), which is influenced not only by the thermal characteristics of a given area but also by the type of kerogen associated with the source rocks. In practice, the construction of burial curves is undertaken by using computer software specifically developed for this purpose. Comparing the time of oil and gas generation with the time of trap formation from geological studies is useful in determining whether the traps in a given area are likely to be hydrocarbon-bearing. It is essential that trap formation predate, or at least be simultaneous with, hydrocarbon generation and migration. Finally, it should be mentioned that the ratio of reservoir oil to petroleum generated in-place in a typical basin is estimated to be less than 15%; therefore, a great proportion of the hydrocarbons generated in the source rocks remains therein. This underpins the unconventional shale oil and shale gas production industries — extraction of the hydrocarbons that have remained in the shale source rocks. Figure 14: Burial history curve based on data from a well for the source rock in the Gulf of Suez basin (modified and redrawn from Hunt, 1995). Compiled by Esther Owusu Boateng Dept. of Petroleum Geosciences and Engineering, UMaT, Tarkwa Page 38 of 91 COMPILED BY JUDAH ANKAMAH Fundamentals of Petroleum Geosciences & Engineering 2020/2021 Academic Year Paleothermometers There is an important relationship between temperature and petroleum generation which enables the maturity of kerogen to be estimated. Although the following questions have to be answered: Are there organic rich source rocks in the basin? Are they present in large enough volumes? Are they oil prone or gas prone? It is also necessary to know whether the source rocks have matured sufficiently to generate petroleum or whether they are super-mature and barren. The bottom hole temperature from boreholes indicates the present-day temperature, and this may be considerably lower than that of the past. It is necessary to have a paleothermometer which can measure the maximum temperature to which the source rock was ever subjected. Two main groups of techniques are used for measuring the maximum paleotemperature to which a rock has been heated. 1. Chemical paleothermometers a. Organic i. Carbon ratio ii. Electron spin resonance iii. Pyrolysis b. Inorganic i. Clay mineral diagenesis ii. Fluid inclusions 2. Biological paleothermometers a. Pollen coloration b. Vitrinite reflectance 1) Chemical Paleothermometers Compiled by Esther Owusu Boateng Dept. of Petroleum Geosciences and Engineering, UMaT, Tarkwa Page 39 of 91 COMPILED BY JUDAH ANKAMAH Fundamentals of Petroleum Geosciences & Engineering 2020/2021 Academic Year We consider a few of the methods. a) The carbon ratio method makes use of the fact that as organic matter matures, organic carbon is lost. The result of kerogen maturation is thus a gradual decrease in the proportion of movable organic carbon and a relative increase in the proportion of fixed carbon. The method compares the total organic carbon (CT) in the sample with the residual carbon (CR) after pyrolysis to 900°C. The ratio CR - CT increases with maturation. This paleothermometer is not easy to calibrate, however, because of the variations in the original carbon content in the different types of kerogen and the assumption that their carbon ratios change at the same rate. b) The electron spin resonance (ESR) technique is based on the fact that the atomic fraction of kerogen contains free electrons, whose number and distribution are related to the number and distribution of the benzene rings. These electron characteristics change progressively as the kerogen matures. Kerogen is extracted from the source rock and placed in a controlled magnetic field. The sample is then exposed to microwaves so that the free electrons resonate and, in so doing, alter the frequency of the microwave. An electrometer is used to measure the number, signal width, and location of the resonance point of the electrons. These parameters vary with kerogen maturation. The ESR technique has the advantage of being quick and can be used on minute and impure samples of kerogen. c) Pyrolysis is the heating of kerogen or source rock. This process was developed to produce petroleum from oil shales. It is now an important laboratory technique in source rock analysis. A flame ionization detector is used with kerogen heated in a stream of helium. The temperature is gradually raised at a carefully measured rate, and the expelled hydrocarbon gases recorded. At relatively low temperatures (200 to 300°C) any free hydrocarbons in the sample are volatilised, referred to as S1. With increasing temperature hydrocarbons are expelled from the kerogen, i.e., S2. Carbon dioxide and water, which are also expelled, are grouped as S3. Pyrolysis generally continues up to 800 to 900°C. The three readings are used to determine the maturation level of the source rock. d) Clay mineral analysis is one of the oldest of the inorganic paleo-thermometers, having been studied quite independently of kerogen maturation. Newly deposited clay minerals include smectites (montmorillonite), illite, and kaolin. The varying abundance of different mineral types depends partly on the source area and partly on the depositional environment. As clays are buried, they undergo various diagenetic changes. Of particular interest is the behaviour of the smectites, which dewater and change to illite within the optimum temperature for oil generation Compiled by Esther Owusu Boateng Dept. of Petroleum Geosciences and Engineering, UMaT, Tarkwa Page 40 of 91 COMPILED BY JUDAH ANKAMAH Fundamentals of Petroleum Geosciences & Engineering 2020/2021 Academic Year (80 to 120°C). This phenomenon is considerably important to oil migration. As temperatures increase to the upper limits at which oil and gas form, another major change in clay takes place. Kaolin and illite recrystallize into micas, whereas in ferromagnesian-rich environments they form chlorites. 2) Biological Paleothermometers One of these techniques measures the colour of the organic matter in the source rock. Kerogen has many colours and shades, which are dependent on both maturation and composition. Spores and pollen, however, begin life essentially colourless. As they are gradually heated, they change to yellow, orange, brown (light to dark), and then to black. These colour changes can be related to the degree of maturation of the associated kerogen. Several colour codes are used. One popular system is the 10-point Spore Colour Index; another is the 5-point Thermal Alteration Index. It has been argued that pollen colour measurement is a subjective assessment, but with reference standards and adequate operator experience the results are valid. The last paleothermometer we consider is the vitrinite reflectance. The shininess of coal increases with rank from peat to anthracite. This shininess, or reflectance, is measured optically. Vitrain, the coal maceral (fragment of plant debris in coal) used for measurement, occurs widely, if sparsely, throughout sedimentary rocks. Vitrain is present in kerogen and is extracted and mounted on a slide. A reflecting-light microscope is then used to measure the degree of reflectivity, termed Ro. A number of reflectivity readings are taken for each sample and plotted on a histogram, reference being made to standard samples of known Ro values. Ro may be measured for a series of samples down a borehole, and a graph of reflectivity versus depth may be plotted. An empirical relationship exists between vitrinite reflectance and hydrocarbon generation. Crude oil generation occurs for Ro val