Flame-retardant finishes PDF
Document Details
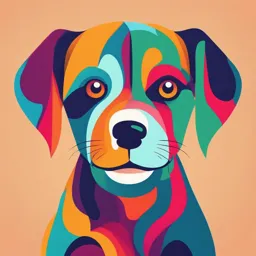
Uploaded by SkillfulAspen
Tags
Summary
This document is a chapter from a book about flame-retardant finishes in textiles, exploring mechanisms of flame retardancy and various techniques used to achieve it. The chapter discusses the chemistry of combustion, endothermic decomposition reactions, and other related aspects.
Full Transcript
8 Flame-retardant finishes 8.1 Introduction Flame-retardant finishes provide textiles with an important performance character- istic. Protection of consumers from unsafe apparel is only one...
8 Flame-retardant finishes 8.1 Introduction Flame-retardant finishes provide textiles with an important performance character- istic. Protection of consumers from unsafe apparel is only one area where flame retardancy is needed. Firefighters and emergency personnel require protection from flames as they go about their duties. Floor coverings, upholstery and drapery also need protection, especially when used in public buildings. The military and the airline industry have multiple needs for flame-retardant textiles. The requirements for a commercially successful flame-retardant textile product have been given1 as meeting flammability requirements: having little or no adverse effect on the textile’s physical properties; retaining the textile’s aesthetics and physiological properties; being produced by a simple process with conventional equipment and inexpensive chemicals; and being durable to repeated home launderings, tumble dryings and dry cleaning. It has been possible to meet these requirements for many textile products since before 19831 and our society enjoys a safer environment as a result. Progress is continuing in this field and recent reviews have highlighted advances in the understanding and chemistry of flame retardants,2,3 but progress has been relatively slow and the advances quite minor and specialised. Two excellent reviews have appeared1,4 and should be required reading for those wishing to have a comprehensive understanding of treatment with flame-retardant finishes. This chapter will cover the same ground in a much more general way. 8.2 Mechanisms of flame retardancy In order to understand the mechanisms of effective flame retardants better, the mechanism of combustion should first be clarified. Combustion is an exothermic process that requires three components, heat, oxygen and a suitable fuel. When left unchecked, combustion becomes self catalysing and will continue until the oxygen, the fuel supply or the excess heat is depleted. A diagram of the current model of combustion of textile fibres is given in Fig. 8.1.2 98 © 2004 by Woodhead Publishing Ltd Flame-retardant finishes 99 8.1 Combustion cycle for fibres. When heat is applied, the fibre’s temperature increases until the pyrolysis temperature, TP, is reached. At this temperature, the fibre undergoes irreversible chemical changes, producing non-flammable gases (carbon dioxide, water vapour and the higher oxides of nitrogen and sulfur), carbonaceous char, tars (liquid condensates) and flammable gases (carbon monoxide, hydrogen and many oxidis- able organic molecules). As the temperature continues to rise, the tars also pyrolyse, producing more non-flammable gases, char and flammable gases. Eventually, the combustion temperature, TC, is achieved. At this point, the flamma- ble gases combine with oxygen in the process called combustion, which is a series of gas phase free radical reactions (Fig. 8.2). These reactions are highly exothermic and produce large amounts of heat and light. The heat generated by the combustion process provides the additional thermal energy needed to continue the pyrolysis of the fibre, thereby supplying more flammable gases for combustion and perpetuat- ing the reaction. The burning behaviour of textiles is determined more by the speed or rate of heat release than by the amount of this heat. Attempts to disrupt this cycle for textile substrates have focused on several approaches. One method is to provide a heat sink on or in the fibre by use of materials that thermally decompose through strongly endothermic reactions. If enough heat can be absorbed by these reactions, the pyrolysis temperature of the 8.2 Some free radical combustion reactions. © 2004 by Woodhead Publishing Ltd 100 Chemical finishing of textiles 8.3 Endothermic decomposition reactions. fibre is not reached and no combustion takes place. Examples of this method are the use of aluminium hydroxide or ‘alumina trihydrate’ and calcium carbonate as fillers in polymers and coatings (Fig. 8.3). Another approach is to apply a material that forms an insulating layer around the fibre at temperatures below the fibre pyrolysis temperature. Boric acid and its hydrated salts function in this capacity (Fig. 8.4). When heated, these low melting compounds release water vapour and produce a foamed glassy surface on the fibre, insulating the fibre from the applied heat and oxygen. A third way to achieve flame retardancy is to influence the pyrolysis reaction to produce less flammable volatiles and more residual char. This ‘condensed phase’ mechanism can be seen in the action of phosphorous-containing flame retardants which, after having produced phosphoric acid through thermal decomposition, crosslink with hydroxyl-containing polymers thereby altering the pyrolysis to yield less flammable by-products (Fig. 8.5). But there are also other explanations for the first steps of this dehydration, including single esterification without crosslinking, for example, of the primary hydroxyl group in the C-6 position of the cellulose units. These phosphorous esters catalyse the dehydration (Fig. 8.6) and prevent the formation of undesired levoglucosan (Fig. 8.7), the precursor of flammable volatiles.5 8.4 Formation of foamed glass. 8.5 Crosslinking with phosphoric acid. © 2004 by Woodhead Publishing Ltd Flame-retardant finishes 101 8.6 Dehydration of cellulose by strong acids. 8.7 Thermal degradation of cellulose. Table 8.1 Comparison of two important flame-retardant mechanisms Type of mechanism Condensed phase Gas phase Type of chemistry involved Pyrolysis chemistry Flame chemistry Typical type of synergism P/N Sb/Br or Sb/Cl Effective for fibre type Mainly cellulose, also All kinds of fibres, wool, catalysing their because their flame dehydration to char chemistry is similar (radical transfer reactions) Particularities Very effective because Fixation with binder dehydration and carbon- changes textile isation decrease the properties such as formation of burnable handle and drape, volatiles preferably for back coating, for example of furnishing fabrics and carpets Application process If for durable flame Relatively simple, retardancy then demand- standard methods of ing multi-step processes coating, but viscosity control is important Environment, toxicity With durable flame retard- Antimony oxide and ancy, formaldehyde organic halogen emission during curing donators (DBDPO and and after finishing, HCBC) are discussed phosphorous compounds as problems (for in the waste water example possibility of generating polyhalo- genated dioxins and furanes) © 2004 by Woodhead Publishing Ltd 102 Chemical finishing of textiles 8.8 Competing free radical reactions during combustion of halogen (X)-containing material (M). R is the organic residue. The ‘condensed phase’ strategy includes the described mechanism of removal of heat and the enhancement of the decomposition temperature as in heat resistant fibres. In Table 8.1 the ‘condensed phase’ and the ‘gas phase’ mechanisms are compared. A fourth approach to preventing combustion is to interfere with the free radical reactions (flame chemistry, Fig. 8.2) that provide the heat needed for the process to continue. Materials that act in this ‘gas phase’ mechanism include halogen- containing compounds which, during combustion, yield hydrogen halides that form relatively long lived, less reactive free radicals, effectively reducing the heat available for perpetuating the combustion cycle, and which decrease the oxygen content by flame gas dilution (Fig. 8.8). 8.3 Flame-retardant chemistry The most important commercial flame retardants can be classified into three broad categories.1 Primary flame retardants based on phosphorous (condensed phase mechanism) and halogens (gas phase mechanism); synergistic retardancy enhancers that have only small flame retarding effects by themselves, but greatly enhance the flame retardancy of primary flame retardants (nitrogen with phospho- rous and antimony with halogens); and adjunctive flame retardants that exhibit their activity through physical effects (borates, alumina trihydrate, calcium carbonate and intumescents, explained in Section 8.10). Organic nitrogen is thought to help control the pH during the crosslinking reactions of phosphoric acid. The nitrogen can become protonated, reducing the amount of acid available. If the pH is too low, cellulose will undergo acid hydrolysis rather than crosslinking. If the pH is too high, the acid catalysed crosslinking cannot take place. Organic nitrogen may be converted to phosphorous acid amides that also catalyse the dehydration and carbonisation of cellulose. The synergistic effect of antimony comes from the volatility of antimony trihalides and © 2004 by Woodhead Publishing Ltd Flame-retardant finishes 103 8.9 Gas phase free radical reactions with antimony. Table 8.2 Synergistic combinations of flame retardants6 Synergistic combination Suitable for Generated primary active compounds P/N Cellulose H3PO4, P-amides Halogen (X)/Sb2O3 Synthetic fibres, especially SbOX → SbX3 PAN, PP, PA P/halogen (X) PP, (PET, PAN, PA) POX3, PX3 Halogen/radical generator Synthetic fibres, especially Halogenated polymers PET, CT, CA PP = polypropylene, PET = polyethylene tetrachloride, PAN = polyacrylonitrile, PA = polyamide, CT = cellulose triacetate, CA = cellulose acetate. the effectiveness of antimony compounds in scavenging free radicals (Fig. 8.9) over a broad temperature range (for example 245–565 °C).6 Table 8.2 shows common synergistic combinations of flame retardants. 8.4 Flame retardants for cellulose One important thermal degradation mechanism of cellulose fibres (cotton, rayon, linen, etc.) is the formation of the small depolymerisation product levoglucosan (Fig. 8.7). Levoglucosan and its volatile pyrolysis products are extremely flamma- ble materials and are the main contributors to cellulose combustion. Compounds that are able to hinder levoglucosan formation are expected to function as flame retardants for cellulose. The crosslinking and the single type of esterification of © 2004 by Woodhead Publishing Ltd 104 Chemical finishing of textiles 8.10 Thermal decomposition of ammonium salts. cellulose polymer chains by phosphoric acid reduces levoglucosan generation, catalyses dehydration and carbonisation, and thus functions as an effective flame- retardant mechanism. This carbonisation of cellulose is similar to the well known carbonisation process of wool with sulfuric acid, removing plant dirt and other cellulosics. In an idealised equation, flame-retardant finished cellulose (C6H10O5)n would be decomposed to 6n C and 5n H2O. The first step of this reaction is shown in Fig. 8.6. The resulting char is much less flammable than the volatile organic pyrolysis products of untreated cellulose. Chemicals that can yield phosphoric acid during the early stages of fibre pyrolysis form the majority of successful flame retardants for cellulose. However, it is not sufficient to supply just phosphoric acid precursors. The presence of nitrogen has been found to provide a synergistic effect with phosphorous. Mini- mum levels of added phosphorous and nitrogen for effective flame retardancy have been estimated at ~ 2 % P and ~1 % N. However, these minimum levels can vary greatly depending on fabric construction and test requirements. 8.4.1 Non-durable flame retardants for cellulose Inorganic salts have long been known to provide flame retardancy on cellulosic material that will not be exposed to water, rain or perspiration. The French chemist Gay-Lussac proposed a borax and ammonium sulfate treatment as a flame retard- ant for cotton in 1820. Today, a mixture of boric acid and borax is still an effective flame retardant for cotton at ~ 10 % solids add-on. Ammonium salts of strong acids, especially phosphoric acid (P/N synergism) are particularly useful as non- durable flame retardants for cellulose. Three commercially important products are diammonium phosphate, ammonium sulfamate and ammonium bromide. These salts readily form the corresponding strong acids upon heating (Fig. 8.10). © 2004 by Woodhead Publishing Ltd Flame-retardant finishes 105 Diammonium phosphate and ammonium sulfamate are used at ~ 15 % solids add- on and function as condensed phase flame retardants, not only by crosslinking but also by dehydrating cellulose to polymeric char with reduced formation of flammable by-products (Fig. 8.6). The water insoluble ammonium polyphosphate is an effective flame retardant and is added to coatings and binder systems, for example for pigment printing. Ammonium bromide is applied at ~ 10 % solids add-on and is effective in the gas phase. 8.4.2 Durable flame retardants for cellulose Although inorganic salts can provide excellent flame-retardant properties for cellulose, reasonable laundering durability must be incorporated into any finish destined for apparel use. The most successful durable flame retardants for cellu- lose are based on phosphorous- and nitrogen-containing chemical systems that can react with the fibre or form crosslinked structures on the fibre. The key ingredient of one of these finishes is tetrakis(hydroxymethyl)phosphonium chloride (THPC), made from phosphine, formaldehyde and hydrochloric acid (Fig. 8.11).1 THPC reacts with urea to form an insoluble structure on cellulose in a pad–dry–cure process (Fig. 8.12). 8.11 Synthesis of THPC. 8.12 Reaction of THPC with urea. © 2004 by Woodhead Publishing Ltd 106 Chemical finishing of textiles 8.13 THPC–urea–ammonia reaction. Some reaction with cellulose also occurs. Treating the cured finish with hydro- gen peroxide to convert the phosphorous atoms to their highest oxidation state results in cellulosic goods with very durable flame retardancy. Applying 25 % THPC with 15 % urea yields a final phosphorous add-on of 3.5–4 %, which is adequate for most fabrics. Although the THPC–urea system can give highly effective and durable flame retardancy to cellulose, treated fabrics are stiff and have significantly impaired tensile and tear strengths as well as releasing formal- dehyde during processing. Typically, carefully chosen softeners and mechanical finishing techniques are used to provide commercially acceptable fabrics. Varia- tions on THPC-based systems have been the use of the sulfate or hydroxy salts; THP-S to eliminate the possible formation of highly toxic bis(chloromethyl) ether during processing, and THP-OH to reduce acidic tendering of the goods. A variation on the THPC–urea system was developed to produce finishes with less stiffness and fibre damage (Proban process). A precondensate is prepared by the careful reaction of THPC with urea. This precondensate is padded onto the fabric and the fabric is dried to a specific moisture content (~ 15 %). The fabric is then exposed to ammonia vapours in a special reaction chamber, followed by oxidation with hydrogen peroxide (Fig. 8.13). The polymer that forms is primarily located in the lumen of the cotton fibre. The final finish provides durable flame retardancy to cotton with much improved fabric properties.1 It is important to note © 2004 by Woodhead Publishing Ltd Flame-retardant finishes 107 8.14 Reaction of N-methylol dimethylphosphonopropionamide with cellulose. that very few direct or fibre reactive dyes can withstand exposure to THP-based finishes. Almost all cellulosic goods that are to be flame retardant treated with a THP finish should be dyed with vat dyes. Another successful commercial approach to durable phosphorous-containing finishes is the use of N-methylol dimethylphosphonopropionamide (Fig. 8.14) in combination with trimethylol melamine and phosphoric acid as catalyst in a pad– dry–cure process.1 The required add-on is 20–30 % depending on the weight of the fabric. In this process, washing after curing is necessary to remove the phosphoric acid, leading to higher costs associated with the second drying step. In addition, the finish may give rise to an unpleasant odour during the curing step. Novel developments include higher product purity, decrease in formaldehyde emission during curing5 and by the finished textile, and also higher fixation rates enabled by moderate condensation conditions (accompanied by less fibre damage). Table 8.3 shows a comparison of these two important permanent flame-retardant finishes for cellulosics. Both processes are justified by the common finishing practice. Table 8.3 Comparison of two permanent flame-retardant finishes for cellulosics Modified THPC–urea finish N-methylol phosponopropionamide finish (‘Proban’ type) (‘Pyrocatex CP new’ type) Demanding process, including Extra washing after curing including moisture control, ammonia vapour drying costs treatment and oxidation Smaller wash shrinkage Softer handle Better stability to hydrolysis Fewer dyestuff restrictions, including brilliant shades Somewhat better ripping strength Much less free formaldehyde development Less odour bothering Shorter after-burning time Also for fibre blends with small Less smoke development cellulose content Preferably for large production No licence required, including runs, to minimise the process costs corresponding restrictions and costs including machinery requirements © 2004 by Woodhead Publishing Ltd 108 Chemical finishing of textiles 8.15 Viscose spinning bath additive for flame-retardant rayon. 8.4.3 Flame retardants for rayon Although in principle rayon can be flame retarded by the same processes devel- oped for cotton, the majority of research efforts have focused on additives to the viscose spinning bath. One successful additive, based on an alkyl dioxaphosphorinane disulfide (Fig. 8.15), is used at ~ 20 % for effective flame retardancy.1 8.5 Flame retardants for wool Despite the fact that wool fibres are inherently less flammable than most other fibres, some flame-retardant treatment is usually necessary in order to meet specific flammability tests. One well known process (Zirpro, developed by Benisek for the IWS) is based on hexafluoro zirconate and titanate salts.1,5 These products can be applied by exhaustion and pad processes under acid conditions (at pH < 3). The heavy metal complex anions form ionic and perhaps other polar bonds with the wool fibre, similar to dyestuff anions. The flame-retardancy mechanism is thought to take place in the condensed phase through zirconium ions or zirconium compounds that enhance or catalyse the char formation.5 Zirconium levels of ~ 2 % are needed for effective flame retardancy. The hexafluoro titanium salt is more effective and cheaper, but a yellow shade is imparted to the treated wool, which is increased by exposure to light. The finish is durable to dry cleaning and water washing up to 40 °C at pH < 6. At higher pH values ineffective zirconium oxide is formed.7 This finish can be combined with dyeing at pH < 3 (levelling acid or 1:1 metal complex dyes). It is compatible with shrink-resist and insect-resist finishes. Repellent finishes should be applied after the Zirpro process.8 This finish was modified for wool used for thermal insulation material, especially with skin contact,9 enabling less strength loss after heating and high flame retardancy (limiting oxygen index, LOI up to 35, see Section 8.11). Another flame-retardant treatment for wool based on exhaustion of an anionic species is the use of tetrabromophthalic anhydride, TBPA (Fig. 8.16), which hydrolyses to the carboxylic form during application. Use of TBPA at ~ 10 % on weight of fabric under acid conditions provides effective flame retardancy that is durable to dry cleaning and mild laundering conditions (cold water washing at neutral pH).1 But TBPA is suspected to generate polybrominated dioxins under burning conditions. © 2004 by Woodhead Publishing Ltd Flame-retardant finishes 109 8.16 Tetrabromophthalic anhydride (TBPA). 8.6 Flame retardants for polyester The three possible approaches to flame-retardant polyester – additives to the polymer melt, flame-retardant copolymers and topical finishes – have all been used commercially to produce flame-retardant polyester textiles.1 All the methods employ phosphorous- or bromine-containing compounds as the active flame retardant. One of the most useful flame-retardant finishes for polyester was a bromine- containing phosphate ester, trisdibromopropylphosphate, commonly known as ‘Tris’ (Fig. 8.17). Most simple phosphate triesters could also have been given the name of ‘tris’ for simplicity but the best known is the dibromopropyl product. ‘Tris’ was an extremely versatile and effective product, being applied by both padding and exhaustion processes (even though the substantivity and exhaustion yields were low) and provided excellent flame retardancy at reasonable add-ons. However, ‘tris’ was shown to be a potential carcinogen and was eventually removed from the marketplace by legislation.1 At the time, in the mind of the general public, anything that could be named ‘tris’ was considered to be a dangerous carcinogen! One current commercial flame retardant for polyester is a mixture of cyclic phosphate/phosphonates used in a pad–dry–heat set process (Fig. 8.18).10 Heat set conditions of 190–210ºC for 0.5–2 min are adequate. This product when applied at ~ 3–4 % add-on can provide durable flame retardancy to a wide variety of polyester textiles. 8.17 Tris(2,3-dibromopropyl) phosphate. 8.18 Cyclic phosphate/phosphonate flame retardant. © 2004 by Woodhead Publishing Ltd 110 Chemical finishing of textiles 8.19 Hexabromocyclododecane (HBCD). Another approach to durable flame retardant finishes for polyester is the use of highly brominated chemicals as topical finishes. One particularly useful material is hexabromocyclododecane (HBCD, Fig. 8.19). To achieve durable flame retardancy, fabric padded with ~ 8 % of a dispersion of this water insoluble material must be heated above 190 °C or 375 °F to form a film of the flame retardant on the fibre surface.11 Polyester fabrics when burned exhibit a melt–drip behaviour. Since the fabric melts away from the flame, some polyester fabric constructions can actually pass vertical flame tests without any flame-retardant treatment. The waiving of melt– drip specifications for children’s sleepwear has allowed untreated polyester garments to be sold into that market. 8.7 Flame retardants for nylon Although there are several possible methods of incorporating flame retardants into nylon, only additives to the polymer melt and topical finishes have been commer- cialised. Phosphorous- and bromine-containing compounds are the most common melt additives.12 The topical flame-retardant finishes for nylon that are of special interest are the treatments based on the condensation product of thiourea with formaldehyde and urea. The flame-retardancy effect of these products is attributed to the lowering of the melting point of nylon by 40 ºC and allowing the fibre to drip away from the ignition source.1 Common practice in nylon flame retardancy, especially for nylon carpets, is back-coating with antimony trioxide combined with bromine donators and a binder as shown in Fig. 8.20. 8.20 Flame-retardant system for fibre blends. © 2004 by Woodhead Publishing Ltd Flame-retardant finishes 111 8.8 Flame retardants for other fibres The most successful approach for flame-retarding acrylic fibres is to copolymerise halogen-containing monomers into the fibre. These modacrylic fibres have excellent permanent flame retardancy and acceptable fibre properties.1 Some problems including reproducibility of dyeing gave rise to their substitution by flame-retardant modified polyester, for example for curtain fabrics and other decorative textiles. Polypropylene fibres can be flame retarded with bromine- and phosphorous- containing additives to the polymer melt. However, very high add-ons are necessary and fibre properties are adversely affected. When polypropylene is used in carpets, flame retardancy can be achieved by incorporating halogen-containing compounds and antimony trioxide into the latex backing.1 Although m-aramid fibres (for example Nomex®) have an inherent flame retardancy, caused by their high decomposition temperature, this retardancy can be enhanced by the use of certain halogen/phosphorous compounds during ex- haust dyeing.13 Along with Nomex®, other inherently flame-resistant fibres such as Kevlar® (p-aramid), PBI (polybenzimidazole), Basofil® or Kynol® (melamine or phenol formaldehyde condensates), and flame-redardant modified polyester and regenerated cellulose find uses in protective clothing and other textiles that require flame resistance. Inorganic fibres such as ceramic and glass may be incorporated into, or used entirely for, textile products where appropriate to take advantage of their inherent non-flammability. Product examples are curtains, textile tapestries and fireblockers in airplane, automotive and military textiles. 8.9 Flame-retarding fibre blends Providing flame retardancy for fibre blends has proved to be a difficult task. Fibre blends, especially blends of natural fibres with synthetic fibres, usually exhibit a flammability that is worse than that of either component alone. Natural fibres develop a great deal of char during pyrolysis, whereas synthetic fibres often melt and drip when heated. This combination of thermal properties in a fabric made from a fibre blend results in a situation where the melted synthetic material is held in the contact with the heat source by the charred natural fibre. The natural fibre char acts as a candle wick for the molten synthetic material, allowing it to burn readily. This can be demonstrated by the LOI values of cotton (18–19), polyester (20–21) and a 50/50 blend of both (LOI 18), indicating a higher flammability of the blend as described later (Section 8.11). But a rare case of the opposite behaviour is also known (modacrylic fibres with LOI 33 and cotton in blends from 40–60 % can raise the LOI to 35). Even an antagonistic behaviour is reported for wool/polyester blends.8,14 Both Zirpro finished wool and Trevira CS, which is inherently flame retardant modified © 2004 by Woodhead Publishing Ltd 112 Chemical finishing of textiles by copolymerisation with methylpropionylphosphinic acid, are excellent flame protectors. But their blends burn easily. In order to flame retard natural/synthetic fibre blends, high levels of flame retardants are often required. One approach to this dilemma is to add the necessary amounts of retardant as a fabric coating. By using decabromodiphenyl oxide (DBDPO) in combination with antimony trioxide (Fig. 8.20), a cotton/polyester blend fabric has been produced that exhibits durable flame retardancy.1 Unfortu- nately, the finish required 37 % add-on of the retardants in addition to a latex binder and softener. The colour and hand of the finished fabric are significantly altered and chemical costs are high. However, if all of the coating is on the back of the fabric, the fabric face can be left essentially unaltered. The rubber-like hand of the fabric back can be minimised if the coating is applied discontinuously. Despite these drawbacks, advances in application technology and careful fabric design have led to the commercialisation of flame-retardant blends of cotton/polyester15 and cotton/nylon16 using precise combinations of phosphorous and bromine flame retardants and formulations that capitalise on a narrow window of effectiveness. 8.10 Novel approach to flame retardancy: intumescents Prevention from burning as early as possible is an accepted aim. Engineering the first steps of the burning process seems to be better than managing the last ones. In this context it might be preferable to moderate first the pyrolysis and then the flame chemistry. The concentration of research and development (R&D) work on the catalysis of the pyrolysis step gave rise to a new and promising approach, called intumescent.5 This is the generation of expanded, foamed char formed by heat and special additives, such as char formers (for example starch), catalysts that yield inorganic acids at about 150 °C, generators that provide non-flammable gases for the foam and binders for fixation to the fabric. This system provides a foamed insulation layer on the fabric surface, similar to the formation of char by cellulose, wool and especially by Basofil fibres. This porous char layer seems to fulfil many flame retardency requirements, such as preventing or retarding further ignition by thermal and chemical insulation, creating a flame barrier, including reduction of material exchange (volatiles, oxygen). Additionally smoke and toxic gas develop- ment is decreased. This double barrier function (for heat and material exchange) is very effective and avoids some ecological disadvantages of common flame retardants. A more detailed and competent overview is given by Horrocks.5 8.11 Evaluation of flame retardants Many factors influence the flammability of textiles, including the fibre type, the fabric weight and construction, the method of ignition, the extent of heat and material exchange, and the presence or absence of flame retardants. Differing © 2004 by Woodhead Publishing Ltd Flame-retardant finishes 113 Table 8.4 Common flammability tests Test method Sponsoring organisation Comments 16 CFR 1610 Consumer Product Safety Fabric at 45º angle to flame for Commission (CPSC) 1 s. For general apparel. 16 CFR 1615/1616 CPSC Fabric held vertical to flame for 3 s. For children’s sleepwear. NFPA 1971 National Firefighters Fabric held vertical to flame for Protection Association 12 s. For protective clothing. (NFPA) NFPA 701 NFPA Fabric held vertical to flame for 45 s to 2 min. For drapery. ASTM D-2863 ASTM Fabric is held vertical in Limiting oxygen atmosphere of different oxygen/ index (LOI) nitrogen ratios and ignited from top. Determines minimum oxygen level to support combustion. BS 5852 Part 1 British Standards Burning behaviour of and 2, for Institution upholstered furniture fabrics ignition sources (also for private use) against ‘cigarette’ and smoker-materials like cigarettes ‘match’ equivalent and matches. Finished fabric also EN 1021 and must be soaking resistant at EN 597 40 °C according to BS 5651, then horizontally and vertically fixed on a mini chair on a support of foamed PU, by seven ignition methods. ISO 6940/6941 International Standards Vertically held specimens, Organisation determination of the ease of ignition/the flame spread properties. DIN 54333 T1 Deutsches Institut für Horizontally held specimens, Normung because of the heat distribution less severe than vertical tests performance requirements and government regulations have led to the develop- ment of numerous test methods for evaluating the flame retardancy of textiles.17 According to the great variety of textile usage there are numerous test methods with vertical, horizontal or diagonal arrangement of the samples, methods with and without air ventilation, and many special tests, for example for carpets and fire protection clothing. Some of the more commonly encountered tests are given in Table 8.4. In the case of military fabrics, each fabric will have to fulfil the requirements and procedures listed in the MIL specification. © 2004 by Woodhead Publishing Ltd 114 Chemical finishing of textiles Table 8.5 Limiting oxygen index (LOI) values of different types of fibres Flammability in air LOI Fibre type Easy ignition, rapid burning 18.2 Acrylic 18.4 Cotton 18.6 Viscose 19 Polypropylene Normal ignition and 20–21 Polyester PET burning behaviour 20–21.5 Nylon 6 and 6.6 Almost ignition resistant 25 Wool Flame retardant with LOI > 26 29–30 Modacrylic 28–31 Meta-aramide 29–31 Para-aramide Flame retardant under severe 30–34 Phenol–formaldehyde type (Kynol) conditions, for example with 32 Melamine-formaldehyde type (Basofil) heavy air ventilation, LOI 32 Poly(aramide-imide) (Kermel) about > 30 34 Polyphenylenesulfide (PPS) 35 Polyetheretherketone (PEEK) 36–38 Polyimide (PI, P84) >41 Polybenzimidazole (PBI) 44–45 Polyetherimide (PEI) 45–55 Partially oxidised PAN (Preox, Panox) 60 Poly(vinylidene/vinylchloride) PVDC 68 Polybenzoxazole (PBO, Zylon) 98 Polytetrafluoroethylene (PTFE) Not burning, even in pure Glass and ceramic fibres, inorganic oxygen (LOI 100), only melting compounds on their highest oxidation level A measure that enables an obvious assessment of flame protection properties is the limiting oxygen index (LOI), determined according to ASTM D-2863. The LOI is defined as the content of oxygen in an oxygen/nitrogen mixture that keeps the sample at the limit of burning: LOI = 100 × O2 : (O2 + N2) [8.1] As the oxygen content of air is 20 % corresponding to LOI = 20 all textiles with lower LOI values will burn quite easily in air and those with LOI values much higher than 20 will not burn. Table 8.5 illustrates this readily imaginable measure for the flammability and burnability of different types of fibres. For all methods, strict adherence to the testing protocol is crucial to obtaining reliable and repeatable results. However, it is important to recognise that if a fabric passes a particular test, it just means that the fabric passed this particular test. There are no other performance guarantees. More detailed information on actual flame retardancy test methods and an outlook on their development have been published.4,5,18 © 2004 by Woodhead Publishing Ltd Flame-retardant finishes 115 8.12 Troubleshooting for flame-retardant finishes and particularities The legal implications of selling a flame-retardant fabric require a very thorough testing programme to guarantee that all areas of the fabric, side to side, end to end, and piece to piece meet the necessary performance requirements. Uniform appli- cation of flame retardants is the key to meeting these requirements. For pad applied finishes, all the factors that influence uniformity must be identified and controlled. These include pad roll pressures, finish bath concentrations and uniformity of drying and heat setting. Both exhaust and pad applied finishes are also susceptible to adverse flammability interactions from softeners, lubricants and other finish components. Laboratory evaluations of all new components must be completed before introducing any new finish into a production environment. There are also serious side effects to fabric physical properties from flame- retardant finishing that must be recognised, often caused by the high application levels of the flame retardants. Harsh hand, loss of tensile strength and colour effects (fabric yellowing and dye shade changes) are common problems with durable flame-retardant finishes for cotton. The combination with other finishes, such as softeners, easy-care and repellent finishes, must be carefully tested. The flame retardancy of the multi-purpose finish is more often reduced than it is acceptable. The toxicity of some flame-retardant components and of their combustion gases is a particular concern for flame-retardant finishes, especially if based on halogens and several heavy metals. Therefore, aircraft textile equipment has to fulfil special requirements, for example, smoke density and toxicity tests. Toxicity problems include: halogenated compounds, especially aromatics, are capable of generating polyhalogenated dioxins and furans, whether or not the hexa- or the penta-bromium compounds (HBCD or DBDPO) are more dangerous, dust that contains antimony oxide, phosphorous, antimony and zirconium compounds in the waste water, halogenated organic flame retardants, especially aromatic ones, get into the waste water (often they are only slowly biodegradable and cause high AOX (halogenated adsorbable organics) values) and formaldehyde release during curing of the permanent flame retardant finishes of cellulose and free formaldehyde of finished fabrics (storage, transport). Most flame-retardant finished textiles are excluded from the Öko-Tex Standard 100 label. In all cases, the durability of the finish is often a problem. It is the responsibility of the fabric finisher to address these issues if commercial flame- retardant fabrics are to be produced. An alternative for the fabric designer without most of the named problems is the use of flame-resistant modified fibres, but © 2004 by Woodhead Publishing Ltd 116 Chemical finishing of textiles unfortunately these give rise to other problems. Combination with other finishes mostly enhances the flammability if they include flammable organic compounds. With silicone-containing finishes the silicate residue, formed during burning, may prevent thermoplastic fibres from melting away from the flame, thus increasing the burning. Similar effects are reported for fluorocarbon finishes on polyester. References 1 Levin M, Handbook of Fiber Science and Technology, Vol. II, Chemical Processing of Fibers and Fabrics. Functional Finishes, Part B, Levin M and Sello S B (eds), New York, Marcel Dekker, 1984, 1–141. 2 Horrocks A R, ‘Flame retardant finishing of textiles’, Review Progress Coloration, 1986, 16, 62–101. 3 Holmes I, ‘Recent advances in chemical processing’, Colourage, 1998, 45(annual), 41– 56. 4 Horrocks A R, ‘Textiles’, in Fire Retardant Materials, Horrocks A R and Price D, (eds), Cambridge, Woodhead Publishing, 2001, Chap 4, 128–181. 5 Horrocks A R, in Textile Finishing, Heywood D (ed.), Bradford, Society of Dyers and Colourists, 2003, 214–250. 6 Einsele U, ‘Wirkungsweise und synergistische Effekte bei Flammschutzmitteln für Chemiefasern’, Melliand Textilberichte, 1976, 57, 64–72. 7 Benisek L, private communication. 8 Heiz H, ‘Flammhemmende Ausrüstung von Wolle’, Textilveredlung, 1981, 16(2), 53– 58. 9 Schindler W and Korndörfer C, ‘Modifizierung von Wolle zur Verbesserung ihrer Thermostabilität und Flammresistenz’, Chemiefasern/Textilindustrie, 1988, 38/89(7/8), T82–T83. 10 (a) Anderson J J, Camacho V G and Kinney R E, ‘Cyclic phosphonate esters and their preparation’, US Patent 3,789,091, 1974; (b) Anderson J J, Camacho V G and Kinney R E, ‘Fire retardant polymers containing thermally stable phosphonate esters’, US Patent 3,849,368, 1974; both patents assigned to Albright & Wilson Inc. 11 Johnson J R, Functional Finishes and High Performance Textiles, AATCC Symposium, Charlotte, NC, January 27–28, 2000. 12 Pearce E M, Shalaby S W and Barker R H, ‘Retardation of combustion of amides’, Flame Retardant Polymeric Materials, Vol. 1, Lewin M, Atlas S M and Pearce E M (eds), New York, Plenum Press, 1975, Chap 6, 239–290. 13 Riggins P H and Hauser P J, ‘Exhaust process for simultaneously dyeing and improving the flame resistance of aramid fibers’, US Patent 4,898,596, 1990, assigned to Burlington Industries. 14 Benisek L, ‘Antagonism and flame retardancy’, Textile Research Journal, 1981, 51, 369. 15 Johnson J R and Sujarit C, ‘Flame resistant polyester/cotton fabric and process for its production’, US Patent 4,748,705, 1988, assigned to Burlington Industries. 16 Hauser P J, Triplett B L and Sujarit C, ‘Flame-resistant cotton blend fabrics’, US Patent 4,732,789, 1988, assigned to Burlington Industries. 17 Standard D-4723, American Society of Testing Materials, 1999. 18 Wakelyn P J, Rearick W and Turner J, ‘Cotton and flammability – overview of new developments’, American Dyestuff Reporter, 1998, 87(2), 13–21. © 2004 by Woodhead Publishing Ltd