Foundation Engineering PDF
Document Details
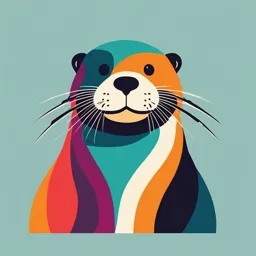
Uploaded by AmenableRhodolite
Tags
Summary
This document provides a detailed overview of foundation engineering, encompassing various aspects including design principles, types of foundations (shallow and deep), and considerations for different soil conditions. The key concepts discussed include bearing capacity and settlement analyses, with specific examples and illustrations. The information also touches upon site improvement methods.
Full Transcript
FOUNDATION ENGINEERING Foundation – is the part of an engineered system which transmits to, and into, the underlying soil or rock the loads supported by the foundation and its self-weight. All engineered construction resting on the earth must be carried by a foundation. Superstructure – is commonl...
FOUNDATION ENGINEERING Foundation – is the part of an engineered system which transmits to, and into, the underlying soil or rock the loads supported by the foundation and its self-weight. All engineered construction resting on the earth must be carried by a foundation. Superstructure – is commonly used to describe the engineered part of the system bringing load to the foundation, or substructure. The term superstructure has particular significance for buildings and bridges; however, foundations also may carry only machinery, support industrial equipment (pipes, towers, tanks) ast as sign in bases, and similar. It is better to describe foundation as that part of the engineered system interfacing the load carrying components to the ground. It is evident based on this definition of a foundation that it is the most important part of the engineering system. Foundation Engineer – is the person who by reason of training and experience is sufficiently versed in scientific principles and engineering judgment to design a foundation. The necessary scientific principles are acquired through formal educational courses in geotechnical (soil mechanics, geology, foundation engineering.) and structural (analysis, design in reinforced concrete and steel, etc.) STEPS IN DESIGNING A FOUNDATION: 1. Locate the site and the position of the load. 2. Physically inspect the site for any geological, or other, evidence of possible problems. Supplement this with any previously obtained soil data. 3. Establish the field exploration program and based on discovery set up the necessary supplemental field testing and the laboratory test program. 4. Determine the necessary soil design parameters based on integration of test data, scientific principles, and engineering judgment. Simple or complex computer analyses may be involved. For complex problems, compare the recommended data with published literature or engage another geotechnical consultant to give an outside perspective to the results. 5. Design the foundation using the soil parameters from step 4. The foundation should be economical and be able to to be built by the available construction personnel. Consider practical construction tolerances and local construction practices. Interact closely with all concerned (client, engineers, architect, contractor) so that the substructure is not excessively overdesigned, and risk is kept within acceptable levels. CLASSIFICATION OF FOUNDATION Shallow Foundation – termed bases, footings, spread footings, or mats. Where depth is generally D/B < 1 Spread Footing- supports a single column. Mat – is used to support several rows of parallel columns and may underlie a portion, or the entire building plan. Bases – foundation supporting machineries Deep Foundations – piles, drilled piers, or drilled caissons. D/B > 4 Pile – deep foundations are analogous to spread footings but distribute the load vertically rather than horizontally. A qualitative load distribution over depth for a pile. Drilled Pier and Drilled Caisson – are for the pile type member which is constructed by drilling a hole 0.76m in diameter, adding reinforcing, and backfilling the cavity with concrete. Retaining Structure – any structure used to retain soil or other material; in a geometric shape other than that naturally occurring under the influences of gravity. It may be constructed of many materials including wood and metal sheeting, plain or reinforced concrete, reinforce earth, precast concrete elements, closely spaced piling, interlocking wood or metal elements (crib walls), and similar. Sometimes it is permanent and in other cases it is removed when no longer needed. FOUNDATION ENGINEERING GENERAL REQUIREMENTS IN FOUNDATION DESIGN: 1. Determining the building purpose, probable service-life loading, type of framing, soil profile, construction methods and construction cost. 2. Determine the client/ owner’s needs. 3. Make the design but ensuring that it does not excessively degrade the environment and with a margin of safety which produces a tolerable risk level to all parties: the public; the owner; and the designer ADDITIONAL CONSIDERATIONS The previous section outlined in general terms requirements to be met in designing a foundation in terms of settlement and soil strength. We will now outline number of additional considerations which may have to be considered at specific sites. 1. Depth must be adequate to avoid lateral squeezing of material from beneath the foundation for footings and mats. Similarly, excavation for the foundation must take into account that this can happen to existing footings on adjacent sites and protection may be required for adjacent buildings. 2. Depth of foundation must be below the zone of seasonal volume changes caused by freezing, thawing, and plant growth. Most local building codes will contain minimum depth requirements. 3. The foundation scheme may have to consider expansive soil conditions. Here the building tends to capture soil moisture in the interior zone and allow normal evaporation around the perimeter. The soil in a distressingly large number of geographic areas tends to swell in the presence of substantial moisture and carry the foundation up with it. 4. In addition to compressive strength considerations, the foundation system must be safe against overturning, sliding, and any uplift (flotation}. 5. System must be safe against corrosion or deterioration due to harmful materials present in the soil. This is a particular concern in reclaiming sanitary landfills but has application where chemical agents present can corrode metal piling, cause adverse reactions with Portland cement in concrete footings or piles, etc. 6. Foundation system should be adequate to sustain some later changes in site or construction geometry and be easily modified should changes in the superstructure and loading become necessary. 7. The foundation should be buildable with available construction personnel. 8. The foundation and site development must meet local environmental standards. While not all the above are applicable to a given project, it is readily apparent that those that tend to introduce additional uncertainty into the system making the application of engineering judgement an even more important ingredient in the design process. SOIL EXPLORATION/ SAMPLING Investigation of the underground conditions at a site is prerequisite to the economical design of the substructure elements. It is also necessary to obtain sufficient information for feasibility and economic studies for a proposed project. Elimination of the site exploration, which usually ranges from 0.5 to 1.0 percent of total construction cost, only to find after construction has started that the foundation must be redesigned is certainly false economy. The elements of a site investigation depend heavily on the project but generally should provide: 1. Information to determine the type of foundation required (shallow or deep). 2. Information to allow the geotechnical consultant to make a recommendation on the allowable load capacity of the foundation. 3. Sufficient data/ laboratory tests to make settlement predictions. 4. Location of the groundwater table (or determination of whether it is in the construction zone). 5. Information so that the identification and solution of construction problems (sheeting and dewatering or rock excavation) can be made. 6. Identification of potential problems (settlements, existing damage, etc.) concerning adjacent property. 7. Identification of environmental problems and their solution. Methods of Exploration: - The most widely used method of subsurface investigation for compact sites and most extended sites is boring holes into the ground from which samples may be collected for either visual inspection or laboratory testing. FOUNDATION ENGINEERING Soil Boring o Geologic engineering tests to determine the capability of the soil to support proposed improvements or to determine the strength and properties of a required building foundation. o Exploratory holes into the soil may be made by hand tools, but more commonly mounted power tools are used. Hand Tools o The earliest method of obtaining a test hole was to excavate test pit using a pick and shovel. Because of economics, the current procedure is to use power-excavation equipment such as a backhoe to excavate the pit and then to use hand tools to remove a block sample or shape the site for in situ testing. This is the best method at present for obtaining quality undisturbed samples or samples for testing at other than vertical orientation. Mounted Power Drills o For numerous borings to greater depths and to collect samples that are undisturbed, the only practical method is to use power-driven equipment. 1. Wash boring – a term used to describe one of the more common methods of advancing a hole into the ground. A hole is started by driving a casing to a depth of 2 to 3.5m. Casing is simply a pipe which supports the hole, preventing it from caving in. 2. Rotary Drilling – is another method of advancing test holes. This method uses rotation of the drill bit, with the simultaneous application of pressure to advance the hole. Rotary drilling is the most rapid method of advancing holes in rock unless it is badly fissured; however, it can also be used for any other type of soil. 3. Continuous-flight augers – with a rotary drill are probably the most popular method of soil exploration at present in North America and Europe. The flights act as a screw conveyor Execution of soil borings General safety instructions Before performing any drilling, it is important to make sure that: - No utilities are present in the selected drilling points. Special attention must be paid to gas lines, electric wires and steam or hot water pipes. Beware that clashing of such lines may cause deaths. For this reason, a utility survey must be carried out at each drilling location using a pipe locator and if necessary, pre-drilling must be conducted. - Personnel is familiar with these tasks and the equipment used. - Personnel is using appropriate protective equipment. - Every person/organization that could be affected by the drilling activities knows about them. - In some cases, permits issued by appropriate authorities might be required in order to perform drilling activities Types of soil borings - When carrying out soil sampling very close to the surface, borings may be replaced by excavations with a trench digger. Only in rare cases digging by hand will be advantageous. Excavating by machine provides a good overview of the soil layers and how the contamination varies along the face of the excavation. Excavations are particularly advantageous in cases where the contamination is distributed unevenly, like for example in landfills. - borings are shallow investigation borings of up to 3-4 m in depth, which are made to determine contamination in the upper soil layers and in the aquifers close to the surface. - Investigation borings are borings of more than 4-5 m depth, where contamination in deeper soil layers and deeper groundwater is described. Drilling tools and operation - Soil borings can be completed by using drilling rigs, portable motor-driven augers, percussion gouge equipment or hand augers, and they should be correctly identified with a specific identification number. - Hand augers, portable motor-driven augers and percussion gouge equipment. In connection with shallow soil borings hand augers, portable motor-driven augers and percussion gouge equipments are usually used. Drilling can be performed up to a maximum of 3-4 m depth, but this will depend very much on the soil consistency. These equipments are recommended rather for loose soil and unpaved areas, because the rigs can’t drill through asphalt and concrete. - If asphalt or concrete is present, it has to be drilled previously by means of a drill. If geological conditions permit, the borings can be executed without the use of casing, since this method can provide adequate levelspecific soil samples in connection with shallow borings. Shallow localization soil borings can be filled back with excavated material if the borings do not exceed a depth of 3-4 m FOUNDATION ENGINEERING - Drilling rigs. Normally rotary and auger drilling rigs are used for drilling of environmental investigation borings: - Auger rigs (equipped usually with augers of 62 - 135 mm diameter). Drilling can be performed up to 5 - 30 m depth. - Rotary rigs. Drilling of boreholes by rotating a hollow core barrel (76 - 151 mm diameter) for soil and water sampling. Drilling depth is not limited. - One of the targets of investigation soil borings is to ensure water sampling at specific depths through a screen. For this reason, the dimensions of the borings must be selected according to the desired screen size and gravel pack. The following table shows typical associations between borehole and screen diameters. - Borings with this depth (> 4-5 m) should always be carried out using casing in order to ensure representative soil samples and in order to prevent cross contamination. Usually the method of boring used is dry rotation soil boring with casing, which will be removed during either well development or borehole abandonment activities. For drilling of deep soil borings, a double casing system will be used. One larger diameter will be used to drill down to the first layer. A smaller diameter casing will then be used inside the first casing to drill down to the second layer. This will prevent vertical cross contamination from the shallow soil to the aquifer. At conclusion of each soil boring, the hole will be filled with grout to ground surface to prevent cross- contamination between stratigraphic layers. Piezometers can be installed to investigate deeper aquifers, to monitor and remediate groundwater. - Furthermore, it is also important to decontaminate drilling equipment which meets soil before each drilling operation in order to avoid cross-contamination between different drilling locations and to ensure that collected samples are representative (see Chapter III “Management of Investigation Derived Waste and Equipment”). Finally, it is important to consider the following information, which should be collected during drilling operations: Name or identification number of soil boring Start and end date of works Observed lithology Soil appearance and color Presence of humidity Water levels and non-aqueous phase liquid levels, if present. Once drilling a soil boring is concluded, water level and possible presence and depth of non-aqueous phase liquids shall be measured by means of an interface probe. Drilling company Drill typology Boring depth Drilling device diameter Collected samples, with relative sampling depth and identification code Stratigraphy, with possible visual exam notes Photo of soil boring All field works must be performed in accordance with the corresponding procedures and security measures. In case no groundwater is present in the study area, the best scenario will be studied depending on each specific case: boreholes might be abandoned, or drilling operation might be continued to find groundwater at deeper depths, or to study contamination in the soil. Number and Depth of Borings There are no clear-cut criteria to give directly the number and depth of borings (or probings) required on a project in advance of some subsurface exploration. For buildings a minimum of three borings where the surface is level, and the first two borings indicate regular stratification may be adequate. Five borings are generally (at building cornes and center), and especially if the site is not level. On the other hand, a single boring may be sufficient for an antenna or industrial process tower base in a fixed location with the hole made at the point. In practice, the exploration contract is somewhat open as to the number of borings,. The drilling program advances so that sufficient borings are taken for that site to allow the geotechnical engineer to make a design recommendation with an adequate margin of safety that is economically feasible for the client. Borings should extend below the depth where the stress increase from the foundation load is significant. Since footing sizes are seldom known in advance of the borings a general rule-of-thumb is 2 x least lateral plan dimensions of the building or 10 m below lowest building elevation. Where 2 x width is not practical as, say, for a one-story warehouse or department store boring depths of 6 to 15m may be adequate. On the other hand, for important (or high rise) structures which may have small plan dimensions, it is common to extend some of the borings to bedrock or competent (hard) soil regardless of depth. It is axiomatic that borings for important structures do not terminate in a soft stratum. FOUNDATION ENGINEERING Summarizing, there is no binding rules on either number or depth of borings. Each site must be carefully considered with engineering judgment in combination with site discovery to finalize the program and with an adequate margin of safety Lesson 3: BEARING CAPACITY OF FOUNDATIONS The soil must be capable of carrying the loads from any engineered structure placed upon it without a shear failure and with the resulting settlements being tolerable for that structure. Seldom has a structure collapsed or tilted over from a base shear failure in recent times. Most reported base failures have occurred under embankments or similar structures where a low factor of safety was deemed acceptable. Most structural distress attributed to poor foundation design is from excessive settlements. Even here, however, structural collapse seldom occurs. This may in part be due to settlements being timedependent so that when cracks or other evidence first appears, there is sufficient time to take remedial measures. It is necessary to investigate both base shear resistance and settlements for any structure. In many cases settlements criteria will control the allowable bearing capacity; however, there are also several cases where base shear dictates the recommended bearing capacity. We should note that while primary focus is on bearing capacity for framed structures and equipment foundations, the same principles apply to obtain the bearing capacity for other structures such as tower, bases dams and fills. A recommendation for the allowable bearing capacity qa to be used for design is based either on settlement considerations or on the ultimate bearing capacity. The ultimate bearing capacity is divided by a suitable safety factor SF as: Bearing Capacity Equation by Terzaghi Example Prob 3-1: Compute the allowable bearing pressure using the Terzaghi Equation for the footing and soil parameters given below. Use safety factor of 3 to obtain qa. γ = 110 pcf ϕ = 250 c = 300psf D = 4 ft (depth of footing from ground surface) B x L = 2 ft X 2 ft (footing size) FOUNDATION ENGINEERING Effect of water table on Bearing Capacity The effective unit weight of the soil is used in the bearing capacity equations for computing the ultimate capacity. Example Prob 3-2: A square footing vertically and concentrically loaded is to be placed on a cohesionless soil as shown. The soil and other data are as shown. What is the allowable bearing capacity if γsat = 20.12 KN/m3 using the Terzaghi using SF = 2.0? Bearing Capacity for Rocks: Except for a few porous limestone and volcanic rocks and some shales, the strength of bedrock in situ will be greater than the compressive strength of the foundation concrete. This may not be true if the rock is in a badly fractured, loose state where considerable relative slip between rock fragments can occur. The major problem is to identify the rock soundness, and on occasion take cores for unconfined compression testing of the intact fragments. On very important projects and where it is economically feasible, one may make in situ strength tests. FOUNDATION ENGINEERING Example Prob. 3-3: We have a drilled pier with a diameter = 3 ft to be founded at a depth of 10 ft in a rock mass to get through the surface irregularities and the weathered zone as determined by coring to a depth of 25ft. From the cores the average RQD = 0.50 (or 50 percent) below the pier point. Assume: ϕ = 45o, and c = 0.5 ksi, SF = 3. Estimate the allowable bearing capacity for the pier base. Foundation Settlement Foundation settlements must be estimated with great care for buildings, bridges, towers, power plants, and similar high-cost structures. The settlement for structures such as fills, earth dams, levees, braced sheeting, and retaining walls can usually be estimated with a great margin of error. Soil settlement computations are, except for occasional happy coincidences, only best estimates of the deformation to expect when the future load is applied. During settlement we have the soil transitioning from the current body (or self-weight) stress state to a new one under the additional applied load. The stress change ∆q from this added load produces a time dependent accumulation of particle rolling, sliding, crushing, and elastic distortions in a limited influence zone beneath the loaded area. The statistical accumulation of movements in the direction of interest is the settlement. In the vertical direction the settlement will be defined as ∆H. The principal components of ∆H are the particle rolling and sliding which produces a change in the void ratio and crushing which alters the material. Only a very small fraction of ∆H is from elastic deformation of the soil grains. Therefore, if the applied stress were to be removed, very little of the settlement ∆H would be recovered. Even though ∆H has only a very small elastic component it is convenient to treat the soil as a pseudoplastic material with “elastic” parameters of Es, G’, μ and ks to estimate settlements. This would appear reasonable since a stress change causes the settlement and it is observed that larger changes produce larger settlements. Es = stress-strain modulus or modulus of deformation of soil G’ = shear stress-strain modulus of soil or other material μ = Poisson’s ratio - strain perpendicular to applied stress strain in direction of applied stress ks = modulus of subgrade reaction either vertical or horizontal There are two major problems with soil settlement analyses: 1. Obtaining reliable values of the “elastic” parameters. Problems of recovering “undisturbed” soil samples means laboratory values are often in error by 50 percent or more. There is now a greater tendency to using in situ tests, but a major drawback is they tend to obtain horizontal values. Since anisotropy is a common occurrence the vertical value (usually needed) is often substantially different. Because of these problems, correlations are commonly used particularly for preliminary design studies. 2. Obtaining a reliable stress profile from the applied load. We have both the problem of numerical values and the effective depth of the influence zone. Theory of elasticity equations are usually used for the stress computations with the influence depth H below the loaded area taken from about 2B to H - infinity. The values from these two problem areas are then used in some form of It is not uncommon for the ratio of measured versus computed ∆H to range 0.5- to 2+. Most values are in the 0.8 to 1.2 range, however. We might note, too, that a small computed ∆H of, say, 10mm where the measured value is 5 or FOUNDATION ENGINEERING 20mm has a large “error” but most practical structures can tolerate, either the predicted or measured value. What we do not want is to estimate 25mm and have the structure settle 100mm. If we err in settlement computations it is preferable to have computed values larger than the actual (or measured) ones – but we must be careful that the “large “value is not so conservative that expensive (but unneeded) remedial action is required. Settlements are usually classified as: 1. Immediate, or those which take place as the load is applied or within a time period of about 7 days. 2. Consolidation, or those which are time-dependent and taking months to years to develop. The “Leaning Tower of Pisa” in Italy has been settling unevenly (causing the “lean”) for over 700 years. This, however, is an extreme case with the principal settlements for most projects occurring in 1 to 5 years. Immediate settlement analyses are used for all fine-grained soils including silts and clays with a degree of saturation S < 90 percent (approximately) and for all coarse-grained soils with a large coefficient of permeability. Consolidation settlement analyses are used for all saturated, or nearly saturated, fine-grained soils where the consolidation theory applies. This is because for these soils we want estimates of both settlement ∆H and how long it will take for the settlement to occur. Both types of settlement analyses are in the form of Since the Ab term is a function of only the ratio of r/z, it may be tabulated in several values as follows: IMMEDIATE SETTLEMENT COMPUTATIONS The settlement of the corner of a rectangular base of dimension B’ x L’ on the surface of a elastic half-space can be computed from an equation from Theory of Elasticity as follows: where: qo = intensity of contact pressure in units of Es B’ = least lateral dimension of contributing base area in units of H Ii = influence factors which depend on L’/B’, thickness of stratum H, Poisson’s ratio , and embedment depth D. Es, = elastic soil parameters FOUNDATION ENGINEERING The H equation is strictly applicable to flexible bases on the half-space. In practice, most foundations are flexible – even very thick ones deflect when loaded by the superstructure loads. Some theory indicates that if the base is rigid the settlement will be uniform (but may tilt) and the settlement will be about seven percent less. On this basis if your base is “rigid” you should reduce the Is factor by about seven percent (that is, Isr = 0.931 Is). For best result, equation should be used as follows: 1. Make your best estimate of qo. 2. For round bases, convert to an equivalent square. 3. Determine the point where the settlement is to be computed and divide the base, so the point is at the corner or common corner of the contributing rectangles. 4. Note that the stratum depth actually causing settlement is not at H/B to infinity but is either: a. Depth z = 5B (B= least total lateral dimension of base), or b. Depth to where a hard stratum is encountered. Take “hard” as that where Es in the hard layer is about 10Es of adjacent layer. 5. Compute your H/B’ ratio. For a depth H = z = 5B and for the center of the base we have H/B’ = 5B/0.5B = 10. For a corner 5B/B =5 6. Enter Table below and obtain I1 and I2 and with your best estimate for m compute Is. 7. From the Figure estimate If. 8. Obtain the weighted average Es in the depth z = H. The weighted average can be computed as Improving Site Soil for Foundation Use 1.0 INTRODUCTION When the site soil is marginal (e.g., not enough bearing capacity, excessive settlement, or high permeability), soil improvement may be more economic than other means. Soil improvement refers to a technique to improve the engineering properties of soil. It involves changing soil characteristics by physical action such as compaction, vibration (to reduce void ratio) or by inclusion or mixing of stronger material (injecting admixture) in the soil. Aims of soil improvement: increase the load-bearing capacity and/or shear strength reduce both absolute and differential settlements or in certain cases, accelerate them mitigate or remove the risk of liquefaction in the event of an earthquake or major vibrations. The scope of application of the various techniques depends mainly on the type and grading of the soils that requires improving. FOUNDATION ENGINEERING 2.0 SOIL IMPROVEMENT TECHNIQUES A. Densification a. Consolidation (Preloading and Vertical Drains) b. Compaction c. Deep Dynamic Compaction d. Vibrocompaction (Vibroflotation) e. Vibro-Replacement Method f. Stone Columns g. Compaction Grouting h. Blasting B. Adhesion a. Chemical stabilization b. Slurry grouting, jet grouting c. Thermal stabilization C. Reinforcement a. Soil and rock anchors b. Mini piles c. Soil nailing d. Soil mixing D. Physico-chemical alteration a. Electro-osmosis b. Lime columns c. Vitrification d. Soil mixing E. Biological transformation 1. CONSOLIDATION: PRELOADING It is a relatively inexpensive, effective method to improve poor foundation soils in advance of construction of permanent facilities. As a rough estimation, it is about only 10-20% as expensive as other methods, and with vertical drains it is about 20-40%. It can be applied using earth fill, ponding (with geo-membrane if the topsoil is pervious), water tanks, or lower ground water table. This method is most effective on normally consolidated cohesive soil. If the deposits are thick and do not have alternative, sand seams, sand, wick, or PV drains may be necessary to reduce the consolidation time. Temporary surcharge is often used for the same purpose. 1) Preloading Methods a. Simply place a surcharge fill on top of the soil that requires consolidation. b. Once sufficient consolidation has taken place, the fill can be removed, and construction takes place. c. Surcharge fills are typically 10-25 feet thick and generally produces settlement of 1 to 3 feet. 2) Two major goals of Preloading: a. Eliminate settlements before the permanent structure is completed b. Densify the soil 3) Advantages of Preloading a. Requires only conventional earthmoving equipment b. Any grading contractor can perform the work c. long track record of success 4) Disadvantages of Preloading a. Surcharge fill must extend horizontally at least 10 m beyond the perimeter of the planned construction, which may not be possible at confined sites b. Transport of large quantities of soil required FOUNDATION ENGINEERING c. Surcharge must remain in place for months or years, thus delaying construction 2. CONSOLIDATION: VERTICAL DRAINS Vertical drains are installed under a surcharge load to accelerate the drainage of impervious soils and thus speed up consolidation. These drains provide a shorter path for the water to flow through to get away from the soil. Time to drain clay layers can be reduced from years to a couple of months. 1)Types of Vertical Drains A. Sand Drain In general, typical sand drains have a diameter from 20 to 45 cm and spacing from 1.5 to 6 m. Sand drains can be installation with several procedures: mandrel-driven pipes, driven pipes, rotary drill, or continuous flight hollow auger. The design is based on the time of preloading. Figure 2 shows a typical sand drain. B. Prefabricated Vertical Drain (PVD) / Wick Drain The technique consists in driving prefabricated drains vertically into the ground, following a regular grid. Most are about 100 mm wide and 5 mm thick. Geosynthetics are used as a substitute to sand columns Method of Installation of PVD 1. The wick drains are installed with mobile crane or mast-equipped rigs by using a needle- punching technique. 2. A long and thin mandrel is pushed into the ground. 3. An anchor keeps the drain in place and must attach to the wick drain before the installation. 4. The wick drain attached at the tip of mandrel is pulled into the ground as the mandrel advances. 5. At the desired depth, the fabric is cut. 6. The mandrel is withdrawn, and the fabric remains in place. 7. The rate of installation can be on the order of 1 m per second. 8. Installation depths up to 30 m have been used. (a) Pore water pressure flows laterally to the wick drain s and is carried vertically up to the ground surface. (b) Without inserting PVD, dissipation of excess pore pressure is a slow process. (c) With PVD, the excess pore pressure dissipates quickly through shorter drainage paths. Advantages of Using Wick Drains a. ease of installation (does not require drilling), b. Flexibility c. Reliability d. low cost (1/4 to 1/3 of the cost of sand drains) FOUNDATION ENGINEERING Wick drains have almost totally replaced conventional sand drains for most of the application. 5.0 COMPACTION Compaction is one of the most economical methods in soil improvement. It is the process by which the strength and stiffness of soil may be increased, and permeability may be decreased. Fill placements work often has specifications requiring a specific degree of compaction, or alternatively, specific properties of the compacted soil. In-situ, soils can be compacted by: a. excavation and recompaction b. vibrocompaction c. deep dynamic compaction d. compaction grouting 6.0 EXCAVATION AND RECOMPACTION Soil is excavated to a desired depth. Then it is filled with controlled lift thickness, water content, and compaction effort. The backfill material can be modified by mixed with an admixture (e.g. sand or gravel, cement, lime, or fly ash) or be come from nearby borrow pit. 7.0 DEEP DYNAMIC COMPACTION It is like Proctor Test Performed in the laboratory. A special crane lifts a heavy tamper (5-30 tons) to a height of 40 to 100 feet and drops the tamper several times onto the ground in a grid pattern. On clayey soils, stone is placed across the site under the mass, making the dynamic replacement effective. It is a very popular site improvement for land fill. 8.0 VIBRO-COMPACTION (VIBROFLOTATION) This is applied essentially to granular and non-coherent soils, such as sands and gravels. The vibrations induce a temporary liquefaction of the soil around the vibrator. In this case, the intergranular forces are temporarily nullified, and the grains are rearranged in a more compact pattern offering better characteristics. The induced vibration will reduce the void ratio and strengthen the soil. This improvement method has been found useful to treat liquefiable soils. This technique is often used on large works for the densification of hydraulic fills for reclamation. Site suitability The vibro-compaction system is one of the most economical and effective methods of densifying deep deposits of granular soils when the soils having a maximum of about 20% fines (preferable for 10% fines), of which not more than 3 % are active clay. The increase of fineness and plasticity of the soil decreases the feasibility of using the vibro-compaction method. Table 1 shows the summary of relative effectiveness of the methodology in various soil types. 9.0 VIBRO-REPLACEMENT METHOD Vibro Replacement is a combination of vibroflotation with a gravel backfill resulting in stone columns, which not only increases the amount of densificton, but provides a degree of reinforcement and a potentially effective means of drainage. Method of Installation 1) First, the vibroflot penetrates the ground to the desired depth. 2) Granular material (sands or gravels) is then progressively introduced to the hole, and the vibroflot is alternately raised and lowered to produce a packed sand (stone) column. 3) This method works well when the soil contains a large amount of fine contents. FOUNDATION ENGINEERING 4) The excellent candidates are fine sands and silty sands. 5) Only marginal success is reported for silts and clays. 6) This method can be used to form stone columns. 10.0 FOUNDATION GROUTING Grouting Methods a. Intrusion grouting - Consists of filling joints or fractures with grout - Primary benefit is reduction in hydraulic conductivity - Used to prepare foundation and abutments for dams - Usually done using cementitious grouts b. Permeation grouting - Injection of thin grouts into the soil - Once the soil cures, becomes a solid mass - Done using chemical grouts - Used for creating groundwater barriers or preparing ground before tunneling c. Compaction grouting - Used to repair structures that have excessive settlement and to compact loose soil or to produce control displacement to lift structure. - When low-slump compaction grout is injected into granular soils, grout bulbs are formed that displace and densify the surrounding loose soils. - Involves injection of low-slump (less than 2 inches) grout (soilcement mixture) which does not enter soil pores but remain in a homogeneous mass. - Grout material may consist of fine sand mixed with 12% cement and water to produce stiff, mortar-like mixture. - Grout pipe is installed to maximum treatment depth and grout is injected at high pump pressure as the pipe is withdrawn incrementally, forming a column of interconnected grout bulb - Can be performed “stage down” or, more commonly, in a “stage up” process, as follows: o Advancing the grout pipe to the bottom of treatment depth o Injecting the grout until refusal criteria is achieved, based on injected grout volume, injection pressure, or ground heave o Extracting the grout pipe to the next depth interval and injecting the grout o Repeat the process until reaching the upper limit of treatment zone d. Jet grouting - It is developed in Japan. - Uses a special pipe with horizontal jets that inject grout into the ground at high pressures - Jet grouting is an erosion/replacement system that creates an engineered, in situsoil/cement product known as Soilcretesm. - Effective across the widest range of soil types, and capable of being performed around subsurface obstructions and in confined spaces, jet grouting is a versatile and valuable tool for soft soil stabilization, underpinning, excavation support and groundwater control. 11.0 STABILIZATION USING ADMIXTURES The most common admixture is Portland Cement. When mixed with soil, forms soilcement which is comparable to a weak concrete. Other admixtures include lime and asphalt. The objective of this method is to provide artificial cementation, thus increasing strength and reducing both compressibility and hydraulic conductivity. This method is also used to reduce expansion potential of clays and in surface mixing applications. 1) Reinforcement - Soil is stronger in compression than in tension, so to improve strength in tension, geosynthetics placed in soil for soil reinforcement. 2) Soil Nailing - The fundamental concept of soil nailing consists of reinforcing the ground by passive inclusions, closely spaced, to create in-situ a coherent gravity structure and thereby to increase the overall shear strength of the in-situ soil and restrain its displacements FOUNDATION ENGINEERING 3) Inclusion - This technique is designed to provide structural support on all compressible soils. The process permits settlement reduction within acceptable limits. They should display deformation and stiffness characteristics appropriate for the surrounding ground and the structures to be supported. Inclusions are generally vertical and arranged in a regular grid. Different methods can be used (drilling with or without displacement, driving, vibrating) and different types of fills (ballast, gravel, soil-cement mixing and all types of mortar or concrete) can be used for constructing a superficial foundation system at minimal cost when compared to a deep foundations system. 4) Soil Mixing - The technique consists in the construction of columns of mixed in place soil with a cementitious material, normally cement. The in-situ remolding and mixing of the soil is achieved with rotary tools. The binder can be introduced as powder or slurry. The cement content can be varied to obtain a wide range of strengths and more flexibility in the mix properties, appropriate to the project requirements.