Forensic Examination of Fibres, Third Edition-23-78.pdf
Document Details
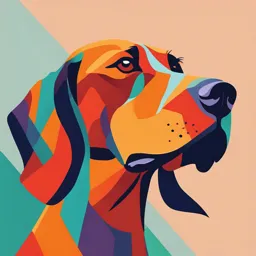
Uploaded by InvulnerableSun
Bundelkhand University
Tags
Full Transcript
2 Forensic Examination of Fibres 1.6.2.4 Polyvinyl Fibres 34 1.6.2.5 Polyurethane Fibres 35 1.6.2.6 Aram...
2 Forensic Examination of Fibres 1.6.2.4 Polyvinyl Fibres 34 1.6.2.5 Polyurethane Fibres 35 1.6.2.6 Aramides 36 1.6.2.7 Polyvinyl Alcohol Fibres 37 1.6.2.8 Inorganic Man-Made Fibres 37 1.7 Bicomponent Fibres 38 1.8 New Fibres 39 1.9 From Fibre to Fabric 39 1.9.1 Introduction 39 1.9.2 Yarns 40 1.9.2.1 Types of Yarns 41 1.9.3 Fabrics 41 1.9.3.1 Woven Fabrics 42 1.9.3.2 Knitted Fabrics 47 1.9.3.3 Pilling 52 1.9.4 Non-Woven Materials 53 1.9.4.1 Leather 55 1.10 Conclusions 57 Acknowledgments 58 Bibliography 58 Further Readings 58 Textbooks 58 1.1 Introduction If forensic fibre examiners are to fully exploit fibre and textile evidence during analysis they require not only specialised forensic knowledge but also knowledge of fibres, yarns and fabrics. Production, structure (both chemical and physical) and the properties of these materials is required in order to assess the value of fibre evidence. It is necessary to also know production figures, fashion changes, sudden arrivals of new material/s, dye variabil- ity and other factors that may have a bearing on the information obtained. What follows is a summary of fibres and the textiles that can be constructed from them. Information can also be obtained from catalogues, journals and texts, examples of which are listed at the end of this chapter. 1.2 Fibres The fundamental use of textiles by ancient man was for the purpose of protection and the initial textile used was the animal skin. As man became more sophisticated textiles became a way of showing status in wealth and society. Rapid population growth since World War II and consumerism has seen a rapid expansion of textile products from synthetic sources. Without synthetic textile products world production of natural fibres would be unable to satisfy world consumer demand for fibres and their products. The common misconception is that textiles relate in the main to apparel and home furnishings. There are however many other applications. These applications can be in the Fibres, Yarns and Fabrics 3 Table 1.1 Use of Textiles in Various Industries Industry Uses Medical Bandages, swabs, sutures, absorbent cloths Transport Inflatables, ropes, canvas, sails Mining Conveyor belts, flexible ducting, sacks Sports Windproof fabrics, playing surfaces, Equipment Furniture Upholstery, netting, bedding, wadding Horticulture Netting, shade cloths, hail protection, Twine Clothing Sewing threads, hats, garments, shoes Water Hoses, membranes, filters Chemical Filter fabrics, vat liners, overalls Architecture Awnings, carpets, wall coverings, drapes Aerospace Glider fabric, parachutes, composites Paper Making Filtration aids, conveyors, felts Automotive Deat belts, drive belts, interior trims, insulaion Civil Engineering Road construction, retaining walls, soil separation, slings Agriculture Animal coats & collars, sacks, flexible silos Defence Camouflage, clothing, ballistic vests industrial, medical, aerospace and automotive industries. Fibre types and processing routes vary depending on the industry of application. Table 1.1 lists examples of the wide variety of applications for textile products in the ever-changing world of industry. 1.2.1 Classification of Textile Fibres The basic component of a textile is a fibre. At the basic level all fibres fall into one of two categories: natural fibres and man-made fibres. Natural fibres fall into three chemical classes: Cellulose based fibres (from the seeds, stem and leaf of plants) Protein based fibres (from the hair, wool or silk of animals) Mineral based fibres (the only naturally occurring mineral fibre is asbestos the use of which is banned in many countries) Man-made fibres are manufactured and fall into three classes: Regenerated fibres (those formed from naturally occurring fibre-forming poly- mers, such as viscose from cellulose) Synthetic fibres (those formed from non-renewable sources, such as polyester) Inorganic fibres (those formed from inorganic materials, such as carbon and glass) Classification of fibres at this broad level is summarised in Figure 1.1. A more detailed breakdown within each class will be given as each is considered throughout this chapter. 4 Forensic Examination of Fibres Fibres Natural Man-made Animal Vegetable Organic Inorganic e.g. e.g. e.g. Wool Cotton Carbon Glass Transformation of natural Synthetic polymers e.g. e.g. Acetate Acrylic Lyocell Aramid Modal Modacrylic Figure 1.1 Broad classification of natural and man-made fibres. 1.2.2 Fibre Production Originally all textiles were composed of natural fibres, such as cotton, wool, silk, flax and jute. With the increasing demand for fibres that could perform a variety of functions, the invention and production of man-made fibres emerged. The first man-made fibre intro- duced in 1911 was rayon, which was a transformation of the naturally occurring poly- mer cellulose. The creation of synthetic polymers in 1930 made possible the production of organic synthetic polymer fibres. The first man-made synthetic polymer fibre was nylon in 1939. The development of new fibres continues even today (see Chapter 11) as advancing technical innovations expand fibre and fabric manufacturing processes. These innovations have resulted in more efficient manufacturing processes giving rise to a wider variety of products at lower cost to the consumer. As demand has shaped the quality of fibres manufactured so does demand influence the quantity of fibres produced each year. Global textile fibre production for the years 2000 and 2008 is illustrated in the Table 1.2. These figures show the very large increase in demand for polyester fibre, with relatively stable cotton production. Other natural fibres such as wool, silk and flax show little or no growth and static production. Polypropylene production and use, on the other hand, is growing, mainly due to its less complex manu- facture compared to nylon and polyester. 1.2.3 Fibre Characteristics Fibres used in textiles are fine and thin; the Textile Institute defines a fibre as: Textile raw material, generally characterised by flexibility, fineness and high ratio of length to thickness* Natural fibres, with the exception of silk, exist as staple fibres†with lengths ranging from 2 to 50 cm in length and cross sections ranging from 10 to 40 microns. The limited length of staple fibres means they need to be spun to be converted to a yarn. Synthetic fibres (and silks) are produced as continuous filament yarns. These yarns are sometimes cut to form staple yarns of specified lengths depending on the end use of the fibre. * Textile Terms and Definitions, 11th edition, Textile Institute. †A fibre of relatively short length. Fibres, Yarns and Fabrics 5 Table 1.2 Global Textile Fibre Production, for Major Fibre Types, for the Years 2000 and 2008 Global Textile Fibre Production (Million Tons) – 2000 2008 Cotton 19.7 24.4 Polyester 18.9 30.7 Polyamide 4.1 3.5 Polypropylene 6.0 5.9 Acrylics 2.7 1.9 Wool 1.3 1.2 Silk 0.1 0.2 Total 56.9 67.8 Source: Koslowski, H.-J. and Kassel, F. M., Fasen für Vliesstoffindustrie aktuelle Markttrends, 2008; American Fiber Manufacturers Association, Fibre Organon, 80, 95–112, 2009. The success of a fibre within a textile is significantly influenced by the polymer com- prising the fibre. 1.2.4 Properties of Fibre Forming Polymers Fibre forming polymers, either natural or synthetic, are a series of polymer chains held together by strong bonding with the lengths being several hundred times greater than the width. These polymers must also possess other general traits to make them useful as a fibre for textile purposes such as: 1. A degree of crystallinity of the molecular chains to give longitudinal strength along the fibre axis 2. Molecular chain flexibility to give extensibility and drape, particularly in apparel fabrics 3. High molecular weight giving both a high melting point and low solubility in most solvents Figure 1.2 shows the internal molecular structure of a man-made fibre illustrating the amorphous and crystalline regions. This polymer has all the features listed above, thereby making it suitable as a textile fibre. 1.3 Natural Fibres – Vegetable Fibres Vegetable fibres are divided into three groups based on the section of the plant from which they are produced (refer to Figure 1.3). 6 Forensic Examination of Fibres The chemical composition of vegetable fibres can vary dramatically, however the principle component in all the vegetable fibres is cellulose. Depending on the fibre, other constituents present consist of: hemi-cellulose; water soluble pectins; lignin as well as fats and waxes (refer to Table 1.3 for typical ratios of fibre constituents for some vegetable fibres). Crystalline region Amorphous region Figure 1.2 Morphology of a synthetic fibre. (Adapted from East, A.J., In J. E. McIntyre (Eds.), Synthetic Fibres: Nylon, Polyester, Acrylic, Polyolefin, Cambridge, Woodhead Publishing and CRC Press, 2005.) Fibres Natural Man-made Animal Vegetable Organic Inorganic Seed Stem (bast) Leaf Cotton Flax Kapok Jute Coir Ramie Hemo Figure 1.3 Sub-classification of vegetable fibres. Table 1.3 Approximate (%) Chemical Composition of Cellulosic Fibres Fibre Cellulose Hemicellulose Pectin Lignin Fat & Wax Cotton 92–95 5.7 1.2 0 0.6 Flax 62–71 16–18 1.8–2.0 2.0–2.5 1.5 Hemp 67–75 16–18 0.8 2.9–3.3 0.7 Ramie 68–76 13–14 1.9–2.1 0.6–0.7 0.3 Jute 59–71 12–13 0.2–4.4 11.8–12.9 0.5 Sisal 66–73 12–13 0.8 9.9 0.3 Abaca 63–68 19–20 0.5 5.1–5.5 0.2 Coir 36–43 0.2 3–4 41–45 Fibres, Yarns and Fabrics 7 OH OH O HO O HO O O OH OH n Figure 1.4 Repeating unit of glucose (units joined between the 1 and 4 carbon atoms). Cellulose is the carbohydrate part of the plant’s cell wall and is a polysaccharide macro molecule consisting of a large number of anhydroglucose units. The number of 1,4-β– glucose units in the cellulose chain can vary from several hundred to several thousand depending on the degree of hydrolysis that the cellulose undergoes. The repeating unit is illustrated in Figure 1.4. The molecular chains in natural cellulose vary in length. Variations in chain length results in differing solubilities in alkali and different viscosities but the same chemical properties. The degree of polymerisation (DP) is a measure of the chain length. Bast fibres have a DP of about 9000; wood cellulose on the other hand has a DP between 7,500 and 10,500. Hemi-cellulose is the name applied to a group of polysaccharides found in the cell walls of plants. The hemi-cellulose found in flax differs in constitution to that found in jute, which is again different to that found in hemp. Whilst hemi-cellulose is similar in structure to cellulose, the typical hemi-cellulose, xylose, does not contain the projecting CH2OH group found in cellulose. Because hemi-cellulose has a much shorter chain length (DP of about 120) it is readily soluble in dilute solutions of alkali. Lignin is an amorphous polymeric material concentrated in the spaces between the cells. Chemically, lignin differs from other natural polymers in being aromatic; destruc- tive distillation yields derivatives of phenyl propane. Lignified tissues are stronger and are more resistant to retting*. The lignin in plant cell walls can be almost completely removed by treatment with chlorine compounds such as sodium hypochlorite or sodium chlorite used in the bleaching process. The presence of large amounts of lignin in cellulosic fibres can result in substantial changes to colour on exposure to light. Jute, for example, a high lignin content fibre, will yellow when exposed to sunlight. Pectins are a complex group of substances of which the principle constituent is polygalaturonic acid in which a large proportion of the carboxyl groups have been meth- ylated. The pectins do not contribute to the overall strength of the fibre, as once removed by boiling in 1.0% solution of sodium hydroxide for 30 minutes in the absence of air, the strength of the fibre and its fluidity †remain unchanged. In mature cells, more rigid bonding between the cells is required and insoluble salts of calcium and magnesium pectinates occur. * Retting is the bacterial decomposition of lignan and hemicellulose. †Fluidity – A measure of the damage to cellulose. 8 Forensic Examination of Fibres 1.3.1 Seed Fibres – Cotton, Kapok and Coir Of all the natural fibres used in textiles, cotton is the most important with 24.4 million tonnes produced in 2008. It is also the most commonly encountered natural fibre in foren- sic examinations. Cotton is a natural vegetable fibre from the seedpod of plants in the genus Gossypium and is grown in subtropical climates. Two species are commonly cultivated; G. hirsutum, or upland cotton, accounts for 94% of world production and, G. barbadense, or Egyptian or Pima type cotton, which accounts for only 3%–4% of world production (Gordon, 2009). Cotton fibres exhibit considerable variability in their chemical and physical properties depending on the country of origin and the conditions under which they are cultivated. Variability can be evident in fibres even from the same seed-pod. Hence consistency in fibre sourcing is required to ensure minimal changes in processing characteristics between production lots. This presents a major challenge to the textile manufacturing industry. Figure 1.5 shows the major features in the cross-section of the cotton fibre. The cellulose content of cotton varies from a low of 88% to a high of about 96%. In the natural harvested state, the average cotton fibre is approximately 90% pure cellulose. This percentage rises to about 96% after industrial scouring and bleaching. Scouring removes naturally occurring waxes, salts and pectins present in the fibre. Bleaching gives the fibre a brighter, cleaner colour and serves to reduce imperfections in fibres from different sources. Depending on the method of yarn manufacture, cotton seed or husk may be present in the final yarn. Combed yarns* contain less cotton seed, as the majority of this is removed during the combing process. Carded yarns†however contain more of the seed, which is bleached during the bleaching process to give a more uniform fabric appearance. Unlike other cellulosic fibres, for example flax, hemp and jute, cotton is unique in that its strength does not decrease when wet. The strength of the cotton fibre increases when wet due to increased hydrogen bonding. This is because water molecules can easily form hydrogen bonds with the free cellulose hydroxyl groups. Water absorption at high humid- ity results in the swelling of the secondary cellular wall, which has little effect on the fibre length. This is unusual, as when swelling takes place there is normally a reduction in length proportional to the degree of swelling. During the scouring of cotton fabrics prior to dyeing, alkalis such as sodium hydrox- ide and sodium carbonate are used. This scouring removes waxes and impurities from the fabric and has an influence on the dye uptake depending on the amount of alkali used. Treatment of cotton with higher quantities of alkali, i.e. 26% sodium hydroxide Cellulose Lumen Wax Figure 1.5 Cross-section of a cotton fibre showing the major features. * Combing is a process that removes short fibres and some vegetable matter. †Carding is a process that straightens the fibres. Fibres, Yarns and Fabrics 9 (mercerising), has a more marked effect on the physical and chemical properties of the cotton fibre. The average regain of the fibre increases by almost 25%–10.5% at 65% relative humidity and 20°C. Mercerising (a permanent treatment) also results in physical changes to the cotton fibre that give added value to the final product. If the fabric is under tension during the sodium hydroxide treatment the fibre is prevented from shrinking during the swelling process. Surface lustre is developed, in part due to the changes that take place in the fibre cross-section. The fibre loses its kidney shape and becomes more circular as shown in Figure 1.6, thus increasing the surface reflective properties. Increased hydro- gen bonding between the molecular chains also occurs, thus giving an increase in fibre strength of approximately 20%. Since the fibre swells dramatically during the treatment, the fibrils in both the crystalline and non-crystalline regions become more accessible to the penetration of moisture. Thus the relative moisture absorbency increases. This increase in moisture absorbency increases the comfort factor of a typical cotton garment. At the (a) (b) Figure 1.6 Scanning electron micrographs of cotton fibre before (a and b) and after (c and d) treatment with 26% sodium hydroxide illustrating the change in cross-section and longitu- dinal aspect. Note – presence of dead cotton not affected by mercerisation process (circled in white). (Courtesy of Dr L. Arnold, RMIT University.) (Continued) 10 Forensic Examination of Fibres (c) (d) Figure 1.6 (Continued) Scanning electron micrographs of cotton fibre before (a and b) and after (c and d) treatment with 26% sodium hydroxide illustrating the change in cross-section and longitudinal aspect. Note – presence of dead cotton not affected by mercerisation process (circled in white). (Courtesy of Dr L. Arnold, RMIT University.) same time the dye-ability of the fibre increases, so that a lower quantity of dye is required for a given shade depth. Not all cotton fabrics are mercerised, however most cotton sewing threads are mercerised to give increased yarn strength. Cotton fibres have a natural twist or convolution which is present during fibre growth. The process of mercerising reduces and in some cases completely removes the convolutions. Figure 1.6a through d show the effect of mercerising on both the cross-section of the fibre and the longitudinal aspect of the fibre. Within any given cotton crop, a percentage of immature and dead fibres will always be present. The percentage of these fibres depends to a large extent on the conditions during the ripening period of the crop. Figure 1.7 illustrates the variability in the fibre cross sec- tion between mature, immature and dead fibres. Immature fibres dye lighter than mature fibres causing colour variation. Dead fibres in a yarn or fabric show as uncoloured or white flecks lowering the appearance of a fabric and thus the final finished quality. Cotton fibres sourced from different growing regions vary widely in fibre length, fine- ness and colour. These variations can result in large fibre losses during spinning and wet processing particularly when immature fibres are present. The presence of short fibres in a yarn can result in pilling, and immature fibres can cause colour variations (due to poor or no dye uptake). Variations between batches can also result in variable uptake of dye by the fibre, also giving rise to colour variations. Fibres, Yarns and Fabrics 11 Mature fibre with a very thick secondary wall. When dyed the secondary wall is dark. Notice the lumen is completely collapsed. Immature fibre secondary wall shows both thick and thin places, so the fibre will dye lighter. Dyeing properties can be improved by mercerising. Dead fibre with very little cell wall, when dyed the fibre appears white. Cannot be improved by mercerising. Figure 1.7 Mature (top), immature (middle) and dead (bottom) cotton fibres. This is particularly so when scouring using sodium hydroxide. Partial mercerisation of the fibre can occur. For this reason, the quantities of chemicals used in scouring and bleaching processes are carefully monitored. Cotton can be dyed with a wide variety of dyes; these include direct, reactive, vat, sul- phur and azoic dyes. Today the most common dye for cotton is the reactive dye. Military fabrics are usually dyed with vat dyes due to their very high performance to light and washing. Many T-shirt garments are printed or dyed using organic pigments, the colour being held on the garment by means of an acrylic resin binder. Kapok is from the seed pods of the kapok tree Celba pentandra, which grows in Malaysia and Indonesia. The fibre is light weight soft and hollow but brittle and is removed by hand from the seed pods, dried and shaken to remove any seeds. Fibre length is approximately 18–24 mm with a pronounced lumen. Compared to cotton kapok has a much lower cellulose content. The fibre is unsuitable for spinning into yarn due to its brittleness and inflexibility. It is an extremely buoyant fibre and will support more than thirty times its own weight and does not waterlog. Originally used in life vests and safety equipment, mattress and furniture fillings and pillows. Today its use has declined due to the introduction of synthetic fibres such as polyester, which is now widely used as fillings in many furniture applications. Coir is a coarse fibre that comes from the fibre mass between the husk of the coconut and the shell of the kernel. Coconuts are soaked in water to loosen the fibre and remove some of the hemicelluloses and pectins. The raw fibre consists of about 40% cellulose 40% lignin, the bal- ance being hemi-cellulose and pectins. The fibre can be recovered either by hand or machine that tears away the non-fibrous material from the longer fibres. It is dark brown in colour. Individual fibres are 15–30 cm in length. The fibres are combed and then spun into yarn, it can be bleached and dyed and has good resistance to abrasion and weathering. The principle application of coir is in ropes, twines and matting. Fishing nets can be made from coir fibre but to a large extent this application is diminishing and being replaced by fibres of synthetic origin. Coarser fibres are used in brushes, broom and door mats. 12 Forensic Examination of Fibres 1.3.2 Stem Fibres – Flax, Jute, Ramie, Hemp, Kenaf Stem or bast fibres form the fibrous bundles in the inner vascular areas of the stems of dicotyledenous plants. They are thick-walled cells that overlap one another and are cemented together by non-cellulose materials, principally pectin. The fibres are released from the woody bundles by a process of natural decomposition called retting, which could be considered to be controlled rotting. Unlike cotton, bast fibres have a lower cellulose content being only 65%–75% of the fibre. The other components are hemi- cellulose, pectin, and lignins. Of the bast fibres the major material is flax, the source of linen. Other bast fibres of interest are ramie, hemp, jute and kenaf. Blends of linen with synthetic fibres such as polyester are now common in high-class fashion shirtings and blouses. 1.3.2.1 Flax Flax comes from the stem of an annual plant Linum usitatissimum, which grows in both temperate and sub-tropical climates. The seeds of the flax plant are crushed, yielding flax oil known commercially as linseed oil. Within the inner bark there are long, thin, thick walled cells that compose the fibre strands. Only about one quarter of the stem is fibre. The fibres are held together by woody material and cellular tissue that must be removed to liberate the final fibre. Retting of the woody bundles is achieved through several different processes: Dam retting where the bundles of stems are immersed in ponds or dams for about 10 days. Dew retting where the stems are laid on the ground and left for several weeks, where they are continually wetted with dew and rain, which results in the forma- tion of moulds that enhance fermentation. Dew retted flax is darker than fibre that has been dam or tank retted. Tank retting where the stems are mechanically stripped of seed bolls and the straw tied in bundles and packed into a concrete tank filled with water. The water is heated to about 30°C and retting is completed in about 3 days. A second retting may be employed to improve the fibre quality. Chemical retting using alkalis such as sodium hydroxide and sodium carbonate with soap have also been used, but this method is more costly compared to the biological methods previously discussed. The lightest colour and best quality flax is produced by tank retting. The dried retted flax is then ready for breaking and scutching. In this process, the dried retted flax is passed between fluted rollers that break the woody core without damaging the fibres. In scotching, the broken straw is beaten on a scutching machine to separate the individual fibres from the unwanted woody matter. After scotching, the fibres are combed or ‘hackled’ by drawing the fibre through sets of pins, each successive set finer than the previous set. This process separates the coarser fibres and also arranges them parallel ready for spinning into yarn. After boiling and bleaching, flax is almost pure cellulose with a specific gravity of 3.54. Flax is soft with a lustrous appearance, yellowish white in colour, but can vary depending on the retting process used. Fibre length is 6–65 mm, but on average is about 20 mm, and Fibres, Yarns and Fabrics 13 has a fineness of approximately 20 microns. In cross-section the fibre is polyganol with thick cell walls. Immature fibres are more oval in cross-section. A characteristic lumen or canal runs through the centre of the fibre. The fibre is stronger than cotton and has minimal extensibility. It has good resistance to alkalis and will withstand the action of dilute acids. Because of its high moisture absorption properties it is used in table linen and towelling. Other applications of flax are in high quality ladies’ blouses and shirts. The application of blends of linen with synthetic fibres such as polyester greatly improves its easy-care properties. 1.3.2.2 Jute The jute plant Corchorus is an annual that grows in hot damp climatic regions and is culti- vated mainly in India and Bangladesh. Only two species are grown on a commercial scale, G, capoularis and G. olitorius; the first species produces ‘white jute’ and the second ‘Tossa jute’. White jute is a little misleading as the natural fibre is in fact a golden yellow colour. Retting is carried out in a similar manner to that employed for flax. Commercial jute var- ies in colour from yellow to brown. Chemically the dry fibre consists of 71% cellulose, 13% lignin 13% hemicelluloses, 0.2% pectin 0.5% fats and waxes and 2.3% water solubles. The fibre strands can be up to 2 meters in length. Following retting, the fibres are hung to dry in the sun, then compressed into bales ready for spinning. In cross section, the jute fibre is polygonal with five to six sides. The lumen is oval in cross-section but irregular compared to flax, which has a regular lumen. Compared to flax, jute is not as strong due to the uneven thickness in the cell walls. The moisture absorption properties of jute are unusual, being very hygroscopic, able to absorb up to 23% moisture in humid conditions with a normal regain of 13.75%. Prolonged exposure to moisture causes the fibre to lose strength. Jute is more resistant to micro-organisms than either grey cotton or flax; this is due to the pro- tective effect of the lignin. To a large extent, many applications for jute have been replaced by synthetic fibres such as polypropylene and polyester, however jute is still used as the secondary backing in carpets due to its non slip properties. Other applications for jute are ropes, cordage, linings in older style furniture, sackings and bales. Kenaf, Guinea hemp or Mesta from the plant Hibiscus cannabinus grown in Africa, India and Bangladesh, has similar properties to jute and can be used as a substitute but is produced on a much smaller scale on the international market. 1.3.2.3 Ramie Ramie or China grass comes from the plant Boehmeria nivea that belongs to the family of stinging nettles. It is a perennial grown mainly in China. The root sends up many stalks to a height of approximately 1.2–2.0 meters. These are harvested every two to three months when the lower stalks turn yellow and new stalks begin to appear. The fibres are removed from the stalks by decortication which consists of mechanically peeling or beating the bast and bark followed by soaking in water and scraping to free the fibres from the bark. The final fibres vary in length from 2.5–30 cm. The cellulose content of ramie rises from 75% cellulose to 85% cellulose after decortications, and after degumming the cellulose content on dry basis is 96%–98%. Degumming is accomplished by treatment in sodium hydroxide solution followed by bleaching. Ramie fibres are white and lustrous and are unaffected by the action of sunlight and can be blended with other fibres. The fibre has a high resis- tance to bacteria, fungi and mildew. Treatment in strong 26% sodium hydroxide solution (mercerising strength) brings about changes to the fibre similar to that achieved in the 14 Forensic Examination of Fibres mercerising process used on cotton. Fabrics made from ramie are very absorbent and dry quickly and can be used for the manufacture of towels and table linen. 1.3.2.4 Hemp True hemp derived from the plant Cannabis sativa has been in use since ancient times. The term hemp has been used to describe a range of fibres from different species used for the manufacture of ropes and cordages, some being derived from stalks and others from leaves such as Manila hemp and New Zealand hemp. Cannabis sativa is an annual plant grown from seed in temperate climates. The stalks should not exceed 1.8 meters in height if good quality fibre is to be produced. Harvesting takes place when the lower leaves turn yellow and the stalks are pulled from the ground. Retting and scutching are similar to that used for flax. Under microscopic examination hemp resembles flax in appearance; this similar- ity means that some hemp varieties are difficult to distinguish from flax. Hemp is overall a courser fibre than flax, being darker in colour and difficult to bleach. The cross-section of hemp is polygonal and the lumen is broader than that of flax. Dry hemp fibres have high cellulose content, approximately 75%; lignins are lower at 3.6%, compared to jute. Other components are hemi-cellulose about 17%, fats and waxes 0.8%, water solubles 2.0%, pectins 1.0% and lignins 3.6%. The major applications of hemp are in the manufacture of ropes, twines and cordages. More recently however there have been garments manufactured from blends of hemp with cotton. High quality hemp can be used as a substitute for flax. 1.3.3 Leaf fibres – Sisal, Hemp (Manila and New Zealand) The leaves of certain tropical and sub-tropical monocotyledonous plants are held in shape by fibrous cells that run in strands (vascular tissue) through the length of the leaf. These cells help to give the fibre strength and maintain its shape. As a general rule the leaf fibres are courser than fibres that come from dicotyledonous plants, which are the source of the bast fibres. The leaf fibres are often referred to as ‘hard’ fibres being that they are thicker and stiffer than either the bast or seed fibres. The major leaf fibres are sisal, henequin, New Zealand hemp and abaca. Synthetic and regenerated fibres from natural polymers have, to a large extent, taken a substantial share of the market for both the bast and leaf fibres. More recently however, with the rising cost of petroleum, there has been an increased awareness of the advantages of these natural products, particularly in relation to their sustainable production. 1.3.3.1 Hemp-Abaca (or Manila Hemp) and New Zealand Hemp Musa textilis a member of the banana family indigenous to the Philippines and is the source of manila hemp for the manufacture of high quality ropes and cordages. The fibre is in the form of strands of many fibres held together by natural gums. The length of these strands varies from 1–3 meters and 0.05–0.3 mm in diameter. Final fibre length is 3–12 mm and 16–32 μm in diameter. The fibre has a good natural lustre and is off-white in colour. Chemically abaca fibres contain about 77% cellulose on a dry weight basis and are coated with about 9% lignin. The individual fibres are separated from the soft fleshy material by drawing the strips of the leaf between a knife edge and a wooden block. The repeated scraping in this manner removes the soft cellular matter that surrounds the fibre. Once the fibre is cleaned it is hung up to dry. When dry it is ready for use. The fibre has a Fibres, Yarns and Fabrics 15 variable cross-section that is irregularly circular or oval in shape. One of the advantages of abaca is that it is not affected by salt water; hence it is ideally suited for use in marine cordage. New Zealand hemp is the perennial plant Phormium tenax native to New Zealand. Captain Cook noted that the Maoris produced fine fabrics from the leaves of this plant. Fibres are extracted from the leaves by mechanical scraping. Whilst the fibre is fairly strong and has good resistance to sea water it is weaker and softer than Manila hemp. The individual fibres are smooth with pointed ends with an average length of about 6–7 mm, a near circular cross-section and a narrow circular lumen. Compared to other leaf fibres, the lignin and hemicelluloses is higher and the cellulose content lower. 1.3.3.2 Sisal and Henequen Sisal is the leaf fibre that comes from the plant Agave sisalana. The name is derived from the Yucatan port ‘Sisal’ situated on the Gulf of Mexico. It was from this port that the fibre was first exported. The sisal plant is grown in tropical regions and grows huge leaves almost from ground level. The leaves are harvested when the plants are 2–4 years old. A good plant may yield up to 400 leaves in its lifetime of seven to eight years. The fibres are extracted from the leaves using a decortication process in a machine that scrapes the pulpy matter from the fibres. The fibres are washed, then dried either in the sun where bleaching takes place or in an oven. Fibre strands vary in length from 60–120 cm in length and have an average diameter of 24 μm. The fibre is polygonal in cross-section with a broad lumen which varies in thickness and is often packed with small granules. The fibre ends are blunt and in some cases forked. Sisal is used for the manufacture of ropes, cordages, twines, upholstery, carpet and custom rugs. Yarns have a tendency to shed the fibre and fabrics quickly fade out. Henequen fibre is produced from the plant Agava bourcroydes and is often known as ‘Yucatan sisal’ or ‘Cuban sisal’. The plant has prickly leaves that are grey green in colour. Processing of the leaves is similar to that for conventional sisal. The fibre strands are long and slightly finer than those of sisal. Applications include string and agricultural twine. 1.4 Natural Fibres – Animal Fibres Natural fibres that have an animal source (refer to Figure 1.8 for sub-classification), such as wool or silk, are protein based. Proteins are chain like molecules formed by the union of α-amino acids joined together by the peptide link as shown below. R R NH2 – CH NH2 – C – COOH COOH H The difference between proteins is related to differences in the side group –R pendent to the main chain. Over 20 amino acids are known to exist because of the difference in size, shape and chemical reactivity. The limited number of side groups is sufficient to result in an extremely large number of polypeptides and proteins due to arrangements that are pos- sible. Table 1.4 lists the differing ratios of amino acids in wool and silk fibres. 16 Forensic Examination of Fibres Fibres Natural Man-made Animal Vegetable Organic Inorganic Hair Silk Wool – sheep Bombyx mori – silkworms Goat family – mohair, cashmere Arachnids – spider silk Camel family – camel hair, alpaca, vicuna Hymenotpera – bees, wasps, ants Other – rabbit fur, possum fur Figure 1.8 Sub-classification of animal fibres. 1.4.1 Wool Of the protein fibres, wool is the predominant fibre, with world production being approxi- mately 1.2 million tonnes in 2008. Raw wool after shearing contains impurities, such as grease, swint (sweat), dirt and vegetable matter. The quantities of these impurities will vary depending on the agricultural region where the animal has been grazing. Merino sheep that are raised under shedded conditions show minimal amounts of vegetable matter and dirt present in the fleece. After scouring and removal of vegetable matter under conven- tional grazing conditions the yield will be about 65% of clean wool. It is normal for scoured wool to retain about 0.5% residual grease as this assists in later mechanical processes such as carding and combing to minimise fibre breakage. In a typical wool fibre there are four regions: 1. The epicutical (or outer sheath): A thin hydrophobic membrane and the only non- protein part of the wool fibre. This membrane is water repellent for water droplets but will absorb water vapour through its many microscopic pores (thus absorbing water vapour from the body and releasing into the air); 2. The scale-cell layer (comprising the enzyme resistant exocuticle and enzyme digestible endocuticle): This layer contains the flat scale-like cells which overlap each other (refer to Figure 1.9 for a cross section of wool illustrating the overlapping scales). The number of scales present on a wool fibre is known to vary greatly depending on the quality of the wool with fine wools having significantly more scales than coarse or medium wools. 3. The cortex: This layer comprises some 90% of the wool fibre consisting of millions of spindle shaped cells; and 4. The medulla: Many wool fibres (particularly the coarser ones) have a hollow cen- tral space running longitudinally to the fibre length. The medulla may be either empty or contain a loose network of open cell walls. Coarse and medium wools are generally characterised as having a greater proportion of the fibres medullated. Conversely, in fine wools the medulla is either absent or so fine as to not be visible. The dimension of wool fibres varies considerably depending on the quality of the wool. Fine wool fibres are generally about 3.8–12.7 cm long, medium wools are generally 6.3– 15.2 cm long and long wools are 12.7–38 cm long. The diameter can also vary significantly Fibres, Yarns and Fabrics 17 Table 1.4 Amino Acids Present in Silk and Wool Protein Fibres g- Amino Acid per 100 g Protein Amino Acid Silk Fibroin Wool Keratin Inert acids Glycine 43.8 6.5 Alanine 26.4 4.1 Valine 3.2 5.5 Phenylalanine 1.5 1.6 Isoleucine 1.4 0.0 Leucine 0.8 9.7 Acidic Glutamic Acid 2.0 16.0 Aspartic Acid 3.0 7.3 Basic Histidine 0.5 0.7 Lysine 0.9 2.5 Arginine 1.1 8.6 Hydroxyl Serine 12.6 9.5 Tyronsine 10.6 6.1 Threonine 1.5 6.6 Miscellaneous Proline 1.5 7.2 Cystine 0.0 11.8 Tryptophan 0.0 0.7 Cysteine 0.0 0.1 Methionine 0.0 0.4 with the average diameter for a top-quality merino wool being 17 μm; a medium wool fibre about 24–34 μm and a long wool fibre about 40 μm. Wool fibres have a natural wave or crimp which is a unique characteristic amongst natural fibres. The crimp is not in a single plane but has a three dimensional waviness. This natural waviness allows fibres to hold together when made into a yarn. Once again the quality of the wool will be reflected in the waviness of the fibre. The finest wools can have as many as 12 waves to the centimetre whereas poorer quality wools will have 2 (or even less). Intercellular cement holds the cuticle cells together and separates them from the underlying cortical cells. During the processing of washable wool, i.e. shrink resistant wool, the hydrophobic epicuticle is damaged and the scale structure is significantly changed due to the action of chlorine and a cationic resin. This process is the ‘KROY TM’ process. 1.4.1.1 Hair fibres or Speciality Wools Hair fibres or ‘Exotic fibres’ are those that come from other animals such as: 1. Goat family—mohair, cashmere 2. Camel family—camel hair, alpaca, vicuna 3. Rabbit fur and other fur animals such as the possum 18 Forensic Examination of Fibres Figure 1.9 Cross section of 28 micron wool illustrating the overlapping scales. Mohair is from the Angora goat which originated in Turkey, is a lustrous fibre with a staple length of 20–30 cm for a full year’s growth. The fibre’s surface has some similarities to wool; it is covered with epidermal scale anchored much more closely to the body of the fibre compared to wool, there being only about half the number of scales as there are on the wool fibre. The fibre has a circular cross section with an average diameter of about 25 μm for kid mohair and 35 μm for the adult fibre. Chemically, the main constituent is keratin, and its chemical behaviour is very similar to wool. Cashmere was the name originally given to the hair from the Asiatic goat Capra hircus laniger, the Tibetan Cashmere goat, which is raised as a domestic animal in parts of China, Persia and northern India. In Australia, New Zealand and Scotland hair from the selec- tively bred feral goats is also called cashmere. The cashmere fibre varies in length from 5–10 cm with diameter of 14–16 μm. In cross-section the fibre is circular or slightly oval, and in the coloured fibre the natural pigment can be clearly seen in the cortical layer. Because of its softness, cashmere is mainly used in high-quality ladies’ dress wear and knitwear. The fibre is more easily damaged chemically particularly by alkalis due to its fineness and better wetting properties. Camel hair is from the camel Camelus bactrianus. The hair is shed by the animal and collected. The soft inner hair is the finest and is used in high grade men’s over-coatings. Camel hair gives warmth without weight so the fibres are highly prized for quality apparel. The coarser outer hair or guard hair length is approximately 26–30 cm and is used in the manufacture of beltings and interlinings. Alpaca is from the fleece of the Lama pacos from South America. The fleece is coloured brown, fawn, black and sometimes white, with a fibre diameter of 24–26 μm and fibre length of 20–28 cm. Alpaca has a distinctive scale structure with a natural crimp and medullation. Dress fabrics, tropical suiting’s and blends with wool are common. Alpaca is difficult to dye and is usually used in its natural colours. The fibre is noted for its softness, fineness and lustre. Fibres, Yarns and Fabrics 19 Vicuna is the smallest and rarest of the llama family. The undercoat hair fibre from the vicuna is regarded as being the finest wool like fibre with a diameter of 13–14 μm. About 500 g of fibre is obtained from each animal. It is the rarest and most expensive of all fibres and has similar uses to cashmere. Angora fibre is obtained from the pelts of the angora rabbit. After shearing the hair from the pelts is separated by blowing. The fine hair, 6–8 cm in length 13 μm in diameter, is used in the manufacture of felt hats. Angora is difficult to dye and is very slippery and is usually blended with wool and or nylon to facilitate spinning. Other animal fibres may be encountered in textiles including fibres from domestic animals such as cats, dogs, cows, horses, rodents, etc., as well as human body hair. 1.4.2 Silk—Silkworm The silkworm is the caterpillar of a small white moth of the species Bombyx mori from the class of insects Lepitoptera. This caterpillar thrives only on Mulberry leaves and dur- ing the growth stage the worms do nothing but eat except for four periods of sleep, which last about 24 hours. During this resting period the worms shed their skin or moult. After the fourth moult it settles down to a feed lasting about 10 days during which period it eats about twenty times its own weight of leaves. Approximately thirty-five days after hatching the worm begins to spin its cocoon. The liquid silk is from two glands inside the worm. The silk flows in two channels to a common exit point which is the spinneret, in the worm’s head. As it exits the liquid hardens into very fine filaments that are coated and stuck together with a gummy substance called sericin which comes from two other glands nearby. Silk as it emerges from the worm is therefore a twin filament held together as a single strand by the sericin cement. Sericin is an amorphous globular protein and dissolves in hot soap solution. Raw silk contains approximately 75% fibroin and 25% sericin. Sericin remains on the filament surface to protect the fibre from damage during processing. De-gumming during wet processing of both silk yarns and fabrics removes the sericin, leaving a fibre of almost pure fibroin. Silk will dissolve in powerful hydrogen bond-breaking solvents such as cuprammonium hydroxide (as it does not contain the disulphide links present in other animal fibres). Each raw filament of silk is roughly elliptical in cross-section. After de-gumming the fibroin fibre is transparent and uniform in width being 9–12 μm with a smooth surface. Wild silk (Tussah Silk) fibres are darker in colour and coarser, approximately 28 μm, and less uniform in width, with pronounced longitudinal striations. Cross-sections of different silk fibres show significant differences, cultivated Bombyx being roughly triangular with the corners being rounded. Tussah silk on the other hand is wedge shaped, whilst Anaphe silk is roughly triangular and the apex of the triangle is elongated and bent. Because of silk’s outstanding strength, toughness, high regain, soft handle, excellent drape and appearance, it has a wide variety of uses in apparel, in particular high-qual- ity dress goods, lingerie and handkerchiefs. As silk loses about 25% of its weight during de-gumming, manufacturers add weight to the silk with tin salts, so called tin weighting, stannous chloride often being used. 20 Forensic Examination of Fibres 1.4.2.1 Spider Silk The protein fibre spun by spiders has superior mechanical properties compared to those of the silkworm. When compared to synthetic fibres such as polyamide and polyester, spi- der silk is said to be superior in strength, elasticity and resistance to compression. There are many specimens of spider silk, some exhibit elongation as high as 200% whilst oth- ers exhibit strength similar to high-performance fibres such as high tenacity polyamide. Spider silk has better water repellent properties compared to both types of natural silk. The domestication of spiders has not yet been possible, therefore its use in textiles has not been fully exploited. Being predators, the raising of spiders in dense populations is not possible. Compared to the silkworm the amount of silk produced by the spider is small. It has been possible to produce artificial spider silk. Currently DuPont has successfully spun a protein analogue of spider silk using solvent spinning technology and regenerating the fibre in a coagulation bath. Spider silk is sticky and may stick to textiles and could be mistaken for natural silk. 1.5 Mineral Fibres (Asbestos) Of the naturally occurring fibres asbestos is the most peculiar. Unlike other rocks that crystallise during their formation, asbestos is derived from a rock that crystallizes in the form of fibres that are closely packed together giving the surface a grainy appearance. The use of asbestos has been known since ancient times when it was used as wicks in lamps. There are three natural fibrous minerals: Anthophyllite: Magnesium-iron silicate but of least importance for commercial use. Amphibole: There are a number of varieties of this mineral, of which Crocidolite is the most important as it is from this mineral blue asbestos is extracted. Chemically the long flexible fibres are iron sodium silicate and are a blue colour. The average fibre length is 7.5–10 cm, but it is not as resistant to high temperatures as crysotile. Serpentine: Which occurs in two fibre forms is a hydrated silicate of magnesium. The most important of these is crysotile. It is green to brown in colour and the fibres extracted form the major world supply of asbestos. The crysotile fibres are strong, but the ultimate fibrils are very fine, about 25 nm in diameter. Asbestos has been spun into yarns and then woven into fire-resistant fabrics. Asbestos was used in manufacture of construction materials (asbestos cement sheet) but this has now been discontinued in Australia due to health considerations. Aramid polymer fibres such as NomexTM, and glass fibres have replaced asbestos in fire resistant fabrics and yarns. 1.6 Man-made Fibres Man-made fibre can be classified into two groups, those derived from natural polymers such as cellulose (the regenerated fibres) or true synthetic fibres synthesized from simple organic chemicals found in coal and oil. Methods of production vary with the different Fibres, Yarns and Fabrics 21 polymers. Essentially there are three methods of producing fibres from polymeric materi- als. These are; 1. Melt spinning: Applied only to thermoplastic polymers. The concentrated molten polymer is forced through tiny holes in a spinneret. As the polymer cools, the fila- ments are formed. 2. Dry spinning: The polymer is dissolved in a volatile organic solvent and the vis- cous solution pumped through the spinneret into a column of air where the sol- vent evaporates, leaving a continuous filament of fibre. The solvent is recovered for reuse in the process. 3. Wet spinning: The viscous polymer solution, dissolved in either an aqueous or organic solvent, is pumped through the spinneret, which is immersed in a bath containing suitable coagulating chemicals. The polymer precipitates in the bath; the extruded filaments are formed and drawn off ready for further processing. Following extrusion the filaments are stretched or drawn mechanically to orient the molecular chains along the longitudinal axis of the fibre. This stretching maximises the molecular forces between the molecular chains, increasing polymer crystallinity and fibre strength. The degree of crystallinity is a measure of the percentage of polymer present in its crystalline form, the balance being in a disordered or random state. The random state of a polymer is referred to as being an amorphous state. Within the fibre the crystalline and amorphous regions do not have any particular order but are randomly spaced along the fibre axis. The extent to which one or the other predominates will determine the ultimate properties of the fibre. The filaments can be cut into lengths (staple fibres) or remain continuous (continuous filament fibres); they can also be crimped so that they are compatible with the natural fibre yarn spinning system that may be used. Often synthetic fibres are blended with a natural fibre to enhance the properties of the final fabric. A small amount of twist is inserted into a filament yarn to aid in its further processing. Some synthetic fibre yarns are often further processed to increase the bulk and elasticity of the yarn. One such treatment is that of false twisting where high twist is inserted in the filaments as the yarn passes through a heating zone. The heat applied sets the twist, which is then untwisted, giving the yarn an increase in bulkiness in its relaxed state. 1.6.1 Fibres Regenerated from Natural Polymers Man-made fibres regenerated from natural polymers are sub-classified (refer to Figure 1.10) based on the source of the natural polymer (i.e. cellulose based or protein based). 1.6.1.1 Viscose Rayon Of all the regenerated cellulosic fibres viscose is by far the most important. There are many manufacturers throughout the world. In 2006 world production of viscose grew by about 10% to 2.7 million tons, China being the largest consumer at 1.5 million tons*. Viscose rayon is manufactured from wood pulp, usually made from spruce wood. During the * Source: www.worldresearchandmarkets.com. 22 Forensic Examination of Fibres Fibres Natural Man-made Animal Vegetable Organic Inorganic Transformation of natural polymers Synthetic polymers Cellulose based Protein based Rayon (viscose, cupro, lyocell, modal) Casein Cellulose ester (acetate, triacetate) Soya protein fibre Figure 1.10 Sub classification of major man-made fibres from natural polymers. preparation of the pulp using the acid sulphite process, the lignin is removed by sulphona- tion as a lignosulphonic acid. The cellulose chains are shortened due to the degradation that takes place. The degree of polymerisation (DP) of Spruce is about 2400; this is too large for fibres. After pulping, the DP is 1400 and the degree of polymerisation in the final viscose fibre is 200–400 compared to cotton at 300–4000. At this stage the α-cellulose content is too low and the lignin content too high so the pulp is bleached using sodium hypochlorite. The pulp is then beaten to separate the cellulose fibres, the slurry of pulp and water run out onto a gauze belt where the water is extracted using vacuum extraction. The fibres in the pulp are held together by strong hydrogen bonds between the chains. The non- crystalline (amorphous) regions have random distribution of hydrogen bonds that are not numerous. The pulp is then treated with alkali to form alkali cellulose (see Equation 1.1) and the mixture allowed to age to reduce the polymer chain lengths. The aging is carefully controlled to produce chain lengths short enough to give optimum viscosity in the spin- ning process but still long enough to impart good physical properties to the fibre product. R = cellulose chain OH = any one of 3 –OH groups in the glucose residue R − OH + NaOH → R − ONa + H2O (1.1) (alkali cellulose) R − ONa + CS 2 → R − O.CSS Na (1.2) 2R − O.CSSNa + H2SO 4 → 2R − O.CSSH + 2Na 2SO 4 → 2R − OH + 2CS 2 (1.3) The alkali cellulose is reacted with carbon disulphide (Equation 1.2) to form the cellu- lose xanthate, which is completely soluble in 7% sodium hydroxide, giving a viscous liquid, which is the spinning solution. The spinning solution is squirted through a spinneret into a bath containing sulphuric acid (Equation 1.3), where the filaments harden, initially from the outside. As regeneration occurs toward the inside of the fibre the fibre shrinks giving rise to a wrinkled cross-section (refer to Figure 1.11a and b). Viscose has a much lower crystallinity, about 35%–40%, compared to cotton 65%– 70%, which results in a much weaker fibre in both the dry and wet states. Viscose fabrics Fibres, Yarns and Fabrics 23 Figure 1.11 (a and b) Cross-section of viscose fibre showing wrinkled appearance and Viscose spinneret, diameter 26 mm with 200 holes. have good drape but tend to feel cold compared to cotton. Viscose is amorphous in nature with a large number of polar hydroxyl groups in the polymer, which results in a fibre with higher moisture-absorbing properties. Viscose has much lower resistance to acids and bleaching agents than cotton and is discoloured and weakened when attacked by mould and fungi. 1.6.1.2 Bamboo Bamboo fibre is a type of viscose rayon manufactured from cellulose pulp derived from the bamboo plant. Its properties of moisture absorbency, tensile strength and extensibil- ity are similar to those of normal viscose. It is interesting to note that it is not possible to determine the origin of the cellulose (i.e. bamboo or spruce) once it has been through the viscose process. 24 Forensic Examination of Fibres Bamboo has become increasingly popular as a fibre for garments and is marketed as environmentally friendly due to the renewable source. The major source of bamboo for fibre manufacture is grown in China with plants growing up to 30 m at a rate of 0.3 m per day. There are more than 70 genera divided into about 1450 species. The cellulose content of the bamboo plant is about 61% and lignin content approximately 32%. Although the bamboo itself is a renewable resource, the chemical process required to produce a fibre useable in a textile can have serious implications for the environment. Bamboo fibre is predominantly produced using a process similar to that for mak- ing viscose where the woody stalks are steamed and then mechanically crushed. Sodium hydroxide solution is added to produce a pulp that is then treated with carbon disulphide to produce the cellulose xanthate, which is then extruded into a bath of dilute acid. There is an emergence in legislation in relation to labelling bamboo products with two governing bodies (the Canadian Competition Bureau and the U.S. Federal Trade Commission) stipulating that products must be labelled as rayon with the optional quali- fier of ‘from bamboo’. 1.6.1.3 Improved Forms of Viscose 1.6.1.3.1 High Wet Modulus Rayon An improved form of Viscose was developed by LENZING as a high wet modulus viscose under the trade name MODAL ® for many uses. To produce this high tenacity viscose the cellulose is regenerated slowly. Slow regeneration is accomplished by increasing the amount of Zinc Sulphate and lowering the acid concen- tration in the coagulating bath, at the same time a higher degree of stretch is applied. The higher stretch results in a more crystalline fibre; the filament is all skin and has no core. This improved form of viscose has lower water absorption and swelling and has mechanical properties that are much closer to cotton. They have a fine micro-fibrillar structure resistant to 8% aqueous sodium hydroxide solution. Characteristically these fibres have higher wet strength, increased resistance to swelling in alkaline solutions, a higher degree of polymerisation DP and a more fibrillar internal structure similar to cot- ton. Typically the DP would be 500–700 (regular viscose has a DP of 250–400) with the fibres being 50%–60% crystalline with larger crystallites than in cotton. Because of the higher crystallinity and the higher orientation these fibres are less likely to deform under wet conditions compared to regular viscose. 1.6.1.3.2 Lyocell Lyocell is a regenerated cellulosic fibre made by spinning from a sol- vent. The development of the process of dissolving cellulose in a tertiary amine N-oxide was patented in 1989 by Akzona, Inc. (now Akzo Nobel) and the process licensed to Courtauld’s and Lenzing. Lyocell fibres are sold under the trade name ‘TENCEL ®’. This development has to a large extent resolved two of the major issues in relation to regener- ated cellulose (viscose) production, that of poor wet strength and environmental pollution. Little has been divulged regarding the manufacturing process for Tencel. What is known of the process is that the regenerated cellulose is produced directly from a solution of cellulose rather than from a cellulose derivative, which increases the degree of polymeri- sation. Raw cellulose is dissolved directly in a solvent, amine oxide, the solution is filtered, extruded into a dilute amine oxide solution and coagulated into fibres. The technology used also allows the recovery of the solvent and the reuse of most of the process water. Lyocell fibres have better extensibility than cotton, higher crystallinity (resulting in greater tenacity) and a wet strength that is 2–3 times greater than regular viscose (refer to Fibres, Yarns and Fabrics 25 Table 1.5 for comparison of properties of cotton, viscose and lyocell). Because the fibres have a fibrillar structure the fibrils will separate when the fibres are wet and when under tension. Fibrillation is the longitudinal splitting of the fibre into a bundle of micro fibres of smaller diameter usually 1–4 μm. This occurs mainly at the fibre surface and usually occurs during processing in rope form either in a winch or jet-dyeing machine. The degree of fibrillation can be controlled during processing and allows the production of attractive fabric surface effects such as peach skin with a soft pleasant handle. Tencel has been used in a range of garment types including sportswear, mens’ shorts and trousers. 1.6.1.4 Esters of Cellulose Schutzenberger in 1869 first prepared cellulose acetate, but it was Cross & Bevan in 1894 who described a practical method of production. In 1903 it was discovered that cellulose acetate could be dissolved in the relatively cheap and safe solvent acetone. Early produc- tion of cellulose acetate in Britain was carried out by the brothers Dreyfus at their factory in Spondon, Derby. Initial production of the cellulose acetate was as a plasticised lacquer (dope) for the wings of aircraft. At the end of the First World War the brothers Dreyfus found themselves with a large factory and no demand for aircraft dope. By 1921 they had ironed out most of the technical difficulties and cellulose yarn was being marketed in Britain with the name ‘Celanese’. The development of cellulose acetate was a major break- through in the development of alternatives to natural silk. Cellulose is reacted with acetic anhydride and acetic acid in the presence of sulphuric acid. Initially the cellulose is fully acetylated, all –OH groups are acetylated and cellulose triacetate or 6/6 acetate is formed. Whilst fibres could be made from cellulose triacetate, and were by the Lustron Company, it ceased production in 1915 because the fibres could not be dyed with existing dyes and the solvents used (chloroform) in production of the fibre were not satisfactory. Courtauld’s work in the 1950’s resulted in the production of ‘Courpleta’, dry spun cellulose triacetate using methylene chloride as the solvent. To hydrolyse to a 5/6 acetate, the triacetate is run together with the excess acetic anhy- dride and acetic acid into water so that a 95% solution of acetic acid results. The solution is warmed to 50°C so that the water can remove some of the acetyl groups. This acid hydro- lysis takes place over a period of about 20 hours. At this stage the product becomes soluble in acetone, which has a boiling point of 56.5°C. The Degree of Polymerisation is 350–400. By acetylation the possibility of hydrogen bonding has been removed and therefore the water absorption properties are greatly reduced. The following illustrates the effect of hydrogen bonding on the water absorption properties of both natural and regenerated Table 1.5 Comparison of Physical Features of Viscose, Lyocell and Cotton Viscose Lyocell Cotton Tenacity (cN tex-1) Dry 18–22 37–41 20–24 Wet 11–13 30–35 26–30 Moisture regain (%) at 20°C 65% RH 12–14 11–12 8.5 Extensibility (%) Dry 17–25 13 7–9 Wet 23–38 15 12–14 Melting Point (°C) Does not melt Does not melt Does not melt Softening point (°C) 130 none none Handle Soft and cold Soft and Silky Soft and warm 26 Forensic Examination of Fibres cellulosic fibres when measured at 20° C and 65% relative humidity, cotton 8.5%, viscose 13.0%, secondary acetate 6.5% and triacetate 4.5%. Acids will hydrolyse the fibres and the acetyl group can be saponified by alkali leading to fibre yellowing. The secondary acetate is soluble in acetone but not soluble in chloroform and the triacetate because of its higher degree of acetylation is soluble in methylene chloride and chloroform. Both secondary acetate (5/6 acetate) and triacetate are thermoplastic and can be heat sett to give a permanent pleat. Triacetate is more extensively affected by heat setting than the secondary acetate. Secondary acetate has been used extensively as linings in suitings and in satin fabrics destined for wedding apparel. Blends of triacetate with polyester in knitted fabrics have been popular in the past. 1.6.1.5 Regenerated protein fibres Casein: Acid treatment of milk coagulates the protein casein into curd which is then washed and dried. The casein fibres are spun from an alkaline solution of the casein into an acid coagulating bath which may also contain formaldehyde. The coagulated filaments are stretched during the coagulation process. Initially the filaments are soft and weak and require further hardening in formaldehyde to increase their stability to water and alkali and give adequate strength to the fibre. Fibres were manufactured as Merinova in Italy, Fibrolane in the UK and Lanital in France. Casein fibres have a silk-like appearance and are insoluble in cold concen- trated hydrochloric acid which will dissolve silk. Soya protein fibre (SPF): The development of this fibre was pioneered by the Ford Motor Company, production commencing in 1939. The manufacturing process is similar to that of casein fibre. However production in the United States ceased after several years. More recently Harvest SPF Textile Co., Ltd., have been manufactur- ing and marketing soya protein fibre. The fibre has a soft cashmere-like handle and is ideally suited for knitted fabrics. The fibre cross-section is a dumb-bell in shape which gives it natural lustre. The fibre is readily dyed with acid and reactive dyes with colour yields and fastness properties similar to wool. SPF fibre has a moisture regain of 8.6%, which is comparable to that of cotton. Acid and alkali resistance are similar to that of wool. 1.6.1.6 Alginate Fibres Sodium alginate solution extracted from seaweed is extruded into a coagulating bath con- taining a calcium salt. This precipitates the fibre as the calcium alginate, which is non- flammable, but soluble in alkaline solutions. Alginate fibres have been used as a support yarn to produce ultra light-weight fabrics, when the alginate yarn is dissolved out in the finishing process. Alginate fibres are used in surgical dressings which are non toxic and readily absorbed into the blood stream. The fibre is soluble in sodium carbonate and has high ash content. 1.6.2 Synthetic Fibres True synthetic fibres are manufactured from two polymer types, condensation polymers and addition polymers. Figure 1.12 shows the sub classification of synthetic fibres. Condensation polymers are prepared from the condensation reaction of two mono- mers having two functional groups from which a simple molecule is eliminated. Polyesters, Fibres, Yarns and Fabrics 27 Fibres Natural Man-made Animal Vegetable Organic Inorganic Transformation of natural polymers Synthetic polymers Polyester Polyamide Polyolefin Polyvinyl Polyurethane Aramid -Polyethylene -Nylon -Polypropylene -Acrylic -Segemented -Kevlar terephthalate -Polyethylene -Modacrylic -Non segmented -Nomex (PET) -Chlorofibre (PVC) -Polybutylene -Vinylal (PVA) terephthalate -Fluorofibre (PBT) -Polytrimethylene terephthalate (PMT) Figure 1.12 Sub classification of synthetic polymer fibres. polyamides, polyurethanes and aramids are examples of linear polymers produced by this process. The repeating units in the polymers’ chain are joined by functional groups such as amide and ester which can undergo hydrolysis by acids and alkalis resulting in polymer degradation. Addition polymers are formed by the direct addition of the monomer to itself without the elimination of any molecules. Monomers that undergo addition polymerisation must contain double bonds. Addition polymers are more crystalline and have a much higher degree of polymerisation than condensation polymers and because the C-C bonds along the molecular chain are strong there are no sites for easy access to corrosive chemicals that would cause cleavage of the chain. Polymers formed by the addition method have the same number of atoms in the struc- tural unit as the monomer from which the polymer has been made. Typical examples of addition polymers are polyolefins and the polyvinyls (the acrylic polymers). Polymers can be linear where there is one single repeating unit as in polyethylene or they may be branched chain polymers. Some branched chain polymers may link two linear chains to form a cross-linked structure. Internal cross-linking of polymers in cotton and viscose improve the dimensional stability of the thereby reducing the tendency of the fabric to shrink). Polymers may also be classified as Atactic, Isotactic and Syndiotactic. 1. Atactic polymers possess a random distribution along the polymer chain of the two asymmetrical carbon atoms; because they are not orderly structures they will not crystallise hence are not suitable for fibres. X X X – CH2 – CH – CH2 – CH –CH2 – CH – CH2 – CH – CH2 – CH – CH2 – CH – X X X 28 Forensic Examination of Fibres 2. Isotactic polymers have a regular arrangement of the substituent groups, i.e. where all the groups X are disposed in the same fashion. Isotactic polymers are of con- siderable interest as they have higher melting points than the normal atactic mate- rials, e.g. atactic styrene has a melting point of 85°C compared to 230°C for the isotactic styrene. – CH – CH2 – CH – CH2 – CH – CH2 – CH – CH2 – X X X X 3. Syndiotactic polymers have a regular arrangement of the substituent groups. X X CH2 – CH – CH2 – CH – CH2 – CH – CH2 – CH – CH2 – CH – CH2 – X X X 1.6.2.1 Polyesters Polyester fibres, the most common being polyethylene terephthalate (PET)*, dominate the global synthetic fibre industry. Polyester fibre represented 77% of world synthetic fibre pro- duction in 2004 with an expected annual growth of 7% over 10 years†. There are a large number of manufacturers of polyester, the majority being in Asia. Typical trade names are Dacron, Terylene, Fortrel, Terigal, Teteron, Trevira, Grilene, Diolen and Tergal. Polyesters are a very versatile fibre and have extensive end product uses from carpets, industrial fibres and yarns for tyre cords, to car seat belts, sailcloth, and fabrics as well as apparel and household furnishing fabrics. The condensation polymer is synthesized from a dibasic acid (teraphthalic acid) and a diol (ethylene glycol) this combination of monomers results in a fibre of high melting point and good stability to chemical attack. ( n + 1) R ( OH )2 + n R ′ ( COOH )2 → HO[ROOCR ′COO ]n ROH + 2n H2O Polyester is highly crystalline (65%–85%) and hydrophobic in character, which results in very low moisture regain, 0.4%–0.5% at 20°C and 65% relative humidity. The presence of the aromatic nucleus in the chain increases the melting point (249°C–250°C) and the chemical stability. Stability to concentrated acids is excellent but the ester linkage is hydro- lysed by alkali. Alkaline hydrolysis with sodium hydroxide of the surface is used in the weight reduction of polyester fabrics, this process in common to polyester woven georgette. The surface hydrolysis of the fibre improves the drape as well as giving the fibre improved antistatic properties. The fibre is insoluble in most organic solvents, but will dissolve in σ-chlorophenol and hot meta-cresol. Polyester is melt spun and produced as filament yarns and staple fibre. Staple fibre lengths vary depending on the spinning system, the shortest staple being made for the cot- ton system. Filament yarns are textured to increase the bulk, some staple fibre is crimped, * Other forms of polyester include polybutylene terephthalate (PBT) and polytrimethylene terephthalate. †Journal for Asia on Textile & Apparel – June 2006. Fibres, Yarns and Fabrics 29 again to increase the bulk and make it more suitable for spinning with wool. The fibre is de-lustred with titanium dioxide, the quantity being varied depending on whether the yarn is dull or bright. Yarn cross-sections can vary depending on the configuration of the holes in the spinneret. Polyester and polyester blended fabrics can be heat set to give dimensional stability, and prevent shrinking during domestic laundering. Fabrics made from polyester are more stable to ultraviolet light than a comparable product made from nylon. The blending of polyester staple fibre with wool and cotton take advantage of the easy care properties of the fibre and increase its suitability in a wide variety of furnishing and fashion fabrics. Disperse dyes are used for the dyeing of polyester yarns and fabrics; some filament yarns are coloured during the spinning process, pigments being added to the polymer melt to accomplish this technique. High tenacity continuous filament yarns are used for ropes, conveyor belts, automotive seat belts and tarpaulins. Polyesters are available that have been modified by copolymerisation with an acidic monomer to make them dyeable with cat- ionic (basic) dyes. Trevira CS is modified polyester that is inherently flame retardant that has been used in home furnishings, particularly drapes and upholstery fabrics. The introduction of polyester microfibres in the late 1980’s has enhanced the per- formance properties of the fibre. Microfibres are generally regarded as being finer than 1 Decitex per filament [dtexpf]*. Microfibres enable fabrics to be made that have a tighter construction, lighter weight, improved drape and greater softness similar to that avail- able from natural fibres. The diameters of a number of common fibres are compared in Table 1.6†. To date the major application of microfibres has been in apparel, particularly sports wear and active wear, where garments are required to be both windproof and permeable to perspiration. There have been a number of innovations in the production of polyester fibres, prin- cipally from ‘INVISTA CORPORATION‡’ of the United States. These innovations have principally been in the modification of the extrusion technique. These modifications pro- duce specific variations in cross-section to enhance the performance of the fibre. The two fibres in question are Type 704EF and Type 727. Type 704EF is sold under the trade name COOLMAX® (refer to Figure 1.13) the registered trade name of INVISTA. This modified cross-section improves the transport of moisture and results in a garment with improved comfort particularly for sporting apparel. Type 727EF is marketed as THERMOLITE ® (refer to Figure 1.14) the cross-section of this fibre has a hollow core resulting in increased thermal insulation properties. The world of fibre production has undergone dramatic change in the past two decades with many long established chemical companies exiting from the fibre production industry. These include household names such as ICI, Hoechst, Monsanto and Eastman. INVISTA emerged from DuPont. Geographically, production has moved from North America to Asia and other emerging economies. New fibre producers will now buy polyester polymer as a commodity convert it into fibre and yarn, Hence, the old regime of end-to-end produc- tion from chemicals to fibres to end products has dramatically changed forever. As a result, * Decitex = weight in grams of 10,000 metres of yarn. †Dr.Glover, B., October 2004. Microfibres. In SDCANZ Conference, Christchurch, New Zealand. ‡ LYCRA®, COOLMAX®, THERMOLITE ®, TACTEL ® and Antron® are registered trademarks of INVISTA. 30 Forensic Examination of Fibres Table 1.6 Diameters of Some Common Fibres Fibre Diameter (micron) Human hair 30–100 Wool 15–49 Regular polyester 15 Cotton 6–13 Silk 5–8 Microfibre 2.5–5 Super/Ultra fine Less than 2.5 Microfibre Figure 1.13 Cross section of COOLMAX® FABRIC (Type 704EF) fibres. new players and new fibres are emerging. Polyester will continue to evolve as it is a cheaper polymer to manufacture than, for example, polyamide (nylon). 1.6.2.2 Polyamides Nylon 66 is a condensation polymer made from a dicarboxylic acid (adipic acid) and a diamine (hexamethylene diamine). The 66 in Nylon 66 refers to the number of carbon atoms in each of the monomers. The final polyamide contains polar amide groups along the polymer chain that allow many hydrogen bonds to form between adjacent polymer chains. Since there are no side groups present hydrogen bonding is maximised resulting in a highly oriented crystalline structure with good tensile strength. Nylon 6 is manufactured by the polymerisation of ε-caprolactam yielding the polymer H ⎡⎣ − NH − ( CH2 )5 CO − ⎤⎦ n OH Comparative properties of nylon 6, nylon 66 and polyester are shown in Table 1.7. Fibres, Yarns and Fabrics 31 Figure 1.14 Cross section of THERMOLITE® FABRIC (Type 727) fibres. The highly crystalline nature of the polymer reduces the tendency for the polar amide groups in the polymer chain to attract water into the non-crystalline amorphous parts of the chain. From Table 1.7 it can be seen that on wetting the fibre loses about 10%–20% of its strength due to the water disrupting the hydrogen bonding between the molecular chains. A consequence of the low moisture absorbency of nylon is its tendency to build up static electricity. This can be overcome by the addition of hygroscopic chemicals to the fibre surface during finishing. A novel approach to controlling static in nylon carpets was the introduction of a fine copper thread into the carpet backing. When exposed to sunlight over a prolonged period the fibre is degraded by oxidation of the amide groups resulting in a substantial; lowering of strength. The cross section of nylon can be highly varied. Some nylons are marketed with a cir- cular cross section, while others may have a tri-lobal cross section. For example a tri-lobal cross section is marketed as white tri-lobal nylon by INVISTA and is used extensively for the manufacture of tufted carpets. Cross sectional shape is determined by the shape of the spinnerets through which the fibre forming substance is extruded (refer to Figure 1.15a and b). Nylon fibres are melt extruded. The purpose of the different cross sectional shapes is to enhance bulk and improve the performance of the fibre in the end products. A popular carpet fibre Antron® from INVISTA uses a square cross section fibre with four internal holes. This shape is pro- duced by an unusual spinneret of two ‘I’ shapes at right angles with extended bars at the top and bottom. Nylon can be heat sett either with dry heat or steam. The heat setting process disrupts the hydrogen bonds which are then reformed in new positions when the fabric or yarn is cooled. Heat setting is usually carried out under controlled stress conditions. Both nylon 6 and 66 are reasonably stable to alkalis, but are soluble in concentrated acids (formic acid) and phenols. Nylon 6 is soluble in 4.4N Hydrochloric acid which distinguishes it from nylon 66. Nylon is readily dyed with acid and premetallised dyes. Pale shades can be dyed with disperse dyes due to their very even dyeing properties. Blends of nylon with wool are com- mon in the carpet industry, usually an 80% wool 20% nylon blend. In sportswear, blends of nylon with elastomeric fibres such as Lycra® are common, the amount of elastomeric fibre 32 Forensic Examination of Fibres Table 1.7 Comparison of Features of Nylon 6, Nylon 66 and Polyester Fibres Nylon 6 Nylon 66 Polyester -1 Tenacity (cN tex ) Dry 39–51 40–51 35–85 Wet 36–45 35–45 35–85 Moisture regain (%) at 4–4.5 4–4.5 0.4–0.6 20°C 65% RH Extensibility (%) 23–42 26–32 8–30 Melting Point (°C) 210–216 252–260 249–260 Specific gravity (g/cm3) 1.14 1.14 1.38 Solubility cold conc. cold conc. hot Formic acid Formic acid meta-cresol varying up to 20%. Nylon hosiery and support hose are common uses of the fibre. Many swimwear manufacturers use nylon containing fabrics in their products. Tufted nylon car- pets are a common application due to the very high abrasion resistance of the fibre. High tenacity nylons with lower extensibility are produced by increased stretching after extrusion. These are used in ropes, conveyor beltings and parachute fabrics. A number of other nylon fibres noted for their abrasion resistance and high thermal stability have been developed for use in engineering these include nylons 6.10, 7, 11 and 4.6. Dupont developed a soft silky nylon under the trade name Qiana in 1968 for the high fashion trade. The fabric became popular in the 1970’s, it had higher moisture regain due to the additional polar groups incorporated into the polymer. Cost may well have been the cause of the cessation of production. Typical trade names for Nylon 6.6 are Antron®, Rhodiastar, TACTEL ®, Ultron and for Nylon 6 Perlon, Enkalon, Patina, Ansa and Zeftron. 1.6.2.3 Polyolefins Polyethylene (polyethene) and polypropylene (polypropene) are manufactured by addition polymerisation from the common petroleum products ethene and propene. The two poly- mers are manufactured by free radical polymerisation under conditions of high tempera- ture and pressure to low-density polyethylene (LDPE) and atactic polypropylene. Atactic polypropylene, being an irregular structure, cannot crystallise and is therefore unsuitable for fibres. Isotactic and syndotactic polypropylene are regular structures polymerised using the special Natta-Ziegler catalyst which enables polymerisation to take place at a much lower pressure (30atm) and temperature (100°C). This process allows for chain packing and there is no possibility of side chain or branch chain formation, thus a highly crystalline fibre, 90% crystalline in the case of the isotactic polymer, can be produced. Polyethylene can be produced in the highly crystalline (85%) high-density form. Because of the densely packed polymer chains the interchain forces have the effect of giving the highly drawn fibre high tenacity and good elongation similar to that of high-tenacity nylon and polyester. Both polyethylene and polypropylene are melt spun, into both filaments and sheet film. When extruded into sheet the film is slit into tapes that can be handled like yarn. Since polyolefin fibres do not absorb moisture, they are very difficult to dye and are resistant to attack by acids and alkalis. Fibre colouration is by mass pigmentation, i.e. pig- ment is added to the polymer melt before extrusion, however a number of polypropylene Fibres, Yarns and Fabrics 33 (a) (b) Figure 1.15 (a) spinneret for melt spinning trilobal Nylon fibres, (b) Spinneret profile for tri- lobal Nylon fibre. fibres have been chemically modified to make them dyeable with disperse, acid and pre- metallised dyes. The hydrophobic nature of polyolefins results in low moisture absorbency giving the fibre water transport properties that enables moisture to be wicked away from the fibre surface without being trapped within the fibre itself. Active wear when worn next to the skin takes advantage of this property. Compared to nylon and polyester, polyethylene and polypropylene have relatively low melting points being 135°C and 165°C respectively, which makes them unsuitable for many textile appli- cations. Being hydrocarbons, polypropylene is flammable and burns rapidly with black smoke. Polypropylene when extruded as sheet film is cut into strips approximately 2–3 mm in width. In this form the fibre can be used as a yarn and woven into an industrial weight fabric that is used as a primary backing in tufted carpets and in sacks. Wider strips up to 50 mm wide are formed into a yarn by twisting and are used as varying types of cordage such as ropes and fishing nets. Warp-knitted polypropylene is finding extensive use as sun awnings, i.e. shade cloths, the polymer having been treated with a suitable ultraviolet stabiliser to minimise degradation. There are many textile applications for polypropylene fibres; these include carpets, furnishings, synthetic grass (particularly for tennis courts). In apparel the very low density of the fibre makes it ideally suited for light-weight sports cloth- ing. Thermally bonded non-woven products have been developed for medical applications, the material is light weight, absorptive and resistant to bacteria. Recent developments include a new fibre from BASF Performance Chemicals MOOOTM. This polypropylene fibre is dyeable by conventional means with disperse dyes, is light weight, and controls odour and bacterial growth. Other developments include ultra-high-strength polyethylene fibre with the strength similar to aramides, DyneemaTM from DSM Nederlands BV; being light weight and ultra strong it is being manufactured into cargo nets for the airline industry resulting in fuel savings. Trade names include Dyneema, Polysteen, Spectra and Vestolan for polyethylene, and for polypropylene Danaklon, Asota, Downspun, Gymlene, Merkalon, MOOOTM, Novatron and Vegon. 34 Forensic Examination of Fibres 1.6.2.4 Polyvinyl Fibres 1.6.2.4.1 Acrylic Fibres There are two types of acrylic fibres, both polymerised from acrylonitrile. Acrylic fibres contain at least 85% by weight of acrylonitrile and modacrylics contain at least 35% but not more than 85% acrylonitrile. Production of modacrylic fibre was begun by Union Carbide in 1949. Polyacrylonitrile had been known for some time, but for making fibres it suffered from the defect of being insoluble in common solvents. Because its softening temperature was close to its decomposition point it was precluded from melt spinning. It was found that hydrotropic solvents such as calcium thiocyanate would dissolve polyacrylonitrile and polar organic solvents such as dimethyl formamide and tetramethyl suphone were also suitable. Since 1943 polyacrylonitrile and its copolymers have been manufactured into fibres. These are marketed under various trade names; some of the common fibres available have the names Orlon, Courtelle, Acrilan, Cashmilon, Dolan, Dralon, Leacril and Vonnel. Polymeri