Food Safety Handbook PDF
Document Details
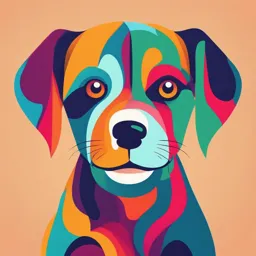
Uploaded by FeistyAloe
Ronald H. Schmidt and Gary E. Rodrick
Tags
Related
- Food Safety Handbook PDF
- Food Safety Handbook PDF
- Risk Management Concepts and Principles PDF
- Occupational Safety and Environmental Safety for the Food Industry Lecture 2 PDF
- Occupational Safety and Environmental Safety for the Food Industry Lecture 3 PDF
- Occupational Safety And Environmental Safety For The Food Industry Lecture 4 PDF
Summary
This handbook details food safety issues, covering biological, chemical, and physical hazards. It explores risk analysis frameworks, dose-response modeling, and economic consequences. The book provides a comprehensive understanding of food safety for professionals in the industry and regulation.
Full Transcript
FOOD SAFETY HANDBOOK FOOD SAFETY HANDBOOK RONALD H. SCHMIDT and GARY E. RODRICK A JOHN WILEY & SONS PUBLICATION Copyright (” 2003 by John Wiley & Sons, Inc. All rights reserved. Published by John Wiley & Sons, Inc., Hoboken. New Jersey Published simultaneously in Canada. N o part of this...
FOOD SAFETY HANDBOOK FOOD SAFETY HANDBOOK RONALD H. SCHMIDT and GARY E. RODRICK A JOHN WILEY & SONS PUBLICATION Copyright (” 2003 by John Wiley & Sons, Inc. All rights reserved. Published by John Wiley & Sons, Inc., Hoboken. New Jersey Published simultaneously in Canada. N o part of this publication may be reproduced. stored in a retrieval sqstem, or transmitted in an) form or by any means. electronic, mechanical, photocopying, recording, scanning. or otherwise. except as permitted under Section 107 or 108 of the 1976 United States Copyright Act, without either the prior written permission of the Publisher, or authorization through payment of the appropriate per-copy fee to the Copyright Clearance Center, Inc., 222 Rosewood Drive. Danvers MA 01923. 978-750-8400, fax 978-750-4470. or on the web at www.copyright.com. Requests to the Publisher for permission should be addressed to the Perniissions Department, John Wiley & Sons. Inc.. 11 1 River Street, Hoboken, NJ 07030, (201) 748-601 1, fax (201) 748-6008, e-mail: permrccl~~wiley.com. Limit of Liability/Disclairner of Warranty: While the publisher and author have used their best efforts in preparing this book, they make no representations or warranties with respect to the accuracy or completeness of the contents of this book and specifically disclaim any implied warranties of merchantability or fitness for a particular purpose. No warranty may be created or extended by sales representatives or written sales materials. The advice and strategies contained herein may not be suitable for your situation. You should consult with a professional where appropriate. Neither the publisher nor author shall be liable for any loss of profit or any other commercial damages, including but not limited to special, incidental, consequential, or other damages. For general information on our other products and services please contact our Customer Care Department within the U.S. at 877-762-2974, outside the U.S. at 317-572-3993 or fax 31 7-572-4002. Wiley also publishes its books in a variety of electronic formats. Some content that appears in print. however, may not be available in electronic format. Library of Congress Catali~ging-in-PublicariNgData is uvailuble: ISBN 0 - 4 7 1 - 2 1 0 6 4 - 1 Printed in the United States of America 10 9 8 7 6 5 4 3 2 1 CONTENTS Preface xi PART I CHARACTERIZATION OF FOOD SAFETY AND RISKS 1 Edited by Joan Rose 1 DEFINITION OF FOOD SAFETY 3 Robert (Skip) A. Seward I/ 2 CHARACTERIZATION OF FOOD HAZARDS 11 Robert (Skip) A. Seward /I 3 RISK ANALYSIS FRAMEWORKS FOR CHEMICAL AND MICROBIAL HAZARDS 19 Margaret E. Coleman and Harry M. Marks 4 DOSE-RESPONSE MODELING FOR MICROBIAL RISK 47 Chuck Haas 5 EXPOSURE ASSESSMENT OF MICROBIAL FOOD HAZARDS 59 Richard C. Whifing V Vi CONTENTS 6 EXPOSURE AND DOSE-RESPONSE MODELING FOR FOOD CHEMICAL RISK ASSESSMENT 73 Carl K. Winter 7 ECONOMIC CONSEQUENCES OF FOODBORNE HAZARDS 89 Tanya Roberts, Jean Buzby, and Erik Lichtenberg PART I1 FOOD HAZARDS: BIOLOGICAL 125 Edited by LeeAnne Jackson 8 PREVALENCE OF FOODBOURNE PATHOGENS 127 LeeAnne Jackson 9 PHYSIOLOGY AND SURVIVAL OF FOODBOURNE PATHOGENS IN VARIOUS FOOD SYSTEMS 137 G.E. Rodrick and R.H. Schmidt 10 CHARACTERISTICS OF BIOLOGICAL HAZARDS IN FOODS 157 R. Todd Bacon and John N. Sofos 11 CONTEMPORARY MONITORING METHODS 197 Jinru Chen PART Ill FOOD HAZARDS: CHEMICAL AND PHYSICAL 21 1 Edited by Austin R. Long and G. William Chase 12 HAZARDS FROM NATURAL ORIGINS 213 John J. Specchio 13 CHEMICAL AND PHYSICAL HAZARDS PRODUCED DURING FOOD PROCESSING, STORAGE, AND PREPARATION 233 Heidi Rupp 14 HAZARDS ASSOCIATED WITH NUTRIENT FORTIFICATION 265 Anne Porada Reid CONTENTS vii 15 MONITORING CHEMICAL HAZARDS: REGULATORY INFORMATION 277 Daphne Santiago 16 HAZARDS RESULTING FROM ENVIRONMENTAL, INDUSTRIAL, AND AGRICULTURAL CONTAMINANTS 291 Sneh D. Bhandari PART IV SYSTEMS FOR FOOD SAFETY SURVEILLANCE AND RISK PREVENTION 323 Edited by Keith R. Schneider 17 IMPLEMENTATION OF FSlS REGULATORY PROGRAMS FOR PATHOGEN REDUCTION 325 Pat Sfolfa 18 ADVANCES IN FOOD SANITATION: USE OF INTERVENTION STRATEGIES 337 Judy W. Arnold 19 USE OF SURVEILLANCE NETWORKS 353 Craig W. Hedberg 20 HAZARD ANALYSIS CRITICAL CONTROL POINT (HACCP) 363 Debby Newslow PART V FOOD SAFETY OPERATIONS IN FOOD PROCESSING, HANDLING, AND DISTRIBUTlON 381 Edited by Barry G. Swanson 21 FOOD PLANT SANITATION 383 Henry C. Carsberg 22 FOOD SAFETY CONTROL SYSTEMS IN FOOD PROCESSING 403 Joellen M. Feirtag and Madeline Velazquez 23 FOOD SAFETY AND !NNOVATIVE FOOD PACKAGING 41 1 Michael L. Rooney Viii CONTENTS 24 SAFE HANDLING OF FRESH-CUT PRODUCE AND SALADS 425 Dawn L. Hentges 25 GOOD MANUFACTURING PRACTICES: PREREQUISITES FOR FOOD SAFETY 443 Barry G. Swanson PART VI FOOD SAFETY IN RETAIL FOODS 453 Edited by Ronald H. Schmidt and Gary E. Rodrick 26 COMMERCIAL FOOD SERVICE ESTABLISHMENTS: THE PRINCIPLES OF MODERN FOOD HYGIENE 455 Roy Costa 27 INSTITUTIONAL FOOD SERVICE OPERATIONS 523 Ruby P. Puckett 28 FOOD SERVICE AT TEMPORARY EVENTS AND CASUAL PUBLIC GATHERINGS 549 Donna L. Scott and Robert Gravani PART VII DIET, HEALTH, AND FOOD SAFETY 57 1 Edited by Mary K. Schmidl 29 MEDICAL FOODS 573 Mary K. Schmidl and Theodore P. Labuza 30 FOOD FORTIFICATION 607 R. Elaine Turner 31 SPORTS NUTRITION 627 Joanne L. Slavin 32 DtETARY SUPPLEMENTS 641 Cathy L. Bartels and Sarah J. Miller 33 FUNCTIONAL FOODS AND NUTRACEUTICALS 673 Ronald H. Schmidt and R. Elaine Turner CONTENTS iX PART Vlll WORLD-WIDE FOOD SAFETY ISSUES 689 Edited by Sara E. Valdes Martinez 34 INTERNATIONAL ORGANIZATION FOR STANDARDIZATION IS0 9000 AND RELATED STANDARDS 691 John G. Surak 35 IMPACT OF FOOD SAFETY ON WORLD TRADE ISSUES 725 Erik Lichtenberg 36 UNITED STATES IMPORT/EXPORT REGULATION AND CERTIFICATION 741 Rebeca Lopez-Garcia 37 EUROPEAN UNION REGULATIONS WITH AN EMPHASIS ON GENETICALLY MODIFIED FOODS 759 J. Ralph Blanchfield 38 FAO/WHO FOOD STANDARDS PROGRAM: CODEX ALIMENTARIUS 793 Eduardo R. Mendez and John R. Lupien Index 801 PREFACE Food safety legislation and regulations have long been impacted by a variety of factors, including socioeconomic, consumer, political, and legal issues. With regard to food safety issues and concerns, certain parallels can be drawn between the beginning and close of the 20th century. At the start of the 20th century, several food safety issues were brought to the public’s attention. Atrocious sanitation problems in the meat industry, highlighted in Upton Sin- clair’s novel The Jungle, had a major influence on the passage of the landmark legislation, the Federal Meat Inspection Act (1906). Likewise, fairly wide-spread food adulteration with the addition of inappropriate chemical substances, and the marketing of a variety of fraudulent and potentially dangerous elixirs, con- coctions, and other formulations, led to passage of the Pure Food and Drug Act (1906). We are now in the 21st century and, food safety issues have as high a prior- ity and significance as they did over 100 years ago.” Public concerns have arisen regarding high-profile food-borne illness outbreaks due to contamination of food with certain pathogens (e.g., Salmonellu, Escherichiu coli 0 1 57:H7, Listeriu monocytogmes, and others) which have serious acute impact and potential chronic long-term complications in the ever-increasing high-risk population segment (e.g., elderly, children, immuno-compromised). In addition, food-borne illness outbreaks are occurring in foods previously not considered high risk (e.g., fruit juices, fresh produce, deli meats). In response to these food- borne pathogen issues, a presidential budgetary initiative was instituted in 1997 to put a multi-agency food safety strategy in place. This National Food Safety Initiative includes a nationwide early warning system for food-borne illness, expanded food safety research, risk assessment, training and education pro- xi Xii PREFACE grams, and enhanced food establishment inspection systems. Pathogen issues have also resulted in endorsement and implementation of comprehensive pre- vention and intervention strategies, such as the Hazard Analysis Critical Con- trol Point (HACCP) system, by the regulatory and industrial communities. Another parallel can be drawn to earlier times. Society today, like that of the early 19OOs, is strongly interested in attaining certain therapeutic and health benefits through special foods (e.g., nutraceuticals and functional foods), and, once again, the line between foods and pharmaceuticals has become blurred. The trend to market these products has created certain labeling concerns with regard to health claims, as well as safety and efficacy concerns. As the world has gotten smaller through increased communication, travel, immigration, and trade, there are current concerns regarding the safety of food products throughout the world. Global consumer concerns regarding geneti- cally modified foods and ingredients, as well as potential chemical residues in foods, have had a major impact on current and future legislation, as well as world trade. The intent of this book is to define and categorize the real and perceived safety issues surrounding food, to provide scientifically non-biased perspectives on these issues, and to provide assistance to the reader in understanding these issues. While the primary professional audience for the book includes food technologists and scientists in the industry and regulatory sector, the book should provide useful information for many other audiences. Part 1 focuses on general descriptions of potential food safety hazards and provides in-depth background into risk assessment and epidemiology. Potential food hazards are characterized in Part 11, where biological hazards are dis- cussed, and in Part Ill, which addresses chemical and physical hazards. Control systems and intervention strategies for reducing risk or preventing food hazards are presented in Part IV, V and VI. The emphasis of Part IV is on regulatory surveillance and industry programs including Hazard Analysis Crit- ical Control Point (HACCP) systems. Food safety intervention in food pro- cessing, handling and distribution are addressed in Part V, while the focus of Part Vl is on the retail foods sector. Diet, health and safety issues are charac- terized in Part VTI, with emphasis on food fortification, dietary supplements, and functional foods. Finally, Part VIII addresses world-wide food safety issues through discus- sion of Codex Alimentarius Cotiztnission ( C A C ) , the European Union per- spectives on genetic modification, and other globally accepted food standards. The topics within each chapter are divided into sections called units. To provide continuity across the book, these units have been generally organized according to the following structure: Introduction and Definition of Issues. Background and Historical Sigiil'fcance, ScientGc Basis and Iiizplic~rtions, Regulatory, Industrial, and International Iniplications, and Current and Future Iniplica tions. This project was a highly ambitious project and the co-editors would like to acknowledge the many people who provided valuable input and assistance and PREFACE Xiii to express our sincere appreciation for their efforts. This appreciation is espe- cially extended to G. William Chase, LeeAnne Jackson, Austin R. Long, Joan Rose, Mary K. Schmidl, Keith R. Schneider, Barry G. Swanson, and Sara E. Valdes Martinez, for their enthusiasm and diligence in serving as Part Editors and to all of the numerous authors of the Chapters. We would also like to ex- tend a sincere thank you to Virginia Chanda, Michael Penn, and all the staff at John Wiley and Sons, Inc. who provided invaluable assistance to the project. RONALDH. SCHMIDTand GARYE. RODRICK PART 1 CHARACTERIZATION OF FOOD SAFETY AND RISKS Edited by JOAN ROSE Food Safety Hrmdhook, Edited by Ronald H. Schmidt and Gary E. Rodrick 0-471-21064-1 Copyright 0 2003 John Wiley & Sons, Inc. CHAPTER 1 DEFINITION OF FOOD SAFETY ROBERT (SKIP) A. SEWARD I1 INTRODUCTION AND DEFINITION OF ISSUES The term “safe food” represents different ideals to different audiences. Con- sumers, special interest groups, regulators, industry, and academia will have their unique descriptions based on their perspectives. Much of the information the general public receives about food safety comes through the media. For this reason, media perspectives on the safety of the food supply can influence those of the general public. Consumers are the end users and thus are at the last link of the food supply chain from production, through processing and distribution, to retail and food service businesses. Consumers are multidimensional and multifaceted. Pop- ulations differ in age, life experiences, health, knowledge, culture, sex, political views, nutritional needs, purchasing power, media inputs, family status, occu- pation, and education. The effect of the interrelationships of these factors on an individual’s description of “safe food” has not been established. When educated consumers were asked by the author to define safe food, their descriptions included some key elements. Safe food means food that has been handled properly, including thorough washing of fish and poultry that will be cooked and anything to be eaten raw. Safe food means food prepared on clean and sanitized surfaces with utensils and dishes that also are cleaned and sanitized. These consumers mention the importance of hand washing by those involved in food preparation and the importance of not reusing cloths or sponges that become soiled. Common sense is a guiding principle for the edu- cated, informed consumer. Other consumers want safe food that retains vitamins and minerals but does not have harmful pesticides. They describe safe food as food that is within its shelf life and has been stored and distributed under proper temperature control. Some consumers know the word “contamination” and will define safe food as food that is not contaminated. Food Safety Hrmdhook, Edited by Ronald H. Schmidt and Gary E. Rodrick 0-471-21064-1 Copyright 0 2003 John Wiley & Sons, Inc. 3 4 DEFINITION OF FOOD SAFETY For other consumers, the descriptions of safe food are more practical, like food that does not make a person ill. For these consumers, safe food means purchasing fresh chicken and not having the package leak or drip juice, making them wonder about the integrity of the initial seal. Consumers use their senses in their descriptions of safe food, and they feel that food that looks or smells bad should not be eaten. Surprisingly, not many consumers refer to labeling as a key component of safe food. Consumers believe they know what to do with food after it is purchased, and they assume that the safety of the food is primarily determined before it reaches their hands. Published data suggest otherwise. McDowell (1998) reported the results of on-site inspections of 106 house- holds in 81 U.S. cities by professional auditors. A college degree was held by 73% of the participants. Inspection of meal preparation, cleanup, temperatures, sanitation, the environment, and personal hygiene resulted in at least one critical violation being cited in 96%)of households. The most common critical violations were cross-contamination (76'%, of households with this violation), neglected hand washing (57'!h), improper leftover cooling (29'%1),improper chemical storage (28%), insufficient cooking (240/;1),and refrigeration above 45°F (23%). Similarly, Jay et al. (1999) used video recording to study food handling practices in 40 home kitchens in Melbourne, Australia. Households of various types were video monitored for up to two weeks during 1997 and 1998. There was a significant variance between what people said they would do and what they actually practiced with respect to food safety in the home. The most com- mon unhygienic practices included infrequent and inadequate hand washing, inadequate cleaning of food contact surfaces, presence of pets in the kitchen, and cross-contamination between dirty and clean surfaces and food. A national telephone survey was done by Altekruse et al. (1995) to estimate U.S. consumer knowledge about food safety. The 1,620 participants were at least 18 years old and had kitchens in their homes. One-third of those surveyed admitted to using unsafe food hygiene practices, such as not washing hands or preventing cross-contamination. There was a disparity between the level of knowledge and corresponding safe hygiene practices. This suggested that deci- sions to practice safe food handling likely are based on various factors includ- ing knowledge, risk tolerance, and experience. Jay et al. (1999) conducted a telephone survey of 1,203 Australian house- holds and found significant gaps in food safety knowledge. The most important were incorrect thawing of frozen food, poor cooling of cooked food, under- cooking of hazardous food, lack of knowledge about safe refrigeration tem- peratures and cross-contamination, and lack of knowledge about frequency and techniques of hand washing. The authors found the participants receptive to educational information regarding the preparation of safe food. Knowledge and compliance regarding the preparation of safe food increased with the age of the participants. BACKGROUND AND HISTORICAL SIGNIFICANCE 5 Special interest groups represent a focused view on safe food. These groups study the issues that they believe are most relevant to food safety and then express their concerns to consumers, regulatory authorities, industry, and aca- demia. They typically define safe food by more specific limits for hazards than those used in the food supply chain. The special interest groups define safe foods through more stringent control limits for microbial pathogens and chemical hazards. They seek a higher level of food safety through requirements for more interventions to control hazards and elimination of chemicals used in food production, over fears of adverse health effects. Special interest groups often question the approvals by governmental agencies of practices designed to increase the productivity and efficiency asso- ciated with agriculture and animal husbandry, for example, the use of anti- biotics and hormones. Furthermore, the definition of safe food by selected spe- cial interest groups would exclude foods made through enhanced technology, such as genetic engineering. Again, they would view with suspicion, the science that established the safety of these new foods for the regulatory authorities responsible for their approval. Special interest groups such as the U.S.-based Center for Science in the Public Interest (CSPI) do provide guidance for consumers and recommenda- tions for government. CSPI and the Safe Food Coalition have outlined their recipe for safe food by calling for funding for the U.S. National Food Safety Initiative proposed in 1997, more authority for the U.S. Department of Agri- culture (USDA) to enforce food safety laws, more power for the U.S. Food and Drug Administration (FDA) to keep contaminated products off the mar- ket, and a single agency responsible for food safety. The CSPI has noted that consumers need to understand the broader range of products involved as vehicles of foodborne illnesses. The CSPI has stated that, although the effort is underfunded and not well-coordinated, government has improved the safety of the nation’s food supply through legislation and regulation. BACKGROUND AND HISTORICAL SIGNIFICANCE Over his distinguished career, E.M. Foster has provided a unique perspective on the history of safe food (Foster, 1997). He has described how, for many, food production and consumption were tied to daily life on a farm. Through experience, time control became the means by which safe food was ensured, because for many people refrigeration was not available. According to Foster, examples of botulism, salmonellosis, and Clostvidium perfringens food poison- ing from new food vehicles have shown how our perceptions and understand- ing of safe food change with new knowledge about the capacities of microbial pathogens to adapt and proliferate in selected environments. 6 DEFINITION OF FOOD SAFETY SCIENTIFIC BASIS AND IMPLICATIONS Because academicians are some of the most educated consumers, they generally have the greatest understanding regarding the safety of foods, balancing the science with the practical application of the science in the food supply chain. Academicians can be the most knowledgeable about the science-based research used in defining safe food. However, the specifics of research, and the innu- merable questions that are generated through research, lead to inevitably vari- able viewpoints on the science. The academic questions surrounding safe food are often multidimensional, involving scientific disciplines including biochem- istry. microbiology, genetics, medicine, plant and animal physiology, and food science, to name only a few. Because academicians generally are narrowly focused in particular research disciplines, their definitions include details sur- rounded by boundaries and assumptions. One of the common scientific measures used to define safe food is the num- ber of illnesses associated with food. In the U.S., data sources for this measure include the Foodborne Diseases Active Surveillance Network ( FoodNet), the National Notifiable Disease Surveillance System, the Public Health Laboratory Information System, the Foodborne Disease Outbreak Surveillance System, and the Gulf Coast States Vibvio Surveillance System. Similar surveillance sys- tems are in use in other countries to gather foodborne disease statistics. Mead et al. ( 1 999) used these data sources, and others, to estimate that foodborne diseases cause -76 million illnesses and 5,000 deaths in the U.S. annually. Viruses. predominantly Norwalk-like viruses, accounted for nearly 80% of the estimated total cases caused by known foodborne pathogens. REGULATORY, INDUSTRIAL, AND INTERNATIONAL IMPLICATIONS Regulatory authorities are also consumers and thus carry many of the biases and perceptions held by consumers in general. However, regulatory authorities typically have a higher level of training in food safety. They differ in the scope of their responsibilities and influence, working at local, state, federal, or global levels. They also differ in their experiences with food along the food chain, from farming and animal production through manufacturing, distribution, and testing, to retail and food service. These experiences will affect their definitions of safe food. Regulatory authorities that oversee food production are more aware of the impact of agricultural chemicals, animal hormones, feed contaminants, and antibiotics and would include details of these factors in their description of safe food. In processing environments, regulators would be more apt to describe safe food in terms of the microbiological, chemical, and physical hazards asso- ciated with manufacturing. Regulatory authorities overseeing retail and food REGULATORY, INDUSTRIAL, AND INTERNATIONAL IMPLICATIONS 7 service would include the human factors such as cross-contamination by food handlers and personal hygiene behaviors. Regulatory authorities also describe safe food according to regulations established by authorities such as the World Health Organization (WHO), the European Commission, and the U.S. FDA. The standards and laws set for international trade become part of the regulatory definitions of safe food. For example, the food safety standards adopted by the Joint Food Agricultural Organization/WHO Codex Alimentarius Commission (CAC) have become the international reference used to resolve international trade issues. Some reg- ulatory authorities are using quantitative risk assessment to help define food safety, as well as to determine optimal intervention strategies. Scientific risk assessments have reportedly become the foundation for food safety worldwide with the issuance of the Sanitary and Phytosanitary Agreement by the World Trade Organization (WTO) (Smith et al., 1999). Government officials often speak of safe food in terms designed to appeal to public emotions about food safety. For example, on July 2, 1998, the U.S. Vice President challenged the U.S. Congress to fund a Food Safety Initiative and “give Americans peace of mind when they reach for a piece of food.” The Vice President stated the need for “new authority to seize meat that may be con- taminated, to protect America’s families.” However, experts know that more recall authority does not improve food safety. The U.S. Food Safety Initiative is broad in its vision and scope. A key component of the Initiative is educating consumers on the responsibilities for food safety of everyone involved in the food supply chain. The industry sector is broad in its constituency. Farmers and ranchers are the basis on which most of the food supply chain exists. At this level, food safety is defined by the practices of the farmers and ranchers, whether in regard to chemical treatment of the soil or use of hormones in animal produc- tion. These plant and animal producers define safe food based on the practical application of production principles, balancing economic pressures of produc- tion with demands for control of hazards. Safe food at this level means doing what is practical to ensure safety and focusing on optimal use of government- approved chemicals to maximize production. Thus far, there has not been a significant focus on controlling microbiological hazards at this level of the food chain; however, there is increasing recognition of the role of farmers and ranchers in defining safe food through their practices. The food industry defines safe food by its specifications for raw materials and finished products. These specifications define the acceptable limits for chemical hazards such as pesticides and hormones, physical hazards such as bone and metal fragments, and microbiological hazards such as Listeviu mono- cytogenes and Sdmonella. The industry defines safe food in terms of pathogen reduction associated with processing technologies, whether well-established like pasteurization or new like pulsed, high-energy light. The industrial sector also includes distribution, retail, and restaurant busi- 8 DEFINITION OF FOOD SAFETY nesses, as well as related industries supporting the growth of plants and animals and the use of by-products for nonfood applications, such as for health care and clothing. Distributors, retailers, and restaurants define safe food by the expectations of their customers and the regulatory authorities. CURRENT AND FUTURE IMPLICATIONS Safe food is a composite of all of the views and descriptions held by consumers, special interest groups, academicians, regulatory authorities, and industry. Almost any single definition of safe food will be overly simplistic, because safe food is a complex, multifaceted concept. The scientific experts attending the 1998 American Academy of Microbiology Colloquium on Food Safety (AAM, 1999) described safe food as follows: Safe food, if properly handled at all steps of production through consumption, is reliably unlikely (i.e., the probability is low and the variability is small) to cause illness or injury. Everyone wants a safe food supply. The criteria by which food is defined as safe will become more detailed and comprehensive as new steps are taken to improve safety. As capabilities rise, so will the expectations. The difficult decisions are those relating to perceived risks that drive the unnecessary use of public and private resources. If a food is perceived or reported to be unsafe, the story can be amplified in the press and then validated in the public mind by the involvement of politicians and regulators. All this can happen in the absence of scientific data that truly defines the risk (Smith et al., 1999). Consumers have a role to play in ensuring that food is safe. They need to make informed choices about their food and how it is handled and prepared. According to Lopez (1999), consumer education about food safety must take place. Without a widely accepted definition of safe food, the public will have unrealistic misconceptions about the degree of safety that is attainable. Lopez pointed out that food safety standards have economic as well as scientific dimensions and that consumers are not likely to pay the high costs of abso- lutely safe food. To this end, industry and government have responsibility for improving safety as well as for educating consumers on the practical aspects of safe food. Research is needed to determine what impacts consumers' food safety practices (AAM, 1999). The application of Sulmonellu and Eschevichiu coli performance standards for the U.S. food supply exemplifies a trend by regulators toward using micro- bial counts and prevalence data to define safe food. Yet there is general agree- ment among experts in food safety that food sampling and testing is not the sole means of ensuring safe food. The statistics of routine sampling indicate the limits of testing to define safe food. For example, E. coli 0157:H7 in ground beef and Listeviu monocytogenes in cooked foods are present at low levels, typically below 0.1"/0. Even when testing 60 samples per lot, there is a greater than 90% chance of not detecting the pathogen. Companies normally test fewer samples (3-5 per lot) to confirm that their Hazard Analysis and Critical Con- LITERATURE CITED 9 trol Point (HACCP) system is functioning; thus the likelihood that testing will establish the safety of the food is greatly limited. Furthermore, pathogens will not be homogeneously distributed in many contaminated foods, which may also reduce the value of sampling and testing to determine safety. Global differences in judgments on safe food are likely to continue, such as the current disagreements over the safety of beef hormone treatments and genetically modified foods between the U.S. and the European Union. These differences exist despite mechanisms such as the dispute resolution system of the WTO. In general, the European view of safe food is fundamentally different from that in the U.S., with culture and history as important as science in some decision-making processes. LITERATURE CITED American Academy of Microbiology (AAM). 1999. Food Safety: Current Status and Future Needs. AAM, Washington, D.C. Altekruse, S.F., Street, D.A., Fein, S.B., and Levy, A S. 1995. Consumer knowledge of foodborne microbial hazards and food-handling practices. J. Food Prot. 59:287-294. Foster, E.M. 1991. Historical overview of key issues in food safety. Emerg. Infect. Dis. 3:48 1-482. Jay, S.L., Comar, D., and Govenlock, L.D. 1999. A national Australian food safety telephone survey. J. Food Prot. 62:921-928. Lopez, R. 1999. AAM identifies key microbiology-related food safety issues. A S M News 65:147-151. McDowell, B. 1998. Failing grade. C h i n Leader 3:28. Mead, P.S., Slutsker, L., Dietz, V., McCaig, L.F., Bresee, J.S., Shapiro, C., Griffin, P.M., and Tauxe, R.V. 1999. Food-related illness and death in the United States. Emerg. Infect. Dis. 5:601-625. Smith, M., Imfeld, M., Dayan, A.D., and Roberfroid, M.B. 1999. The risks of risk assessment in foods. Food Clwm. Toxirol. 37:183- 189. CHAPTER 2 CHARACTERIZATION OF FOOD HAZARDS ROBERT (SKIP) A. SEWARD I1 INTRODUCTION AND DEFINITION OF ISSUES Hazard characterization with respect to foods began as a means to help pri- oritize risks and categorize hazards. Over time, hazard characterization has broadened in scope, as the criteria used to evaluate hazards have increased in number and breadth. Today, characterization of hazards is more important than ever in developing food safety control programs. The use of categoriza- tion is of lesser importance as susceptibility of the population to the hazards becomes greater. The WHO (1995) described hazard characterization as the qualitative and quantitative evaluation of the nature of the adverse effects associated with biological, chemical, and physical agents that may be present in foods. Van Schothorst (1998) suggested that hazard characterization might be bet- ter termed “impact characterization.” The impact can vary from mild (simple acute diarrhea) to severe (chronic illness or death), depending largely on the susceptibility of the person exposed. To accommodate the many assumptions associated with impact characterizations, a worst-case scenario often is used to estimate the risk presented by a particular pathogen in a specific food. Van Schothorst points out that assumptions and uncertainties of hazard character- ization ultimately can lead to an unreliable risk assessment, as well as credibil- ity and liability problems. The National Advisory Committee on Microbiological Criteria for Foods (NACMCF) (1997) defined a hazard as a “biological, chemical, or physical agent that is reasonably likely to cause illness or injury in the absence of its control.” Microbial pathogens are the most common biological Iiazards, and they can cause infections (growth of the disease-causing microorganism) and intoxications (illness caused by preformed toxin produced by a micro- Food Safktj Hantlhooli, Edited by Ronald H. Schmidt and Gary E. Rodrick 0-471-21064-1 Copyright ’@? 2003 John Wilcy & Sons. Inc. 11 12 CHARACTERIZATION OF FOOD HAZARDS organism). Scott (1 999) has detailed the characteristics of numerous common microbial hazards and described the factors that affect the risk of illness from the hazards. Chemical hazards include agricultural compounds such as pesticides, anti- biotics, and growth hormones; industrial chemicals such as cleaners and sani- tizers; and equipment-related compounds such as oils, gasoline, and lubricants. Other chemical hazards include naturally occurring toxicants such as myco- toxins, environmental contaminants such as lead and mercury, and chemical preservatives and allergens. Physical hazards include glass, wood, plastic, stones, metal, and bones. The introduction of physical hazards has been characterized as inadvertent con- tamination from growing, harvesting, processing, and handling; intentional sabotage or tampering: and chance contamination during distribution and storage (Corlett, 1998). BACKGROUND AND HISTORICAL SIGNIFICANCE The language surrounding the term “hazard characterization” has referred to the food products themselves, as well as to the hazards that might be present in the food. Hazard characterization has been used in the development of Hazard Analysis and Critical Control Point (HACCP) plans and regulatory policies, as well as for risk assessments. In 1969, the National Academy of Sciences issued a report evaluating the Sulnionellu problem (NAS, 1969). This report described three hazard characteristics associated with food and Sublzonellu: 1. Products containing ingredients identified as significant potential factors in salmonellosis, 2. Manufacturing processes that do not include a control step that would destroy Scdwwnellae, and 3. Substantial likelihood of microbiological growth if mishandled or abused in distribution or consumer usage. With the various combinations of these three hazard characteristics, five categories were created that reflected the potential risk to the consumer. Cate- gory I included food products intended for use by infants, the aged, and the infirm, that is, the restricted population of high risk. Category I1 included pro- cessed foods that were subject to all three hazard characteristics (ABC) listed above. Category 111 included those products subject to two of the three general hazard characteristics. These would include such products as custard-filled bakery goods (AC), cake mixes and chocolate candy (AB), and sauce mixes that do not contain a sensitive ingredient (BC). Category IV included products of relatively minor microbiological health hazard level, subject to only one of the hazard Characteristics. Examples include retail baked cakes (A) and some frosting mixes (B). Category V includes foods that are subject to none of the BACKGROUND AND HISTORICAL SIGNIFICANCE 13 microbiological hazard characteristics and therefore of minimal hazard poten- tial, for example, canned foods sterilized after packaging in the final container. The Pillsbury Company is recognized as the first company to have devel- oped HACCP plans. The Pillsbury approach to HACCP systems also used three hazard characteristics to categorize food products. In this instance, the hazard characteristics were generalized to include all potential microbial, physical, and chemical hazards, not only Sulmonellu (Sperber, 1991). As in the NAS report, the permutations of the hazard characteristics resulted in five product hazard classes. The use of the three hazard characteristics to assess risks was standard in the 1970s (Bauman, 1974). In 1989, the NACMCF presented a HACCP document that used six hazard characteristics to rank microbial hazards for risk assess- ments (NACMCF, 1989). Chemical and physical hazards were included sub- sequently (Corlett and Stier, 1991). Hazard characterization at this time was made on the basis of criteria such as: The consumers’ risks associated with factors such as age and health, The risk associated with the ingredients used to make the food product, The production process and its impact on the hazard, The likelihood of recontamination after processing, The potential for abuse during distribution and consumer handling, and The ability of the consumer to detect, remove, or destroy the hazard during the final preparatory steps. The hazard classification scheme (Hazard Categories A-F) described in the 1989 NACMCF document was updated in 1992 (NACMCF, 1992) and again in 1997 (NACMCF, 1998a). These revisions aligned U.S. HACCP concepts with those published by the internationally recognized Codex Alimentarius Commission (CAC) (1997). The most recent HACCP documents characterize hazards as part of the hazard analysis. The hazard characterization, or evalua- tion, is done after the hazards have been identified. The criteria for character- izing the hazard include: The severity of the hazard, to include the seriousness of the consequences of exposure, or the magnitude and duration of the illness or injury, The likelihood that the hazard will occur, based on published information and epidemiological data, The potential for both short-term and long-term effects from exposure, and Available risk assessment data, as well as many of the criteria stated in earlier documents. Ultimately, according to William H. Sperber (personal communication), “the hazard characteristics were discarded in favor of an open-ended hazard analysis in which an unlimited number of relevant questions could be asked 14 CHARACTERIZATION OF FOOD HAZARDS about the product and the process by which it is produced. The product hazard categories fell into disfavor as we recognized that a relatively large percentage of consumers are immunocompromised. All foods must be safe for all con- sumers. The emergence of new foodborne pathogens in relatively narrow niches, e.g., Listeria inonocytogenes in some perishable ready-to-eat foods, fur- ther rendered the concept of product hazards categories moot.” SCIENTIFIC BASIS AND IMPLICATIONS I n addition to its role in the development of HACCP plans, hazard character- ization has been identified as the second step of the risk assessment process (Smith et al., 1999). The characterization includes determination of risk factors, defining the site and mechanism of action, and measuring the dose-response relationship (proportion responding or severity of response). Despite large uncer- tainties, dose-response models are commonly used to predict human health effects and even to establish regulatory policies. According to the WHO (1995), a dose-response assessment should be per- formed for chemical hazards. For biological or physical agents, a dose-response assessment should be performed if the data are obtainable. Although poten- tially hazardous chemicals may be present in foods at low levels, for example, parts per million or less, animal toxicological studies typically are done at higher levels to obtain a measurable effect. The significance of the adverse effects associated with high-dose animal studies for low-dose human exposure is a major topic of debate with regard to the hazard characterization of chem- icals. The extrapolation of animal exposure data to human exposure levels is uncertain both qualitatively and quantitatively. The nature of the hazard may change with dose. Not only is the equivalent dose estimate in animals and humans problematic in comparative pharmacokinetics, the metabolism of the chemical may change as the dose changes. Whereas high doses can overwhelm detoxification pathways, the effects may be unrelated to those seen at low doses (WHO, 1995). A primary contributor to the uncertainty of the hazard characterization is the intraspecies variance in the dose response at different dosage levels. Large exposures often are used to increase the power of a study yet may be inaccu- rate for low-dose exposure. Variance also results from many other differences among individual animals and humans. Toxicologists often use thresholds to quantify adverse effects from chemical exposures, except in the case of carcinogenic effects, where initiating events can occur as persistent somatic mutations that later develop into cancer. Some carcinogens may be regulated with a threshold approach, such as the “No Observed Effect Level (NOEL)-safety factor” approach. A safe level of a chem- ical often is derived from an experimental NOEL or No Observed Adverse Effect Level (NOAEL) by using safety factors. A safety factor of 100 has been applied when using data from long-term animal studies, but it may be adjusted CURRENT AND FUTURE IMPLICATIONS 15 if data are insufficient or if the effect is more severe or irreversible. It has been suggested that conservative models and large safety factors should be used for food systems potentially contaminated with biological hazards because of the unpredictability of these systems (Smith et al., 1999). Obviously, the safety factor approach is full of uncertainty and cannot guarantee absolute safety for everyone. For carcinogens that cause genetic alterations in target cells, the NOEL safety factor approach is usually not used because of the assumption that risk exists at all doses, even the lowest. Risk management options are to ban the chemical or establish a negligible, insignificant, or socially acceptable level of risk with quantitative risk assessment. An alternative approach has been to use a lower effective dose, or a benchmark dose, which depends more on data near the observed dose-response range. This may allow more accurate predictions of low-dose risks. Characterization of biological hazards is done to provide a qualitative or quantitative estimate of the severity and duration of adverse effects due to the presence of a pathogen in a food. Dose-response data are useful but scarce for microbial pathogens. Furthermore, inaccuracies in the data may occur for the following reasons: host susceptibility to pathogenic bacteria is variable; attack rates from specific pathogens vary; virulence of a pathogen is variable; patho- genicity is subject to genetic mutation; antagonism from other microbes may affect pathogenicity; and foods will modulate microbial-host interactions. REGULATORY, INDUSTRIAL, AND INTERNATIONAL IMPLICATIONS As pointed out by Kaferstein et al. (1997), the globalization of trade requires coordination among international regulatory and health protection authorities. Food safety standards, recognized by the WTO, place greater dependence and emphasis on scientific risk assessments. Hazard characterization will remain a key component of the risk assessment (NACMCF, 199%). The International Commission on Microbiological Specifications for Foods (ICMSF) has proposed the use of the Food Safety Objective (FSO) as a man- agement tool to control the risk of foodborne illness. The FSO reflects the fre- quency or maximum concentration of a microbiological hazard in a food that is considered acceptable for consumer protection. FSOs are broader in scope than microbiological criteria. FSOs link risk assessment and risk management processes and establish control measures (ICMSF, 1998). The hazard charac- terization process will contribute information toward establishing the FSO. CURRENT AND FUTURE IMPLICATIONS The Institute of Food Technologists (IFT), a scientific society for food science and technology with over 28,000 members, has stated that food safety policies must be based on risk assessment. IFT agreed with the WHO (1995) that 16 CHARACTERIZATION OF FOOD HAZARDS improvements in risk assessment require more precise characterization of hazards and measures of exposure. Better data on exposure to pathogens, the behavior of pathogens in foods, and dose-response relationships for population subgroups are essential (IFT, 1997). Scientific experts attending the AAM Colloquium on Food Safety (1999) identified future research needs including a cross-discipline definition of dose-response relations and better characteriza- tion of hazards causing chronic disease syndromes such as reactive arthritis and ulcers. As new scientific data are developed, the hazard characterization pro- cess will continue to be redefined and improved. The acceptable limits for haz- ards will change, as will the range of hazards included in a given food safety control program. Harmonization of the hazard characterization approaches will help global trade by facilitating a common basis for setting product standards and defining safe food. The initial steps have been taken with the SPS Agreement and FAO/ WHO CAC standards and guidelines. Hazard characterization, although cru- cial to the development of food safety control programs, will not define safe food by itself. The definition of safe food will improve as we understand how better to integrate hazard characterization, population preferences, cultural biases, and many other considerations into judgments on safe food. LITERATURE CITED American Academy of Microbiology (AAM). 1999. Food Safety: Current Status and Future Needs. AAM, Washington, DC. Bauman, H.E. 1974. The HACCP concept and microbiological hazard categories. Food Technol. 2830. Codex Alimentarius Commission (CAC). 1997. Joint FAO/WHO Food Standards Pro- gramme, Codex Committee on Food Hygiene. Vol. 1B-1997 suppl. Hazard Analysis and Critical Control Point (HACCP) System and Guidelines for Its Application. Annex to CAC/RCP 1-1969, Rev. 3. Corlett. D.A. 1998. HACCP User’s Manual. Aspen Publishers, Inc., Frederick, MD. Corlett, D.A. and Stier, R.F. 1991. Risk assessment within the HACCP system. Food Control 2:71-72. International Commission on Microbiological Specifications for Foods (ICMSF). 1998. Principles for the establishment of microbiological food safety objectives and related control measures. Food Control 9:379-384. Institute of Food Technologists (IFT). 1997. Comment on the “President’s National Food Safety Initiative” discussion draft. IFT Statements and Testimonies, comment (G-068), March 27. Jay, S.L., Comar, D., and Govenlock, L.D. 1999. A video study of Australian domestic food-handling practices. J. Food Prot. 62: 1285-1296. Kaferstein, F.K., Motarjemi, Y., and Bettcher, D.W. 1997. Foodborne disease control: A transnational challenge. Emerg. Infect. Dis. 3503-510. INTERNET RESOURCES 17 National Advisory Committee on Microbiological Criteria for Foods (NACMCF). 1989. HACCP principles for food protection. In HACCP: Principles and Applica- tions (X.X. Pierson and X.X. Corlett, eds.). Chapman & Hall, New York. NACMCF. 1992. Hazard analysis and critical control point system. Int. J. Food Microbiol. 16:1-23. NACMCF. 1998a. Hazard analysis and critical control point principles and application guidelines. J. Food Prot. 61:1246-1259. NACMCF. 1998b. Principles of risk assessment for illness caused by foodborne biolog- ical agents. J. FoorlProt. 61:1071-1074. National Academy of Sciences (NAS). 1969. An evaluation of the Sulvnonellu problem. Committee on Sulrmnella, Division of Biology and Agriculture, National Research Council, National Academy of Sciences. Washington, DC. Scott, V.N. 1999. Biological hazards and controls. In HACCP: A Systematic Approach to Food Safety, 3rd ed. (K.E. Stevenson and D.T. Bernard, eds.). The Food Pro- cessors Institute, Washington, D.C. In press. Smith, M., Imfeld, M., Dayan, A.D., and Roberfroid, M.B. 1999. The risks of risk assessment in foods. Food Chmm Toxicol. 37: 183-1 89. Sperber, W.H. 1991. The modern HACCP system. Food Terlznol. 45: 116-1 18. Tompkin, R.B. and Bodnaruk, P.W. 1999. A proposed food safety management plan for E. coli 0157:H7 in ground beef. It? The Role of Microbiological Testing in Beef Food Safety Programs (C. Calkins and M. Koohmaraie, eds.). American Meat Science Association, Kansas City, KS. Van Schothorst, M. 1998. Risks of microbial risk assessment. Presented at the ILSI (International Life Sciences Institute) Europe 1998 General Assembly Workshop: The Risks of Risk Assessment in Foods, 18 Feb., Brussels, Belgium. World Health Organization (WHO). 1995. Application of Risk Analysis to Food Standards Issues, A Report of the March 13-17 Joint Food and Agriculture OrganizationIWorld Health Orgmization Consultation, World Health Organization, Geneva, Switzerland. INTERNET RESOURCES iviiiiv. cspinrt. oug Web site for Center for Science in the Public Interest that demonstrates the definition of safe food by special interest groups. iwiv.,fuo. org/wairentlfiroinjolmronomiclesnlcoctmx-ld~juiilt. htrw Web site for Codex, summary reports and Joint FAO/WHO Food Standards Pro- gramme. go 1’ ~l~bVIl~.,fdU. Web site for the U.S. Food and Drug Administration that provides information on the role of government in defining a safe food supply. l l ~ l l ~ lfooClscIjdy. t~., go11 Web site that is the gateway to U.S. government food safety information, including the National Food Safety Initiative. 18 CHARACTERIZATION OF FOOD HAZARDS Ltww. ishitehouse.gov Web site providing governmental viewpoint on safe food and the initiatives necessary to achieve food safety. i s i w. who. int~jsf;lllihri.rkassess/crpplie~~~~~i~i~l~~. Iitm Web site for Report of Joint FAO/WHO Expert Consultation on the Application of Risk Analysis to Food Standard Issues. CHAPTER 3 RISK ANALYSIS FRAMEWORKS FOR CHEMICAL AND MICROBIAL HAZARDS MARGARET E. COLEMAN and HARRY M. MARKS INTRODUCTION AND DEFINITION OF ISSUES Human individuals and societies have been identifying risks and managing them since ancient times (When you build a new house, you shall make a par- apet for your roof, so that you shall not put blood in your house if [when] one falls from it. Deut. 22:8) by various procedures, including establishing codes of practice and formal laws. What appears to be a more modern concern is the quantitative nature of risk, that is, the precise calculation of probabilities of risk. Performing these calculations is complex and involves input from many areas of the society. In particular, hazards and the risks associated with them need to be identified, and society must value the knowledge that calculation of the risks provides. Tt is not surprising that in a well-informed, free society, risk analysis has become a serious and growing field. The complexity of the calcu- lations and the political will to make the calculations have created the need to structure the process of risk analysis so that the calculations are performed in an efficient and understandable manner. This chapter is a short discussion of the managerial frameworks that have been adopted in risk analysis and some of the issues surrounding them. A natural beginning point in a discussion of risk analysis is the definition of risk. Even among practitioners of risk analysis, developing a standard defini- tion of risk has been problematic. A committee of professionals in the newly formed Society for Risk Analysis (SRA) convened in the early 1980s and was unable to reach a single, consensus definition for risk after 4 years of deliber- ations (Kaplan, 1997). The recoinmendation of the SRA at that time was that freedom be permitted for professionals to define risk in a manner best suiting the particular discipline or problem at hand. Food Sirl;.tj>Htrizdbooh-, Editcd by Ronald H. Schmidt and Gary E. Rodrick 0-471-21064-1 Copyright 2003 J o h n Wiley & Sons, Inc. t? 19 20 RISK ANALYSIS FRAMEWORKS FOR CHEMICAL AND MICROBIAL HAZARDS A good operational definition of risk is important because from it one can determine how to structure the activities needed to perform the calculations of risk and to disseminate the meaning of the calculations. A general operational definition provided by Kaplan (1 98 1, 1997) will serve, with some modification, as a starting point for our discussions of risk analysis frameworks. Risk was defined (Kaplan, 1981, 1997) with responses to three basic questions. 1) What can happen? 2) How likely is it? 3) What are the consequences? In the Kaplan papers, which use an engineering perspective, the first component, “What can happen?” seems to describe an event such as a fire. Kaplan terms this first component as a scenario, S, and defines So as the successful scenario of nothing happening or nothing going wrong. For risk analysis of foodborne illness, the causal event is always the ingestion of something that is hazardous. Thus, for risk in food, the only answer to the question “What can happen‘?’’is a single event, ingestion of a hazardous chemical or pathogen. Consequently, for our purposes, we shall adapt the concept of “scenario” to mean an event or a pro- cess that can produce the specified potential hazard and consequence for a given population. Thus, for food risk analysis, the scenario represents a c m u ’ i - tiom/ event or process, for which the associated risk must be evaluated. For example, a scenario might be the production of a certain food for which the presence of a certain hazard cannot be excluded. This hazard could result in fetal complications for pregnant women consuming the food. Three aspects of a scenario must be defined for a risk assessment: 1) the process, 2) a potential consequence, and 3) the target population. The “How likely is it?” question represents the conditional likelihood for the given scenario that the hazard- ous agent will be ingested, for example, the number of times that a pregnant woman would consume a serving of the food. The “What are the conse- quences?” question represents the probability of the adverse consequence, given the ingestion of the hazard agent. Our adaptation of Kaplan’s definition emphasizes that the risk analysis must specify conditions (termed scenario) and that the results are depeiideiit on these conditions. If one describes a scenario, then the likelihood of an adverse effect for that scenario is predicted with attendant uncertainty. The elements of the triplet (scenario, likelihood, consequences) do not impose limitations on the methodology used to estimate risk. Societies can choose to control or manage risk by any number of alternative, mitigating strategies. The above definition of risk allows for hypothetical sce- narios to be compared, thus allowing a society to determine the benefits of alternative, mitigating strategies. Societies can choose not to control risks or to leave to the individual the decisions of how to manage risks. An individual might be permitted to engage in or avoid a certain risk voluntarily, such as smoking or driving a car. Management of risks that are involuntarily imposed on members of a society, such as risks of foodboi-ne illness, might cause public outrage if not handled in a consistent. open public process. Risk analysis thus is the field that provides thc public with the information needed to make informed decisions about risks and how to manage them. INTRODUCTION AND DEFINITION OF ISSUES 21 Risk analysis is simply the analysis of scenarios that result in adverse con- sequences. The overarching term “risk analysis” has come to include, in addi- tion to the above, the control and communication of risk. The management of a risk analysis has become a large undertaking, and many strategies of manag- ing it have been proposed. A common risk analysis framework encompasses three components: 1) risk assessment, 2) risk management, and 3) risk com- munication, all of which are briefly described in this introductory section. The remaining sections of this chapter discuss managerial frameworks for the risk assessment and risk management components. along with identification of some of the tensions between these frameworks. Risk Assessment Risk assessment is the estimation of the probability of the occurrence of adverse events with attendant uncertainty [National Research Council (NRC), 19831. Sound science is the underpinning of a good risk assessment, which is viewed as a link between research or science and policy (NRC, 1983). A struc- tured process is essential to risk assessment because risk rarely involves the certainty of direct, measurable observations relevant to human health but does involve inference, prediction, and uncertainty. Thus the probability of adverse consequences is formally estimated with derived models that describe mathe- matically the processes thought to produce adverse consequences. The earliest publication that dealt specifically with the structure of risk assessment applicable to foodborne human health effects was the “red book” of the NRC (1983). The basic structure or managerial framework of risk assess- ment was initially described by four elements: hazard identification, exposure assessment, dose-response assessment, and risk characterization (NRC, 1983). A list of 11 principles for risk assessment for microbiological hazards is pro- vided in Table 3.1 (CCFH, 1998). Risk Management In managing risk, the risk manager considers the results of risk assessment and other factors, including economic, political, social, and technological inputs or limitations, to develop policies to manage the risk. The decisions made by risk managers also often reflect the priorities of a society. Neither risk management nor risk assessment is conducted in a vacuum. Establishing regulatory stan- dards is a risk management activity that reflects the level of safety deemed appropriate for a given hazard. Policy strategies to control one foodborne hazard may well create new hazards. For example, air bag performance in U.S. automobiles was initially determined to minimize risks of death in severe accidents. Because of these performance standards, a new risk was created to young children and others when air bags deployed. Risk-risk trade-offs and cost-benefit analyses are essential analytical activities for fully documenting the risk management options and their consequences. A list of eight principles for 22 RISK ANALYSIS FRAMEWORKS FOR CHEMICAL AND MICROBIAL HAZARDS TABLE 3.1. General Principles of Microbiological Risk Assessment from “Principles and Guidelines for the Conduct of Microbiological Risk Assessment” Document of the Codex Committee on Food Hygiene (CCFH; 1998; M w i i ~ j merg/. WAICENTIFAOINFO/ ECO NO MICIESN/code u/Reports ktiiz) 1. Microbiological Risk Assessment must be soundly based upon science. 7 There should be a functional separation between Risk Assessment and Risk &. Management. 3. Microbiological Risk Assessment should be conducted according to a structured approach that includes Hazard Identification, Hazard Characterization. Exposure Assessment, and Risk Characterization. 4. A Microbiological Risk Assessment should clearly state the purpose of the exercise, including the form of Risk Estimate that will be the output. 5. A Microbiological Risk Assessment should be transparent. 6. Any constraints that impact on the Risk Assessment such as cost, resources or timc. should be identified and their possible consequences described. I. The Risk Estimate should contain a description of uncertainty and where the uncertainty arose during the Risk Assessment process. 8. Data should be such that uncertainty in the Risk Estimate can be determined; data and data collection systems should, as far as possible. be of sufficient quality and precision that uncertainty in the Risk Estimate is minimized. 9. A Microbiological Risk Assessment should explicitly consider the dynamics of microbiological growth, survival, and death in foods and the complexity of the interaction (including sequellae) between human and agent following consumption as well as the potential for further spread. 10. Wherever possible, Risk Estimates should be compared over time with independent human illness data. 11. A Microbiological Risk Assessment may need reevaluation as new relevant information becomes available. risk management is presented in Table 3.2 from the 1996 FAO/WHO consul- tation on risk management (WVH~. fm.orgle,,lesrilr~.sklriskco12)~~t. htm). Risk Communication Risk communication is the process of engaging stakeholders (all interested parties, including consumers, producers, scientists in academia, industry, and government, and various professional or advocacy organizations) in dialogues about risk, its assessment, and its management. The risk assessor might take responsibility for explaining in nontechnical terms the data, models, and results of the risk assessment. The risk manager is responsible for explaining the rationales for various alternative risk management strategies based on the risk assessment. The stakeholders also have a responsibility both to communicate their concerns and to review and understand the risk assessment and risk man- agement options. Some principles for agencies to apply in risk communications are listed in Table 3.3. BACKGROUND AND HISTORICAL SIGNIFICANCE 23 TABLE 3.2. General Principles of Food Safety Risk Management from Joint FAO/ WHO Expert Consultation on the Application of Risk Management to Food Safety, Rome, Italy (1996; wwwlf;lo. orgl WAICENTIFA OINFO/ECONOMIClESN/risk/ risktext. lztm) 1. Risk management should follow a structured approach. 2. Protection of human health should be the primary consideration in risk management decisions. 3. Risk management decisions and practices should be transparent. 4. Determination of risk assessment policy should be included as a specific component of risk management. 5. Risk management should ensure the scientific integrity of the risk assessment process by maintaining the functional separation of risk management and risk assessment. 6. Risk management decisions should take into account the uncertainty in the output of the risk assessment. 7. Risk management should include clear, interactive communication with consumers and other interested parties in all aspects of the process. 8. Risk management should be a continuing process that takes into account all newly generated data in the evaluation and review of risk management decisions. BACKGROUND AND HISTORICAL SIGNIFICANCE Society and Risk Analysis Risk analysis is continuously evolving to address the concerns of society. The next three sections present a short discussion of key concerns for risk analysis of interest to society. The structure of managerial frameworks of risk analysis has an effect on society because it influences how effectively risk is managed. Discussion is provided of various managerial structures and their impacts on risk communication processes. Society and risk assessment Prediction of risk and attendant uncertainty is not a simple process. In constructing risk assessment models, assumptions TABLE 3.3. FAO/WHO Principles of Risk Communication (Joint FAO/ WHO Consultation, February, 1998, Rome, Italy) 1. Know the audience. 2. Involve the scientific experts. 3. Establish expertise in communication. 4. Be a credible source of information. 5. Share responsibility. 6. Differentiate between science and value judgement. I. Assure transparency. 8. Put the risk in perspective. 24 RISK ANALYSIS FRAMEWORKS FOR CHEMICAL AND MICROBIAL HAZARDS about the underlying mechanisms or processes and formal statistical inferences from available data are made. These assumptions and inferences from limited data may be very subjective judgments that can become points of dispute and controversy for other risk analysts and stakeholders. For the risk assessment to be used effectively to support decision making about risk management strategies, the risk manager and the stakeholders must understand the risk assessment. An understanding of the risk assessment involves familiarity with: 1) the simplifying assumptions used in constructing the models; 2) whether or not the particular models used are based on consen- sus of the scientific community; 3) the magnitude of the uncertainty associated with the data and the models; and 4) the procedures for estimation of the like- lihood of the occurrence of adverse events for given scenarios. If clear. com- plete explanations of the assumptions and methodology are provided so that the analysis could be repeated, then the risk assessment is called “transparent.” However, one could say that transparency is in the eye of the beholder, because risk assessors must tailor different levels of technical detail for transparency to various audiences of stakeholders and risk analysis professionals. Besides estimating the magnitude of the risk and the uncertainty of the risk estimates in a transparent fashion, another desirable aspect of a risk assessment framework includes generality. The models must describe processes in a man- ner that permits application to a wide range of scenarios, for example, depict- ing a variety of current farm-to-fork food production processes. However, a model may also be too general, requiring many unsupported assumptions or unwarranted inferences and leading to inaccurate estimates of risk. In contrast, the data and the underlying scientific theory may permit useful estimates of risks for only a small specific set of scenarios. The underlying tension between these two poles of generality and specificity should be addressed in the preliminary phase of risk assessment. This tension can also be addressed in the risk assessment by conducting sensitivity analysis. Sensitivity analysis reveals the effect of changes in model parameters on the estimates of risk. If values of a parameter have high impact on the estimate of the risk (high sensitivity), and the actual value of the parameter is not known accurately, then the uncertainty of the risk assessment results will be great. A thorough analysis of the uncertainty of estimates and the effect of alternative models derived from different assumptions would permit evaluation of more general scenarios, while providing protection against unwarranted conclusions. Each risk assessment can be thought of as unique. Therefore, a unique combination of procedures appropriate for that risk assessment needs to be developed by the risk assessor. For example, some of the methodologies appropriate for engineering applications, such as fault tree analysis, lack the flexibility to account for dynamic growth that is necessary for modeling risk of adverse consequences from many microbial hazards. Because risk assessments are unique, the Codex Committee on Food Hygiene (CCFH) does not specify methodologies in its principles and guidelines document for microbiological hazards (Table 3.1). For example, the N R C framework (1983) discussed later BACKGROUND AND HISTORICAL SIGNIFICANCE 25 in this chapter is applicable to microbial hazards, although the methodologies to account for dynamic growth of pathogens in exposure models were probably not anticipated during formulation of the framework for carcinogenic risk assessment. For a society, the goal of risk assessment is to model realistically the proba- bilities of consequences, with attendant uncertainties, for given scenarios and not to develop “conservative” estimates of the probabilities of consequences. A model should separate for the risk manager and stakeholders “true” variability (irreducible heterogeneity among hosts, pathogens, or environmental matrix) from uncertainty (ignorance reducible by new research data) imposed by the data and the assumptions of the models. The imposition of conservatism throughout a risk assessment model is not good practice, because bias that may be difficult to quantify is imposed on the risk estimate. Conservatism should be the judgment of the risk management process or of the society, informed by the risk assessment that provides a range of possibilities rather than a worst case. Society and risk management The concerns and influence of a society on risk management might be inferred from the history of legislation passed by elected and appointed representatives and enforced by governmental regu- lators. Table 3.4 lists some key legislation that influenced food safety and risk assessment in the United States. Of particular note for this chapter is the TABLE 3.4. Some Legislative History for Food Safety and Environmental Risk Assessment (ENVIRON, 1988; Cohrssen and Covello, 1989; Code of Federal Regulations, Title 9, volume 2, Chapter 111, USDA/FSIS Statutory Requirements) 1. Clean Air Act ( I 970, amended 1974, 1977, 1990. 1997) 7 -. Clean Water Act; Safe Drinking Water Act (1972, amended 1974, 1977, 1978; 1997) 3. Comprehensive Environmental Response, Compensation, and Liability Act; Superfund Amendments and Reauthorization Act (1981; 1986) 4. Egg Products Inspection Act (1970; 84 Stat. 1620, 21 U.S.C. 1031 et ,seq.) 5. Federal Crop lnsurance Reform and Department of Agriculture Reorganization Act of 1994 (Pub. L. 103-354; 7 U.S.. 2204e) 6. Federal Food, Drug, and Cosmctic Act (1938, 52 Stat. 1040. amended 1958. 1960, 1962, 1968; amended 1996, 1998 as Food Quality Protection Act (21 U.S.C. 301 rf sec1)) 7. Federal Insecticide. Fungicide, and Rodenticide Act (1948, amended 1972, 1975, 1978; amended 1996, 1998 as Food Quality Protection Act) 8. Federal Meat Inspection Act ( 1 907, 34 Stat. 1260, amended by Wholesome Meat Act, 81 Stat. 584 (21 U.S.C. 601 et seq.)) 9. Pathogen Reduction/HACCP Rule (1996, 61 FK 38868) 10. Poultry Products Inspection Act (1957; 71 Stat. 441, as amended by Wholesome Poultry Products Act, 82 Stat. 791 (21 U.S.C. 451 clt sq.)) 1 1. President’s Food Safety Initiative ( 1 997) 26 RISK ANALYSIS FRAMEWORKS FOR CHEMICAL AND MICROBIAL HAZARDS Delaney Clause of 1958 (Table 3.4. item 6), which imposed a zero-risk cancer standard designed to prohibit food additives including pesticides with carcino- genic potential from concentrating in processed foods. A zero-risk standard does not require quantitative risk assessment, but rather a simple statistical test at some prescribed level for the presence of a carcinogen hazard in a processed food. The changing climate of risk analysis over the past 40 years is evidenced by two recent pieces of legislation that imply or explicitly require risk assessments for food safety under certain conditions. The Federal Crop Insurance Reform and Department of Agriculture Reorganization Act of 1994 established the Office of Risk Assessment and Cost-Benefit Analysis to review the risk assess- ments and cost-benefit analyses that are used in support of major regulations in the U.S. Department of Agriculture (USDA). The Food Quality Protection Act of 1996, with amendment in 1998, modified the Delaney Clause relating to pesticides and changed the language from “zero risk” to “reasonable certainty that no harm will result from aggregate exposure to pesticide residue.” Thus, “safe” food, with respect to pesticides, is not implied by a lack of statistically significant demonstration for tlie presence of a carcinogenic halard. Rather, “safe” food is implied by estimates of “reasonable” dietary risk and consider- ation of sensitive subpopulations. Society and risk communication In the past, risk assessors may not have considered that risk communication was their responsibility at all. except for coinmunicating with the risk manager. Public meetings might have been con- vened to announce the results of the risk assessment or to explain the policy decisions that were drawn from an assessment. The attitudes projected by more recent work of the NRC (1996) and the President’s Commission on Risk Management (1996) point the way to opening risk analysis to more interactive dialogue throughout the process. Thus U.S. federal agencies (EPA, USDA, and FDA) arc more commonly convening public meetings to introduce risk assessment teams and to solicit data at the start of major risk assessments rather than at the end of the assessments. Risk Management Frameworks The initial framework for risk assessment and risk management proposed by tlie NRC in 1983, as mentioned above, was the first U.S. publication that sys- tcmatically related foodborne human health, risk assessment, and risk man- agement. The work of the NRC marked the first major U.S. effort to define nomenclature for, and the key steps of, public health risk assessment (NRC, 1983). The Committee met to consider chemical hazards and carcinogenic consequences or end points. However, the work, referred to as the “Red Book.” has been applied beyond its initial scope of risk assessment for carci- n ogens. BACKGROUND AND HISTORICAL SIGNIFICANCE 27 Risk Analysis Managerial Strategies The N R C (1 983) outlined a managerial structure of risk analysis in Figure 1.1 of their report. Certain features of this figure are depicted in Eq. 3. I. Risk Risk Research +I Assessment jL2 {Decisions} eLir Management (3.1) These relationships are consistent with the concept that risk assessment is a structured process that links science and policy. The first unidirectional arrow on the left indicates that research data and information are inputs to risk assessment. However, an implication of Eq. 3.1 is that the research goals and objectives are not influenced by the needs of risk assessors. In other words, research projects are not specifically designed to meet the needs of risk assess- ments. The President’s Commission on Risk Management (1 996) recognized this problem and stated that risk assessments should motivate research. Uncer- tainty and sensitivity analyses of risk assessment models can identify crucial research needed for improving estimations of risk. The second and third unidirectional arrows emphasize the objectivity of the science and the independence of risk assessment from other influences that have bearing on the risk manager’s decision making process. Developing preliminary analyses for the scope of the risk assessment requires some initial dialogue between risk assessors and risk managers. As the assessment is conducted, a risk manager should clearly refrain from exerting any influence on the process that might bias the results. Therefore, the phases of risk assessment dealing with scientific data generally exclude risk managers. However, as a risk assess- ment nears completion, risk assessors and risk managers might jointly develop policy options for mitigation or risk reduction and economic analyses such as cost-benefit analysis for potential mitigation, regulations, or guidance. This view of risk analysis demands some dialogue between risk assessors and risk managers, but as implied by Eq. 3.1, only at the end of the process, at the decision point. The results of the risk assessment become an input to the risk manager’s decision making process. One consequence, perhaps unintended, of these uni- directional relationships is the hindrance of open and transparent communi- cation with risk managers and other stakeholders about the scientific data, assumptions, and methodologies used in the risk assessment. The possibility exists in this framework that risk assessment will become a “black box” for the risk manager as well as for the stakeholders. As a consequence of these concerns, a more general framework is needed. Equution 3.2 represents a simple extension of the NRC model utilizing bidirec- tional arrows. Research u Risk Assessment u Risk Management (3.4 28 RISK ANALYSIS FRAMEWORKS FOR CHEMICAL AND MICROBIAL HAZARDS The left bidirectional arrow implies that research should be motivated and directed by the needs of risk assessors in conducting risk assessments. This reflects a new emphasis on the opportunity for risk assessors to identify key data gaps and to take an active part in influencing the direction of research to improve risk assessment models. The right bidirectional arrow implies that not only IS decision making a joint enterprise between risk assessors and risk man- agers, but also the assumptions and inferences used by the risk assessors must be communicated clearly to the risk managers. The bidirectional arrow on the right is not meant t o imply that the influence of risk managers diminishes the independence of the risk assessment, but as a consequence independence could be diminished. Eqiirrtioii 3.1 still does not explicitly include risk communication structure. The NRC 1983 framework appears to omit risk communication with the stakeholders. One motivation for change, or expansion of the framework, involves including stakeholders who want to understand risk and to choose how they respond to risk as individuals and societies (NRC, 1996). Many risk analysts and stakeholders recognize that the usefulness of unidirectional pro- cesses for risk analysis merits further scrutiny (President’s Commission on Risk Management, 1996; Marks, 1998; FDA, 1999). Many levels of communication are needed among researchers, risk assessors, and managers. For example, dialogue is necessary between the risk assessors and managers for defining the scope of the risk assessment, preparing and testing models for various risk management options, engaging in discussions about the results and interpretations of the results of the risk assessment, and influencing the direction of the research. However, communication between the risk analysis professioiials and the stakeholders is more complicated. For example, one of the many sources of difficulty is the depth of technical knowl- edge required to understand the risk assessment, Many technical assumptions are made that can raise questions about the validity of the results. Another problem is that risk assessments often do not address the problem in its entirety, but rather address a portion of it, omitting other associated hazards that could become concerns to the community (NRC, 1996). For stakeholders to develop a better understanding of the nature of the problem and to evaluate possible solutions to the problem, it is necessary for them to understand in detail the risk assessment process. The next section provides a discussion of the nature and limitations of the risk assessment process. SCIENTIFIC BASIS AND IMPLICATIONS Risk Assessment Structure The first task for risk assessors, given an assignment to conduct a risk assess- ment from their risk managers, is to compile the evidence and structure it in some reasonable manner according to a logical framework, such as the four- SCIENTIFIC BASIS AND IMPLICATIONS 29 c occurrence I, deoiity Risk’ characterization 1 Rid Estimate with Attendant Uncertaintv Figure 3.1. Structure of model for microbial risk (Marks et al., 1998; with permission from Risk Anulysis). element framework of the NRC (1983). This framework is sufficiently general to be useful for chemical and microbial hazards (Fig. 3.1; Marks, 1998). As discussed above, the output of a risk assessment is the estimation of the proba- bility and severity of adverse outcomes for given scenarios, according to our modification of Kaplan’s definition of risk. Hazard ZdentiJication (HI) is the first element of the 1983 NRC framework that describes the nature of the problem and the agents that cause adverse effects in a given scenario. Many types of adverse outcomes or “end points” can be considered. Toxicological or epidemiological studies are used to dem- onstrate an association of the hazard in food or water with human health risk. However, the identification of a hazard may be controversial, especially for chemical risk assessments that depend on extrapolation from animal studies and may consider only a single chronic “end point.” E.xposure Assessment (EA) is the second element that focuses on modeling the occurrence and level of hazards, and the potential ingestion of the hazards in the food, which cause or contribute to adverse outcomes. An EA would typically include an assessment of a hazard in a particular food for given sce- narios that describe the production, processing, distribution, and preparation of the food. In addition, the EA must assess the eating habits of the target pop- ulations. This assessment is often accomplished by examining consumption surveys or large databases of surveys such as the USDA Continuing Survey of 30 RISK ANALYSIS FRAMEWORKS FOR CHEMICAL AND MICROBIAL HAZARDS Food Intake by Individuals. Often, however, there is difficulty in categorizing the foods that are surveyed so that they correspond to the types of foods that contain the hazards. Chemical risk assessment must take into account the fact that a chemical in food can undergo changes during processing and preparation. Thus, to realis- tically model exposure, an understanding of food chemistry of the hazardous chemical is necessary. In microbiological risk assessment, the concern is possi- ble continuous growth and decline of pathogens in the food. Methodologies to realistically model chemical changes and microbial growth and decline are still under development by risk assessors. Stakeholders should know that many technical assumptions for EA are based on very limited data. Because a great deal of information is not known with certainty, simplifying assumptions are often made that could lead to an overstatement of the confidence of the results. For example, the major dis- tinguishing feature for microbial pathogens is modeling to account for the dynamics of niicrobial growth and decline, termed predictive microbiology. However, at the time of this writing, EA models for microbial pathogens in foods have not explicitly distinguished strain variability, which can be large for some bacterial pathogens. Data are usually available for only a few strains or a cocktail or mixture of several strains, which may differ taxonomically and biologically from the hazard of interest. From the behavior of a few strains, inferences are made for all strains, without regard to population variability. Another example is that predictive microbiology models are designed to be "conservative" rather than unbiased. Reasons for bias include features of the experimental design such as the use of high levels of pure cultures of a cocktail or mixture of pathogen strains grown under optimal conditions in complete nutrient broth in the total absence of the competing microflora of foods. In reality, pathogen growth is influenced by many factors not explicitly accounted for in the models. Another emerging facet of EA, in which simplifying assump- tions are made, is modeling the potential for person-to-person transmission in addition to dietary exposure for certain foodborne disease agents (Eisen- berg et al., 1996). Dosc-Res~xmseAssessnwrzt (DRA), the third element of the NRC frame- work, involves modeling the relationship between the ingested dose of the hazard and the likelihood and severity of the adverse effect. Much of the work of dose-response modelers in chemical risk assessment involves analysis of data from animal studies. For microbial risk assessment animal studies are not often used, but the DRA depends primarily on data from a small set of controlled clinical studies in which human volunteers were administered the hazard, usu- ally at high doses. In chemical risk assessment, mechanistic or genetic con- siderations could be applied that can contradict the results of animal studies ( i v i v w. e p g o v/oppspsl/jqpa). When extrapolating beyond the observed range of the data from clinical or animal studies to the low-dose region, the model form can have dramatic effects on the outcome (Coleman, 1998). Another issue with which dose- SCIENTIFIC BASIS AND IMPLICATIONS 31 response modelers must wrestle, particularly in the microbial area, is the de- velopment of surrogate dose-response models in the absence of data for the hazard of interest. Chemical risk assessors make inferences about chemicals for which no dose-response information is known ( U S. EPA and LogiChem, 1997) from chemicals with a similarity of chemical structures for which some information is known. However, in the microbial area, apparently, knowledge or information is not available for making such inferences. Questions about the structural aspects of host-pathogen interactions must be considered to deter- mine plausible surrogates. For microbial risk assessors, selection of surrogate dose-response models will continue to be of interest as long as new pathogenic strains evolve and are recognized. Outputs of the dose-response model are the frequency and severity of human foodborne illness at a given exposure or ingested dose. The complexity of predicting frequency or probability and severity of illness must be emphasized by risk assessors. Illness is a complex function of variabil- ity in all aspects of the epidemiological disease triangle of host, pathogen, and environment (matrix) effects and their interactions. A clear association between age of human hosts and frequency of illness for microbial hazards has emerged from epidemiological surveillance and outbreak investigations (CDC, 1998; Coleman, 1998; Terajima et al., 1999). However, these data are not an ideal proxy for age dependence in dose-response relationships because ingested doses are unknown. Pathogen strains are likely to vary in many aspects of growth, physiology, and both the presence and expression of virulence genes. An example of an environmental effect is that fat in foods appears to provide a protective environment for pathogens, enabling them to survive in inhospitable surroundings. A tremendous amount of controversy is associated with DRA. Rkk Charucterizution (RC), the fourth element of the NRC framework, begins with linking the output of the EA models with the DRA models to pre- dict the frequency and severity of human illness (the consequence) for given scenarios. R C commonly relies on techniques such as Monte Carlo simulation. Principles of good practice for Monte Carlo simulation have been published to guide risk assessors in developing sound risk assessment models ( Burmaster and Anderson, 1994). The major output of R C is a series of distributions of the frequency and severity of illness for the subpopulations of interest. Often, risk assessors may estimate illness for certain subpopulations under a baseline (as is) scenario and with interventions or possible system failures. Such a process bridges risk assessment and risk management activities and might include developing the concept of comparative risk, the comparison of simula- tion results for the baseline (as is) and various potential mitigation scenarios most relevant to policy makers. This type of analysis provides information about the relative contribution of different interventions to risk reduction that is necessary to support policy making. A key analytical aspect of R C is the performance of sensitivity and uncer- tainty analyses to determine what variables most strongly affect the uncertainty of the risk estimate. Another aspect of RC involves validating the model or 32 RISK ANALYSIS FRAMEWORKS FOR CHEMICAL AND MICROBIAL HAZARDS testing the predictive abilities of the model (Bowker, 1993). Standard statistical procedures such as goodness of fit testing and construction of confidence inter- vals for predictions can be used. However, because risk assessment models are often so complex, other procedures that are nonparametric are used for vali- dation (Bowker, 1993). The most effective way of validating the model is com- paring the estimates derived from the model with an independent source of data. The problem is that often there are questions concerning the validity of the independent source of data. For example, active surveillance data from the FoodNet study (USDA, 1998) provide some insight into the possible magni- tude of the rates of illness from foodborne pathogens within and between the sentinel sites. However, a variety of difficulties exist in interpretation of these data. Principles of appropriate analysis used in the RC, and more generally in the risk assessment, are given on pages 100-101 of an informative book, Under- stcinding Risk: Znfumzing Decisions in a Denmcratic Society (NRC, 1996). The principles are for the most part readily understood and include the following concepts: Analysis should be consistent with the state of the art; analysis should be checked for accuracy; assumptions should be clearly pointed out; and superfluous assumptions should be discarded. However, one standard or prin- ciple does create some difficulty, the principle that “Calculations are presented in such a form that they can be checked by others interested in verifying the results.” There are two real problems associated with this principle. The first is that often the mathematical and statistical procedures used are so complex that, unless the computer programming is independently recreated, the results cannot be verified. In reality, verification of the analysis is just not possible for most interested parties. A second potential problem with this principle is that managers and risk assessors, in an attempt to adhere to this principle, may simplify procedures and adopt less than state-of-the-art methodologies, con- tradicting the first principle. Our preferred statement of the principle is that methodologies, including mathematical derivations and justification of statisti- cal procedures, should be presented in a clear and coinplete fashion and in accordance with standard practices of the mathematical and statistical profes- sions. Computer programs, in addition to reported results: should be made available to any interested party (USDA, 1998). Developing Risk Communication Risk assessment is highly technical and not without controversy. The coni- munication of the results is difficult and decisions made as a result of a risk assessment could be controversial. As noted above, the 1983 NRC depiction did not include a risk communication component. Because of the complexities of a risk assessment, the uncertainty of the results, and the large stakes involved in the decisions, distrust may arise among the various stakeholders and risk analysis professionals. The National Research Council (1996) addresses these and other problems SCIENTIFIC BASIS AND IMPLICATIONS 33 in an innovative manner. The concept of risk characterization is expanded to include more than the summary of mathematical models and statistical analy- ses associated with risk assessment, defined by the NRC “red book” (1983). By 1996, representatives of the NRC conceived of risk characterization as a decision-driven activity “to enhance practical understanding and to illuminate practical choices.” Thus stakeholders, either directly or through surrogate rep- resentatives, should be involved with the risk assessment from the beginning. The expanded risk characterization process thus can incorporate social, behav- ioral, economic, and ethical aspects of risk. T o make the risk characterization relevant to all parties, the NRC not only includes an analytical component of risk characterization, but adds what is termed a “deliberation” component (NRC, 1996). Thus, risk characterization is an “analytical-deliberative” pro- cess. The new expanded definition (NRC, 1996) is as follows: “RC is a syn- thesis and summary of information about a potentially hazardous situation that addresses the needs and interests of decision makers and affected parties. R C is a prelude to decision making and depends upon an iterative analytical- deliberative process.” Thus, risk characterization is no longer in the domain of risk assessment or is no longer in the hands of the sponsors or managers of the risk assessment. Rather, R C becomes a public or political process. In addi- tion to the term “analytical-deliberative,’’ the NRC also introduces the term “iterative.” This term is meant to imply that the risk characterization is a give- and-take process between participants and can include an updating of the risk assessment. The above definition represents an all-inclusive process, and seems to imply an ongoing process. Of particular interest to us in this chapter is the deliberative process as part of the risk characterization. Deliberation involves the exchange of ideas, opin- ions, reflections on others’ opinions, and so forth. Deliberation is defined for- mally by the NRC (1996) to be “any formal or informal process for communi- cation and for raising and collectively considering issues.” The N R C states that the “deliberation frames the analysis and the analysis informs the deliberation” (NRC, 1996). Two phases of the deliberation are specifically identified. First, for a risk assessment, scenarios must be defined at the beginning of the process, consistent with our definition of risk being conditional on well-defined scenar- ios. All stakeholders should be involved in constructing the scenarios. The sec- ond phase is formulation of decisions from the results of the assessment. A very extensive discussion identifies and describes the principles and difficulties of the deliberative process (NRC, 1996). Disagreement and controversy are inherent in a deliberative process (NRC, 1996). The NRC encourages organizations not to truncate the analyti- cal-deliberative process but, on the other hand, not to delay needed actions under the guise of needing more analysis. In fact, the latter possibility repre- sents a difficulty that needs to be dealt with from the beginning. The N R C gives examples of ongoing risk assessments, where ongoing monitoring might ensure that the assumptions and theories used in the risk assessment are valid and thus 34 RISK ANALYSIS FRAMEWORKS FOR CHEMICAL AND MICROBIAL HAZARDS the initial decisions are still valid. But often risk asses