Metallurgy & Materials Science PDF
Document Details
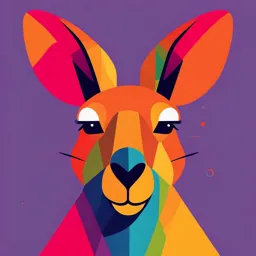
Uploaded by RapturousNovaculite6644
Amrita School of Engineering
Dr. R. VairaVignesh
Tags
Summary
This document is a presentation of metallurgical and materials science, focusing on topics such as heat treatment and stress and strain. It details various procedures and concepts within the field.
Full Transcript
Metallurgy & Materials Science Dr. R. VairaVignesh Assistant Professor (Senior Grade) – Research Track Department of Mechanical Engineering, Amrita School of Engineering - Coimbatore The contents and images in the presentatio...
Metallurgy & Materials Science Dr. R. VairaVignesh Assistant Professor (Senior Grade) – Research Track Department of Mechanical Engineering, Amrita School of Engineering - Coimbatore The contents and images in the presentation are obtained from internet or articles or books that may be protected by copyright. The presentation is distributed freely but only for classroom instruction or the use of students. The presentation may not be retained or disseminated. Any further use of this material may be in violation of Exceptions To Infringement Under Copyright Act (Government of India) Please do not remove the credit line on the title page and republish the file as your own, in whole or in part. Heat Treatment of Steels Stress and Strain ▪ Non-uniform plastic deformation – manufacturing sequence Hooke’s Law ▪ Machining, Welding, Grinding ▪ Deformation processing (hot-work / cold-work) ▪ Thermal variation ▪ Phase transformations ▪ Heterogeneity of a chemical or crystallographic order 3 Stress and Strain Machining sequence ▪ Load is removed – material tries to recover the elastic part of deformation ▪ Inhibited from full recovery – adjacent plastically deformed material 4 Stress and Strain Heterogeneity of a chemical or crystallographic order ▪ Dislocation of atoms ▪ Compressive strain field (large solute) ▪ Tensile strain field (small solute) ▪ Lattice distortion ▪ Distortion – strain ▪ Strain results in stress 5 Stress and Strain Phase Transformation BCC ▪ Martensite – diffusion less shear-type FCC transformation ▪ Fe-C system – rapid quench to vicinity of ambient ▪ FCC Austenite – polymorphically Orthorhombic transform – BCT Martensite ▪ Huge lattice distortion – high BC - Tetragonal stress field near C atoms 6 Cementite Residual Stresses ▪ Stresses – present in matrix – after removal of load (thermal or mechanical) – residual stresses ▪ Residual stresses can be positive or negative – depending on the application ▪ Laser peening – Imparts compressive residual stresses to the surface – strengthening of thin sections or the toughening of brittle surfaces ▪ Typically, however, residual stresses have X-Ray Diffraction Analysis negative effects nλ = 2d sinθ 7 Residual Stresses ▪ Residual stresses – often invisible to a manufacturer – unless significant distortion – negatively affect structural integrity ▪ Thick-walled structures in the as-welded condition are more prone to brittle fracture than ▪ Residual stress – often a cause of premature a structure that has been stress-relieved failure of critical components ▪ Undesired stresses also have an effect on the fatigue ▪ Residual stress – probably a factor in the collapse performance of the Silver Bridge in West Virginia, United States in December 1967. https://www.thestructuralengineer.info/news/the-collapse-of-silver- bridge 8 Heat Treatment Heat Treatment ▪ Combination of heating and cooling operations, timed and applied to a metal or alloy in the solid state in a way that will produce desired properties ▪ Heat-treatment – changes in the micro-constituents of the metal ▪ Changes in the microconstituents – nature, form, size and distribution in the metal piece ▪ Heat treatment – applied to ingots, castings, semi-finished products, welded joints and various elements of machines and instruments 9 Purpose of Heat Treatment ▪ To relieve internal stresses set up during cold- working, casting, welding and hot-working treatments ▪ To refine the grain structure of metal (coarse; fine; engineering phases) ▪ To augment the material properties (mechanical, corrosion, tribological properties) 10 Heat Treatment of Steels ▪ Steel – alloy of Fe and C with the C content between a few hundreds of a percent up to about 2 weight % ▪ Other alloying elements ▪ Total to about 5 weight % in low-alloy steels ▪ Higher in more highly alloyed steels such as tool steels and stainless steels ▪ Steels – exhibit a wide variety of properties ▪ Composition ▪ Phases and microconstituents – which in turn depend on the heat treatment ▪ Heat treating of steels, the liquid phase is always avoided 11 Quick Review ▪ Austenite – Solid solution of C and / or other alloying elements in γ-Fe; not stable in room temperature (unless, stabilizers such as Mn, Ni, Co, & Cu added deliberately) – FCC (Stable) ▪ Ferrite – BCC Iron phase with limited solubility of C (α-Fe) – BCC (S) ▪ Cementite – Fe3C (6.67 wt.% C) – Complex Orthorhombic (Meta) ▪ Leduburite – Eutectic mixture of Austenite and Cementite ▪ Pearlite – Alternate lamellae of Ferrite and Cementite (austenite decomposition by eutectoid reaction) 12 Quick Review ▪ Martensite – Austenite transformation below Ms (240°C) temperature – shear type transformation (M) ▪ Bainite – Austenite transforms at temperature below at which Pearlite produced and above at which Martensite is formed (250°C-650°C) (M) ▪ Troosite – Radial lamellae of Ferrite and Cementite – Tempering of Martensite below 450°C ▪ Sorbite – Ferrite and finely divided Cementite – Tempering of Martensite above 450°C 13 Quick Review ▪ A1 – 727°C (Eutectoid transformation temperature) ▪ Acm – γ-Fe / γ-Fe + Fe3C boundary ▪ A2 – 768°C – Curie temperature (768°C) – Ferro- ▪ Accm – Hypereutectoid steel – solution of magnetic to Para-magnetic upon heating cementite is completed during heating ▪ Arcm – Hypereutectoid steel – precipitation ▪ A3 – γ-Fe / γ-Fe + α-Fe boundary of cementite is starts during cooling ▪ Ac3 – Hypoeutectoid steel – Transformation of ferrite to austenite is completed during heating ▪ A4 – δ-Fe transforms to austenite during cooling ▪ Ar3 – Hypoeutectoid steel – Austenite begins to ▪ 1147 °C (Eutectic transformation temperature) transform to ferrite during cooling ▪ 1495 °C (Peritectic / Monotectic reaction) 14 Heat Treatment of Steels ▪ Annealing of Steels ▪ Tempering ▪ Stress-relief ▪ Martempering ▪ Normalizing ▪ Austempering ▪ Full Annealing ▪ Precipitation Hardening ▪ Spheroidizing ▪ Solution Heat Treating ▪ Quenching ▪ Precipitation Heat Treating 15 Annealing ▪ Annealing – generic term – consists of heating to and holding at a suitable temperature followed by cooling at an appropriate rate, primarily for the softening of metallic materials ▪ Steels – annealed to facilitate cold working or machining, to improve mechanical or electrical properties, or to promote dimensional stability ▪ Annealing Cycles – maximum temperature – A1, A3, Acm ▪ Some Austenite is present at temperatures above A1, cooling practice through transformation is a crucial factor in achieving desired microstructure and properties 16 Annealing Annealing Cycles ▪ Subcritical (Stress-Relief, Process Annealing) ▪ Below A1 ▪ Inter-critical annealing ▪ Above A1, but below A3 Spheroidizing ▪ Above A1, but below Acm Process Annealing ▪ Super-critical (Full annealing, Normalizing) Stress-Relief ▪ Above A3 or Acm Hypo-Eutectoid Steel Hyper-Eutectoid Steel 17 Annealing Stress-Relief Annealing ▪ Relieve stresses that remain locked in a structure – consequence of a manufacturing sequence ▪ Separates stress-relief heat treating from Spheroidizing post-weld heat treating Process Annealing ▪ Post-weld heat treating – relief of residual Stress-Relief stresses & preferred metallurgical structure or properties Hypo-Eutectoid Steel Hyper-Eutectoid Steel 18 Annealing Stress-Relief Annealing ▪ Stress-relief heat treating ▪ Uniform heating of a structure, or portion thereof to a suitable temperature below the transformation range Spheroidizing ▪ Holding at this temperature for a Process Annealing predetermined period of time Stress-Relief ▪ Uniform cooling Hypo-Eutectoid Steel Hyper-Eutectoid Steel 19 Annealing Stress-Relief Annealing ▪ Care – ensure uniform cooling – variable section size ▪ Cooling rate – not constant and uniform ▪ New residual stresses – equal to or greater than existing 20 Annealing Stress-Relief Annealing ▪ Residual stresses ▪ Stress-corrosion cracking (SCC) near welds or cold-strained regions ▪ Ferritic steel – significant reduction in resistance to brittle fracture (reduces ductility) ▪ Cold strain – reduction in creep strength at elevated temperatures 21 Annealing Normalizing ▪ Normalizing – heating at least 55°C above A3 for compositions less than the eutectoid, and above Acm for compositions greater than the eutectoid Spheroidizing ▪ After sufficient time - completely transform to Process Annealing austenite – procedure termed austenitizing Stress-Relief ▪ Treatment is terminated by cooling in air Hypo-Eutectoid Steel Hyper-Eutectoid Steel 22 Annealing Normalizing ▪ Steels – plastically deformed – consist of grains of pearlite (and most likely a proeutectoid phase) irregularly shaped and relatively large, but vary substantially in size Spheroidizing ▪ Normalizing – refine the grains (decrease Process Annealing average grain size) + more uniform and Stress-Relief desirable size distribution Hypo-Eutectoid Steel Hyper-Eutectoid Steel 23 Annealing Full – Annealing ▪ Annealing temperature 50 °C above ▪ A3 for hypoeutectoid steels ▪ A1 for hypereutectoid steels Hypoeutectoid Steels (under 0.83% C) Spheroidizing ▪ Heat above A3 (supercritical annealing) to Process Annealing attain full austenitization – steel is fully Stress-Relief austenitic at the annealing temperature Hypo-Eutectoid Steel Hyper-Eutectoid Steel 24 Annealing Full – Annealing Hypoeutectoid Steels (under 0.83% C) ▪ Lower-carbon steels – carbides are unstable at temperatures above A1 – dissolve in the austenite (dissolution may be slow) Spheroidizing ▪ Approximately eutectoid carbon contents – Process Annealing lamellar transformation product if Stress-Relief austenitized for very long periods of time Hypo-Eutectoid Steel Hyper-Eutectoid Steel 25 Annealing Full – Annealing Hypereutectoid Steels (above 0.83% C) ▪ Heat above A1 temperature – dual-phase austenite-cementite region ▪ Hypereutectoid steels – extremely soft by Spheroidizing holding for long periods of time at the Process Annealing austenitizing temperature Stress-Relief ▪ Long-term austenitizing – effective Hypo-Eutectoid Steel Hyper-Eutectoid Steel 26 Annealing Full – Annealing Hypereutectoid Steels (above 0.83% C) ▪ Long-term austenitizing – effective ▪ Because – produces agglomeration of residual carbides in the austenite Spheroidizing ▪ Coarser carbides promote a softer final Process Annealing product Stress-Relief Hypo-Eutectoid Steel Hyper-Eutectoid Steel 27 Annealing Spheroidizing ▪ Strength of the ferrite depends on its grain size and cooling rate ▪ Carbides – as lamellae in pearlite or spheroids radically affects the formability of steel Spheroidizing Process Annealing ▪ Spheroidized Steels – globular carbides in a Stress-Relief ferritic matrix + lower the flow stress Hypo-Eutectoid Steel Hyper-Eutectoid Steel 28 Annealing Spheroidizing ▪ Flow stress - stress that must be applied to cause a material to deform at a constant strain rate in its plastic range ▪ Flow stress - determined by the proportion Spheroidizing and distribution of ferrite and carbides Process Annealing ▪ Majority spheroidizing performed to improve Stress-Relief cold formability of steels & machinability of hypereutectoid steels and tool steels Hypo-Eutectoid Steel Hyper-Eutectoid Steel 29 Annealing Spheroidizing ▪ Spheroidization in steels ▪ Prolonged holding at just below A1 ▪ Heating and cooling alternately – just above and just below A1 Spheroidizing ▪ Heating to a temperature just above A1, Process Annealing and then either cooling very slowly in the Stress-Relief furnace or holding at just below A1 Hypo-Eutectoid Steel Hyper-Eutectoid Steel 30 Annealing Spheroidizing ▪ Spheroidization in steels ▪ Cooling at suitable rate from minimum temperature ▪ Carbide – dissolved to prevent Spheroidizing reformation of a carbide network Process Annealing ▪ Reheating with above procedures Stress-Relief (applicable to hypereutectoid steel containing a carbide network) Hypo-Eutectoid Steel Hyper-Eutectoid Steel 31 Annealing Spheroidizing ▪ Full spheroidizing – austenitizing either slightly above the A1 temperature or about midway between A1 and A3 ▪ Low-carbon steels are seldom spheroidized Spheroidizing for machining Process Annealing ▪ Excessively soft Stress-Relief ▪ Severe deformation of material Hypo-Eutectoid Steel Hyper-Eutectoid Steel 32 Annealing Process Annealing ▪ During cold working (cold sawing and cold shearing) ▪ Hardness of steel increases ▪ Ductility decreases Spheroidizing ▪ Additional cold reduction – difficult Process Annealing ▪ Must be annealed to restore its ductility Stress-Relief ▪ Process annealing – merely to soften Hypo-Eutectoid Steel Hyper-Eutectoid Steel 33 Annealing Process Annealing ▪ Wire industry – intermediate treatment between the drawing of wire to a size slightly larger than the desired finished size ▪ Such annealing between processing steps – Spheroidizing in-process or simply process annealing Process Annealing ▪ Ensures ductility and hence avoids breaking of Stress-Relief wire during continuous drawing process Hypo-Eutectoid Steel Hyper-Eutectoid Steel 34 Annealing Process Annealing ▪ Most instances – subcritical treatment is adequate ▪ Heating to a temperature (between 10°C and 20°C) below A1 Spheroidizing ▪ Soaking for an appropriate time Process Annealing ▪ Cooling, usually in air Stress-Relief Hypo-Eutectoid Steel Hyper-Eutectoid Steel 35 Quenching ▪ Quenching – process of rapidly cooling metal parts from the austenitizing or solution treating temperature; Stainless and high-alloy steels – quenched ▪ Minimize the presence of grain boundary carbides ▪ Improve the ferrite distribution ▪ Controlled amounts of martensite in the microstructure ▪ Successful hardening – achieving the required microstructure, hardness, Plate Martensite and Retained strength, or toughness while minimizing residual stress, distortion, and Austenite the possibility of cracking 36 Tempering ▪ Steels – tempered by reheating after hardening ▪ Obtain specific values of mechanical properties ▪ Relieve quenching stresses ▪ Ensure dimensional stability ▪ Also to relieve the stresses and reduce hardness developed during welding and to relieve stresses induced by forming and machining ▪ Tempering – previously hardened or normalized steel – heated to a temperature below lower critical temperature and cooled at a suitable rate, primarily to increase ductility and toughness, but also to increase the grain size of the matrix 37 Tempering ▪ Three distinct stages of tempering (even though the temperature ranges overlap) Stage I: Formation of transition carbides and lowering of the carbon content of the martensite to 0.25% (100 to 250 °C) Stage II: Transformation of retained austenite to ferrite and cementite (200 to 300 °C) Stage III: Replacement of transition carbides and low-temperature martensite by cementite and ferrite (250 to 350 °C) Additional stage of tempering (Stage IV) exists for high-alloy steels – precipitation of finely dispersed alloy carbides 38 Martempering ▪ Martempering – describe an interrupted quench from the austenitizing temperature of certain alloy (cast, tool, and stainless steels) ▪ Purpose – delay the cooling just above the martensitic transformation for a length of time, to equalize the temperature throughout the piece ▪ Minimize the distortion, cracking, and residual stress ▪ Martempering – somewhat misleading and is better described as marquenching ▪ Microstructure after martempering – essentially primary martensitic that is untempered and brittle 39 Martempering ▪ Quenching from the austenitizing temperature into a hot fluid medium (hot oil, molten salt, molten metal, or a fluidized particle bed) at a temperature usually above the martensite range (Ms point) ▪ Holding in the quenching medium until the temperature throughout the steel is substantially uniform ▪ Cooling (usually in air) at a moderate rate to prevent large differences in temperature between the outside and the center of the section ▪ Formation of martensite occurs fairly uniformly throughout the workpiece during cooling to room temperature, thereby avoiding formation of excessive amounts of residual stress 40 Austempering ▪ Austempering – isothermal transformation of a ferrous alloy at a temperature below that of pearlite formation and above that of martensite formation ▪ Steel is austempered by being: ▪ Heated to a temperature within the austenitizing range, usually 790 to 915 °C ▪ Quenched in a bath maintained at a constant temperature, usually in the range of 260 to 400 °C ▪ Allowed to transform isothermally to bainite in this bath ▪ Cooled to room temperature 41 Austempering ▪ Molten salt – quenching medium most commonly used in austempering because: ▪ Rapid heat transfer - eliminates the problem of a vapor phase barrier during the initial stage of quenching; viscosity is uniform over a wide range of temperature ▪ Austempering of steel offers several potential advantages: ▪ Increased ductility, toughness, and strength at a given hardness ▪ Reduced distortion, which lessens subsequent machining time, stock removal, sorting, inspection, and scrap ▪ Shortest overall time cycle to through-harden within the hardness range of 35 to 55 HRC, with resulting savings in energy and capital investment 42 Surface Hardening of Steels Surface Hardening of Steels Carburizing ▪ Requirement – high toughness in core and high hardness on the surface ▪ Carburizing – diffusion of carbon; Thermochemical process ▪ Low-carbon steel, plain carbon steels, alloy steels Carbon content – max. of 0.4% and less than 0.25% ▪ Carbon – diffused into the surface of the steel at a temperature above A3 45 Carburizing ▪ High carbon content in surface due to rapid diffusion and high solubility of carbon in Austenite ▪ Quenched and tempered – surface becomes a high-carbon tempered martensite – ferritic centre remains soft and ductile ▪ Thickness of the hardened surface – case depth – smaller in carburized steels than in flame- or induction hardened steels ▪ Increase in C content at the surface – high enough to give a martensitic layer with sufficient hardness, typically 700 HV (wear-resistant surface) 46 Carburizing Gas carburising ▪ Component – furnace with an atmosphere of methane or propane + neutral carrier gas (mixture of N2, CO, CO2, H2 and CH4) ▪ Carburising temperature (925°C) – methane (or propane) decomposes – component surface to atomic carbon and hydrogen ▪ Carburising time: 2 hours for a 1 mm depth case to a maximum of around 36 hours for a 4 mm case 47 Carburizing Liquid carburising (or cyaniding) ▪ Component – salt bath (cyanide-chloride- carbonate mixture) at 845°C to 955°C ▪ Cyanide salts introduce a small amount of nitrogen into the surface - improves its hardness – added advantage ▪ Fastest carburising process ▪ Suitable only for small batch sizes 48 Carburizing Solid (pack) carburising ▪ Components – surrounded by a carburising medium (coke or charcoal mixed with barium carbonate) and placed in a sealed box ▪ Process – one of gas carburisation – CO produced dissociates into CO2 – Carbon diffuses into the components' surface ▪ Temperatures – 790°C to 845°C for times of 2 hours to 36 hours ▪ Pack carburising – least sophisticated carburising process – widely used method 49 Nitriding ▪ Nitriding – surface hardening process ▪ Early 1900s – continues to play an important role in many industrial applications ▪ Manufacture of aircraft, bearings, automotive components, textile machinery, and turbine systems ▪ Develops a very hard case – at relatively low temperature, without the need for quenching ▪ Does not require a phase change from ferrite to austenite, nor a further change from austenite to martensite ▪ Steel remains in the ferrite phase (or cementite, depending on alloy composition) during complete procedure 50 Nitriding ▪ No molecular size change + no dimensional https://www.sciencedirect.com/science/article/pii/B9780857095923500069 change ▪ Only slight growth due to the volumetric change of the steel surface caused by the nitrogen diffusion ▪ Distortion – induced by surface stresses being released by the heat of the process, causing movement in the form of twisting and bending Microstructure of a compound layer, produced by bath nitrocarburising and 51 oxidising; 580°C/150 min.; Steel: 42CrMo4; etching: Oberhoffer’s reagent. Nitriding ▪ Nitriding – ferritic thermochemical method of diffusing nascent nitrogen into the surface of steels and cast irons ▪ Diffusion process – based on the solubility of N in Fe ▪ Solubility limit of nitrogen in iron – temperature dependent ▪ At 450°C – Iron-base alloy will absorb up to 5.7 to 6.1% of N ▪ Beyond – surface phase tends to be predominantly ε phase ▪ High carbon => more potential for ε phase formation 52 Nitriding ▪ Process Parameters for Gas Nitriding ▪ Furnace temperature ▪ Process control ▪ Time ▪ Gas flow ▪ Gas activity control ▪ Process chamber maintenance 53 Carbo-Nitriding ▪ Carbo-Nitriding – modified form of gas carburizing, rather than a form of nitriding ▪ Modification – introducing ammonia into the gas carburizing atmosphere to add nitrogen to the carburized case as it is being produced ▪ Nascent nitrogen forms at the work surface by the dissociation of ammonia in the furnace atmosphere ▪ Nitrogen diffuses into the steel simultaneously with Carbon ▪ Typically – lower temperature and for a shorter time than gas carburizing ▪ Produces a shallower case than is usual in production carburizing ▪ Effects on steel – carbonitriding is similar to liquid cyaniding 54 Carbo-Nitriding ▪ Because of problems in disposing of cyanide-bearing wastes, ▪ Carbonitriding is preferred over liquid cyaniding ▪ Carbonitriding – imparts a hard, wear-resistant case, generally from 0.075 to 0.75 mm deep ▪ Carbonitrided case – better hardenability than a carburized case ▪ Nitrogen increases the hardenability of steel ▪ Also an austenite stabilizer => high nitrogen levels results in retained austenite (alloy steels) ▪ Consequently, by carbonitriding and quenching, a hardened case can be produced at less expense within the case-depth range indicated, using either carbon or low-alloy steel 55 Carbo-Nitriding Process ▪ Carburizing at 900 to 955 °C to give the desired total case depth (up to 2.5 mm) ▪ Followed by carbonitriding for 2 to 6 h in the temperature range of 815 to 900 °C to add the desired carbonitrided case depth ▪ Oil quenched to obtain a deeper effective and thus harder case than would have resulted from the carburizing process alone ▪ Addition of the carbonitrided surface increases the case residual compressive stress level and thus improves contact fatigue resistance as well as increasing the case strength gradient 56 Carbo-Nitriding ▪ Full hardness with less distortion can be achieved with oil quenching, or, in some instances, even gas quenching, employing a protective atmosphere as the quenching medium ▪ Commonly carbonitrided steels –1000, 1100, 1200, 1300, 1500, 4000, 4100, 4600, 5100, 6100, 8600, and 8700 series – Carbon up to about 0.25% ▪ Also, Carbon range of 0.30 to 0.50% - carbonitrided to case depths up to about 0.3 mm ▪ Combination of reasonably tough, through-hardened core and a hard, long-wearing surface – required (shafts and transmission gears) ▪ Steels such as 4140, 5130, 5140, 8640, and 4340 for heavy-duty gearing treated by this method at 845°C 57 THANK YOU [email protected] [email protected] sites.google.com/site/rvairavignesh The contents and images in the presentation are obtained from internet or articles or books that may be protected by copyright. The presentation is distributed freely but only for classroom instruction or the use of students. The presentation may not be retained or disseminated. Any further use of this material may be in violation of Exceptions To Infringement Under Copyright Act, 1957 (Government of India) Please do not remove the credit line on the title page and republish the file as your own, in whole or in part.