Enzymes Lecture Notes 2024 PDF
Document Details
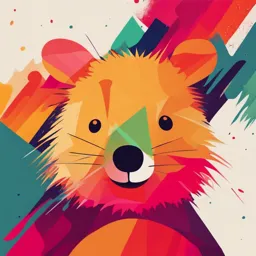
Uploaded by WorthyVerism
Temple University
2024
Marc A. Ilies, Ph.D.
Tags
Summary
These are lecture notes on enzymes from Lehninger, Chapter 6 for the 2024 academic year. The notes cover the biological importance of enzymes, their mechanisms, and kinetics. The document also includes various tables concerning enzymes and their classification.
Full Transcript
6. Enzymes Marc A. Ilies, Ph. D. Lehninger - Chapter 6 (p189-242) [email protected]; lab 517, office 517A (Tu,1 Fr 3-5) For questions, comments please use the discussion tool in Canvas or recitation session ©MAIlies20...
6. Enzymes Marc A. Ilies, Ph. D. Lehninger - Chapter 6 (p189-242) [email protected]; lab 517, office 517A (Tu,1 Fr 3-5) For questions, comments please use the discussion tool in Canvas or recitation session ©MAIlies2024 Part 1 – Principles of enzymatic catalysis Enzymes are catalysts There are two fundamental conditions of life: Ability to self-replicate Ability to catalyze chemical reactions efficiently and selectively Enzymes are biochemical catalysts, central to every biochemical process increase reaction rates without being used up they increase the rate of both the direct reaction and the inverse reaction, helping to reach thermodynamic equilibrium faster Why biocatalysis with enzymes is superior to inorganic catalysts? Enzymes have a greater reaction specificity: avoids side products Enzymes require milder reaction conditions: conducive to conditions in cells pH ~ 7, 37°C Enzymes ensure higher reaction rates: in a biologically useful timeframe Enzymes ensure capacity for regulation and control of biological pathways - - COO COO NH2 Metabolites have many - potential pathways of O COO OH COO - decomposition. - - O COO Chorismate COO - Enzymes make the COO OH mutase - OOC O desired one most favorable. NH2 OH Enzymes in disease states Enzymes can be used as biomarkers to diagnose disease The amount of a certain enzyme may increase/decrease in a particular disease state The activity of certain enzymes may increase or decrease in diseases Many drugs interact with enzymes Most enzymes are globular proteins however, some RNA (ribozymes and ribosomal RNA) also can catalyze reactions. catalytic activity depends on the integrity of the native fold (all primary, secondary, tertiary and quaternary structures are important for catalysis Sometimes catalytic activity depends on the presence of prosthetic groups: cofactors (inorganic ions Fe2+, Mg2+, Mn2+, Zn2+) and coenzymes (NAD+, biotin, thiamine, etc): Protein part denominated apoenzyme/apoprotein Apoenzyme + prosthetic group(s) = Holoenzyme TABLE 6-1 Some Inorganic Ions That Serve as Cofactors for Enzymes Ions Enzymes Cu2+ Cytochrome oxidase Fe2+ or Fe3+ Cytochrome oxidase, catalase, peroxidase K+ Pyruvate kinase Hexokinase, glucose 6-phosphatase, Mg2+ pyruvate kinase Mn2+ Arginase, ribonucleotide reductase Mo Dinitrogenase Ni2+ Urease Carbonic anhydrase, alcohol dehydrogenase, carboxypeptidases Zn2+ A and B These ions are covalently bound to the corresponding enzyme – the enzyme cannot function (is not active) without them! Some Coenzymes That Serve as Transient Carriers of Specific Atoms or TABLE 6-2 Functional Groups Coenzyme Examples of chemical groups Dietary precursor in mammals transferred Biocytin CO2 Biotin Coenzyme A Acyl groups Pantothenic acid and other compounds 5'-Deoxyadenosylcobalamin H atoms and alkyl groups Vitamin B12 (coenzyme B12) Flavin adenine dinucleotide Electrons Riboflavin (vitamin B2) Lipoate Electrons and acyl groups Not required in diet Nicotinamide adenine Hydride ion (:H–) Nicotinic acid (niacin) dinucleotide Pyridoxal phosphate Amino groups Pyridoxine (vitamin B6) Tetrahydrofolate One-carbon groups Folate Thiamine pyrophosphate Aldehydes Thiamine (vitamin B1) Note: The structures and modes of action of these coenzymes are described in Part II. Enzymes are classified by the reactions they catalyze TABLE 6-3 International Classification of Enzymes Class no. Class name Type of reaction catalyzed 1 Oxidoreductases Transfer of electrons (hydride ions or H atoms) 2 Transferases Group transfer reactions Hydrolysis reactions (transfer of functional groups to 3 Hydrolases water) Cleavage of C—C, C—O, C—N, or other bonds by 4 Lyases elimination, leaving double bonds or rings, or addition of groups to double bonds Transfer of groups within molecules to yield isomeric 5 Isomerases forms Formation of C—C, C—S, C—O, and C—N bonds by 6 Ligases condensation reactions coupled to cleavage of ATP or similar cofactor Detailed classification and name by Enzyme Commission Numbers Trivial name varies: - name of substrate + “ase” - broad function performed, etc Enzymes have substrate selectivity OH H H - + OOC NH3 - + OOC NH3 H - OOC + NH3 No binding OH HO OH H H Binding but no reaction H NH CH3 Example: phenylalanine hydroxylase Enzyme-Substrate Complex Drives Selectivity enzyme-catalyzed reaction occurs within the confines of a pocket of an enzyme called the active site: molecule bound in the active site and processed by the enzyme is called substrate the enzyme-substrate complex is central to the action of enzymes: E+S ES EP E+P Enzymatic Catalysis E+S ES EP E+P Enzymes do not affect the position and direction of equilibrium (K ) eq …which means that enzymes cannot affect the free energy of the reaction (ΔG) – enzymes do not affect the thermodynamics of a reaction! Slow reactions face significant activation barriers (ΔG≠) that must be surmounted during the reaction. Enzymes increase reaction rates (k) by decreasing activation energy of the reaction ΔG ≠ - enzymes alter the kinetics of the reaction! kB T G k exp h RT Examples of Rate Enhancement by Enzymes Some Rate Enhancements Produced by TABLE 6-5 Enzymes Cyclophilin 105 Carbonic anhydrase 107 Triose phosphate isomerase 109 Carboxypeptidase A 1011 Phosphoglucomutase 1012 Succinyl-CoA transferase 1013 Urease 1014 Orotidine monophosphate decarboxylase 1017 Reaction Coordinate Diagram From kinetic point of view, the rate of the reaction is dependent on the activation energy ΔG≠ The transition state (≠) is a particular configuration during the advance of the reaction (reaction coordinate) with the highest potential energy; not to be confused with ES or EP complexes, which are reactive intermediates! (defines the advance of the reaction SP) Enzymes Decrease ΔG≠ Enzymes form metastable intermediates with substrate (ES) and with products (EP) called reaction intermediates and decrease the activation energy of the reaction ΔG≠ The rate-limiting step is the step with the highest activation energy → will dictate the overall rate of the reaction How enzymes lower G Enzymes organize reactive groups into close proximity and orient them properly for the chemical reaction to occur optimally Uncatalyzed bimolecular reactions Two free reactants single restricted transition state conversion is entropically unfavorable. Uncatalyzed unimolecular reactions Flexible reactant rigid transition state conversion is entropically unfavorable for flexible reactants. Catalyzed reactions The enzyme uses the binding energy of substrates to organize the reactants to a fairly rigid ES (and subsequently EP) complex. The entropy cost is paid during binding (compensated with binding energy) Rigid reactant complex transition state conversion is entropically neutral. Binding energy is a major source of free energy used by enzymes to lower the activation energies of biochemical reaction How does binding energy help? 1. Entropy reduction (see next slide) 2. Desolvation – E-S interactions replace hydrogen bonds between S and water 3. Compensation of substrate distortion – Binding energy compensates for energy needed for substrate to distort 4. Enzyme conformation (induced fit) – Binding energy helps with enzyme conformation 16 Support for the Proximity Model The rate of anhydride formation from esters and carboxylates shows a strong dependence on proximity of two reactive groups: How enzymes lower G Enzymes bind transition states best. The idea was proposed by Linus Pauling in 1946. – Enzyme active sites are made complimentary to the transition state of the reaction catalyzed – Weak interactions are optimized for the reaction transition state within the active site of the enzyme activation energy is dramatically reduced. – Enzymes bind transition states better than substrates. Illustration of TS Stabilization Idea: Imaginary Stickase Catalytic mechanisms of enzymes Enzymes may use one or a combination of the following: – acid-base catalysis: give and take protons – covalent catalysis: change reaction paths – metal ion catalysis: use redox cofactors, pKa shifters General Acid-Base Catalysis Proton transfer is optimized within the active site of the enzyme: Amino acid residues within the active site of the enzyme, properly oriented, donate and receive protons Amino Acids in General Acid-Base Catalysis Covalent Catalysis A transient covalent bond is usually formed between the enzyme and the substrate Changes the reaction pathway – uncatalyzed: H2O A—B AB – catalyzed (X = catalyst): H2O A — B X : A — X B A X : B Requires a nucleophile on the enzyme – can be a reactive serine, thiolate, amine, or carboxylate Metal Ion Catalysis Involves a metal ion bound to the enzyme Interacts with substrate to facilitate binding – stabilizes negative charges Participates in oxidation reactions Chymotrypsin During digestion, dietary proteins must be broken down into small peptides by proteases. Chymotrypsin is one of several proteases that cuts peptides at specific locations on the peptide backbone. This protease is able to cleave the peptide bond adjacent to aromatic amino acids. Chymotrypsin cuts this bond. Chymotrypsin Uses Most of the Enzymatic Mechanisms Structure of chymotrypsin: polypeptide backbone has a ribbon structure, with many β-sheets and some α-helices; disulfide bonds stabilize the structure Chymotrypsin Mechanism Step 1: Substrate Binding Chymotrypsin Mechanism Step 2: Nucleophilic Attack Chymotrypsin Mechanism Step 3: Substrate Cleavage Chymotrypsin Mechanism Step 4: Water Comes In Chymotrypsin Mechanism Step 5: Water Attacks Chymotrypsin Mechanism Step 6: Break-off from the Enzyme Chymotrypsin Mechanism Step 7: Product Dissociates Chymotrypsin Mechanism https://www.youtube.com/watch?v=6kYpu1eZZHs 34 Part 2: Enzyme kinetics What Is Enzyme Kinetics? Kinetics is the study of the rate at which compounds react. The rate of enzymatic reaction is affected by: – enzyme – substrate – effectors – temperature Why Study Enzyme Kinetics? Quantitative description of biocatalysis Determine the order of binding of substrates Elucidate acid-base catalysis Understand catalytic mechanism Find effective inhibitors Understand regulation of activity Derivation of Enzyme Kinetics Equations Enzyme kinetics: Determine the rate of a reaction and how this rate is changing in response with different experimental parameters 1. Start with a model mechanism. 2. Identify constraints and assumptions. 3. Carry out algebra... –... or graph theory for complex reactions. Simplest Model Mechanism: E + S ES E + P – one reactant, one product, no inhibitors Identify Constraints and Assumptions k1 k2 E+S ES P k-1 Total enzyme concentration is constant. – The mass balance equation for enzymes is [E ] = [E] + [ES]. Total – It is also implicitly assumed that S = [S] + [ES] ≈ [S] since Total enzyme is usually present in a concentration [S] and the [S] term in the denominator of the Michaelis-Menten equation becomes insignificant. The equation simplifies to V0 = Vmax[S]/Km and V0 exhibits a linear dependence on [S] (first order kinetics) At high [S], where [S] >> Km, the Km term in the denominator of the Michaelis- Menten equation becomes insignificant and the equation simplifies to V0 = Vmax; the reaction is zero order and does not depend on [S]. Determination of Kinetic Parameters A nonlinear Michaelis-Menten plot should be used to calculate parameters Km and Vmax. k cat [E tot ][S] V max[S] v K m [S] Km [S] Lineweaver-Burk Plot: Linearized, Double-Reciprocal A linearized double-reciprocal Lineweaver-Burk Plot is good for analysis of two-substrate data or inhibition: k cat [E tot ][S] V max[S] v K m [S] Km [S] y a x + b Enzyme catalytic efficiency kcat/KM Each enzyme has different kcat, KM Best way to compare efficiencies is to refer to kcat/KM , a measure of catalytic efficiency of the enzyme; this specificity constant is a direct measure of the efficiency of the enzyme in transforming substrate and combines ability to bind substrate and to transform it into product Some enzymes are so efficient that diffusion of S into active site and diffusion of P from active site are the limiting steps (diffusion controlled limit: ~ 10 8 – 109 M-1s-1) Enzymes for Which kcat/Km Is Close to the Diffusion-Controlled Limit TABLE 6-8 (108 to 109 M–1s–1) kcat/Km Enzyme Substrate kcat (S–1) Km (S–1) (M–1s–1) Acetylcholinesterase Acetylcholine 1.4 x 104 9 x 10–5 1.6 x108 CO2 1 x 106 1.2 x 10–2 8.3 x 107 Carbonic anhydrase HCO3– 4 x 105 2.6 x 10–2 1.5 x 107 Catalase H2O2 4 x 107 1.1 x 100 4 x 107 Crotonase Crotonyl-CoA 5.7 x103 2 x 10–5 2.8 x 108 Fumarate 8 x 102 5 x 10–6 1.6 x 108 Fumarase Malate 9 x 102 2.5 x 10–5 3.6 x 107 β-Lactamase Benzylpenicillin 2.0 x 103 2 x 10–5 1 x 108 Source: A. Fersht, Structure and Mechanism in Protein Science, p. 166, W. H. Freeman and Company, 1999. Enzyme Inhibition Inhibitors are compounds that decrease an enzyme’s activity. Irreversible inhibitors (inactivators) react with the enzyme. One inhibitor molecule can permanently shut off one enzyme molecule (suicide inhibitors) They are often powerful toxins but also may be used as drugs. Reversible inhibitors bind to and can dissociate from the enzyme. They are often structural analogs of substrates or products. They are often used as drugs to slow down a specific enzyme. Reversible inhibitor can bind to: the free enzyme and prevent the binding of the substrate. the enzyme-substrate complex and prevent the reaction. Reversible inhibitors can be classified into: Competitive Uncompetitive Mixed Competitive Inhibition Inhibitor competes with substrate for binding – binds active site – does not affect catalysis Competitive Inhibition No change in Vmax; apparent increase in KM Lineweaver-Burk: lines intersect at the y-axis. Uncompetitive Inhibition Inhibitor only binds to ES complex does not affect substrate binding inhibits catalytic function Uncompetitive Inhibition Decrease in Vmax; apparent decrease in KM No change in KM/Vmax Lineweaver-Burk: lines are parallel. Mixed Inhibition Binds enzyme with or without substrate ― binds to regulatory site ― inhibits both substrate binding and catalysis Mixed Inhibition Decrease in Vmax; apparent change in KM Lineweaver-Burk: lines intersect left from the y- axis. Irreversible (suicide) inhibitors enzyme behaves in a normal fashion but “commits suicide” by becoming inactivated by covalently bound substrate suicide inhibitors also called mechanism-based inactivators because they highjack the normal enzyme reaction mechanism to inactivate it Example: irreversible binding of diisopropylflurophosphate (DIFP or DFP) to cholinesterase: Cholinesterase Covalent bond 54 Enzyme Activity Can Be Regulated Regulation can be: – noncovalent modification (allosteric) – covalent modification – irreversible – reversible Noncovalent Modification: Allosteric Regulators Allosteric effectors or modulators are generally small chemicals that bind to a site different from substrate binding site, modifying the fold Allosteric effectors can be positive, or improve enzymatic catalysis. Allosteric effectors can be negative, or reduce enzymatic catalysis. Some Reversible Covalent Modifications Zymogens Are Activated by Irreversible Covalent Modification Some Enzymes Use Multiple Types of Regulation Goals and Objectives Upon completion of this lecture at minimum you should be able to answer the following: ► What are enzymes, which properties they display, where are they useful? ► Which biomolecules can act as enzymes, what prosthetic groups they require? ► How are enzymes classified, what is their name/nomenclature? ► Explain the substrate selectivity of the enzymes and present the particularities of enzyme kinetics, how enzymes accelerate biochemical reactions? ► Present the impact of enzymes to G of biochemical reactions, use of binding energy ► Which are the main catalytic mechanisms use by enzymes? ► What is enzyme kinetics and why are we studying it? ► What is the Michaelis-Menten equation and what is the significance of the kcat, Km, kcat/Km?; How one can determine the kinetic parameters? ► Which are the particularities of kinetics at low/high substrate concentration? ► What are enzyme inhibitors, types of inhibitors and their particularities? ► What are the main characteristics of competitive, uncompetitive and mixed inhibition of enzymes? ► What are irreversible inhibitors? ► How enzyme activity can be regulated? ► What are allosteric regulators and how they can act on enzymes? ► What examples of reversible and irreversible covalent modification of enzymes you know? 60