Enzyme Mechanisms Chapter 7 CH250 PDF
Document Details
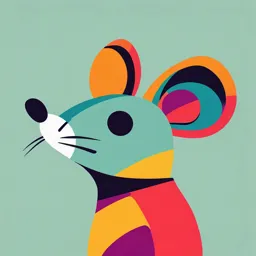
Uploaded by DynamicUkiyoE
2024
James H. Gerlach
Tags
Summary
This document is an introductory biochemistry chapter on enzyme mechanisms. It explains how enzymes work and how they increase the rates of reactions. It explores the induced fit model, catalytic mechanisms, and various factors affecting enzyme efficiency including pH and temperature.
Full Transcript
Enzyme Mechanisms Chapter 7 CH250 Introductory Biochemistry © 2024 James H. Gerlach Dietary modifications and statin drugs are commonly used to reduce serum cholesterol. Statin drugs target the enzyme HMG- CoA reductase, which is an enzyme in the cholesterol biosynthetic pathway. H...
Enzyme Mechanisms Chapter 7 CH250 Introductory Biochemistry © 2024 James H. Gerlach Dietary modifications and statin drugs are commonly used to reduce serum cholesterol. Statin drugs target the enzyme HMG- CoA reductase, which is an enzyme in the cholesterol biosynthetic pathway. Here, the statin drug atorvastatin is shown bound to the active site of HMG-CoA reductase resulting in Chapter Outline 7.1 Overview of Enzymes 7.2 Enzyme Structure and Function 7.3 Enzyme Reaction Mechanisms 7.4 Enzyme Kinetics 7.5 Regulation of Enzyme Activity 7.1 Overview of Enzymes Enzymes usually bind substrates with high specificity. Enzyme active sites are physical pockets or clefts in an enzyme where the substrates bind, and catalytic reactions take place. Substrate binding to the active site is often associated with structural changes in the enzyme. The structural changes may be relatively minor or may involve significant changes in the conformation of the enzyme. Enzyme activity is highly regulated in cells. Enzyme regulation is necessary to maximize energy balance between catabolic and anabolic pathways and to alter cell behavior in response to environmental stimuli. An Example of the Induced-Fit Model of Enzyme Catalysis Is the Glycolytic Enzyme Hexokinase Enzymes Are Chemical Catalysts Chemical catalysts Increase the rate of a reaction Are not altered by the reaction Do NOT alter the ratios of substrates and products at equilibrium Therefore, there is no change to the equilibrium or the change in Gibbs energy (ΔG) Catalytic mechanism Increase the reaction rate by lowering activation energy (EA) of the reaction Hydrogen peroxide decomposition 2 H2O2 ⇌ 2 H2O + O2 ΔG° = −119.2 kJ·mol−1 Inorganic catalyst: Fe3+ 30,000x Enzyme catalyst: catalase 100,000,000x The Enzyme Catalase Has a Heme Group Buried Within the Enzyme Active Site Catalysts Increase the Rates of Reactions by Lowering the Activation Energy Reaction Coordinate vs. Reaction Time Cofactors and Coenzymes Cofactors Aid in the catalytic reaction mechanism within the active site Provide additional chemical groups to supplement amino acids Include a variety of inorganic ions involved in redox reactions e.g., Fe2+, Cu2+, Mg2+, Mn2+, Zn2+ Coenzyme Enzyme cofactors with organic components Prosthetic groups Coenzymes that are permanently associated with enzymes Include vitamin derived species e.g., Nicotinamide adenine dinucleotide (NAD+/NADH + H+) derived from niacin and thiamine pyrophosphate (TPP) derived from vitamin B1 Nicotinamide Adenine Dinucleotide Is a Coenzyme in the Lactate Dehydrogenase Reaction What is the origin of the common names of enzymes? Substrate specificity Name includes the substrate on which the enzyme acts. e.g., proteases acts on proteins, lipases acts on lipids Type of reaction Name includes the chemical reaction catalyzed by the enzyme. e.g., synthases catalyze synthesis reactions, phosphatases remove a phosphate group Source or location Name includes the source organism or the location where the enzyme is found. e.g., Taq DNA polymerase is named after the thermophilic bacterium Thermus aquaticus Historical names Name is based on historical or common usage. e.g., pepsin is named after the Greek word peptein, meaning to digest The International Union of Biochemistry and Molecular Biology (IUBMB) Nomenclature System The IUBMB classification number for hexokinase is EC 2.7.1.1. This EC number is based on classification "2" as a transferase, subclass "7" because it transfers a phosphoryl group, and sub-subclass 1 because the phosphoryl transfer involves an alcohol acceptor group on glucose. The last digit in EC 2.7.1.1 denotes that it is the first enzyme activity named in this category. 7.2 Enzyme Structure and Function Three major ways enzymes increase the rate of reactions: Stabilize the transition state Lowers the activation barrier Provide an alternative path for product formation May involve formation of stable reaction intermediates that are covalently attached to the enzyme Orient the substrates appropriately for the reaction to occur Reduces the entropy change of the reaction Enzymes use all of these strategies to some extent, sometimes in combination for the same reaction Net result = increased reaction rate on a biological timescale The Enzyme Active Site Provides an Optimal Environment That Promotes Product Formation The Enzyme Active Site Facilitates Formation of the Transition State Enzymes Perform Work in the Cell Three general categories of enzyme-catalyzed reactions: Coenzyme-dependent redox reactions Responsible for much of the energy conversion in a cell Central components of 3 major metabolic pathways: The citrate cycle; the electron transport system; the photosynthetic light reactions Metabolite transformation reactions Essential steps in all biosynthetic (anabolic) and degradative (catabolic) pathways e.g., Isomerization, condensation, hydrolysis and dehydration reactions Reversible covalent modification reactions Attach or remove molecular tags on biomolecules to alter properties and regulate cellular processes e.g., Phosphorylation/dephosphorylation of signalling molecules 7.3 Enzyme Reaction Mechanisms Chymotrypsin is a serine protease During digestion, dietary proteins and polypeptides must be broken down into small peptides Chymotrypsin is a digestive enzyme component of pancreatic juice acting in the duodenum, where it performs proteolysis Chymotrypsin is an endopeptidase that hydrolyzes the peptide backbone Chymotrypsin preferentially cleaves peptide amide bonds where the side chain of the amino acid N-terminal to the scissile amide bond (the P1 position) is a large, hydrophobic amino acid (tyrosine, tryptophan or phenylalanine) Chymotrypsin also hydrolyzes other amide bonds in peptides at slower rates, particularly those containing leucine and methionine at the P 1 position Chymotrypsin Reaction Mechanism Chymotrypsin enzymatic mechanism involves covalent catalysis by the catalytic triad The nucleophile (Ser 195) is polarized and oriented by the base (His 57) that is bound and stabilized by the acid (Asp 102) Catalysis is performed in two stages Acylation phase Peptide bond cleaved Ester linkage formed between peptide carbonyl carbon and enzyme Deacylation phase Ester linkage is hydrolysed Enzyme is regenerated Chymotrypsin Preferred Cleavage Site Aromatic amino acid Chymotrypsin hydrolyzes this peptide bond Bovine Chymotrypsin Consists of Three Polypeptide Chains Linked Together by Disulfide Bonds Catalytic Triad in the Chymotrypsin Enzyme Active Site Proposed Catalytic Mechanism of Chymotrypsin Regions of the Substrate Binding Pockets in Serine Proteases Provide Amino Acid Specificity 7.4 Enzyme Kinetics Kinetics Greek kinetikos = “moving” Quantitative study of enzyme catalysis Enzyme function depends on: The relative concentration of enzyme and substrate Temperature, pH and ionic concentration The presence or absence of inhibitors or activators Usually study initial reaction rates Little decrease in substrate concentration Little accumulation of product Michaelis–Menten Kinetics Maud Menten (1 of 2) Born in 1879 in Port Lambton, ON, raised in British Columbia Studied medicine at University of Toronto BA 1904, Master's degree in Physiology 1907, MD 1911 Women were not allowed to do research in Canada Moved to Berlin, Germany to work with Leonor Michaelis (1912) Showed that the rate of an enzyme-catalyzed reaction is proportional to the amount of the enzyme-substrate complex Received her PhD from University of Chicago in 1916 Held six degrees from prestigious universities, three of which were doctorate degrees in medicine, physiology and biochemistry Maud Menten (2 of 2) Pathologist at University of Pittsburgh (1923–1950) Her final promotion to full Professor, in 1948, was at the age of 69 in the last year of her career Research fellow at the British Columbia Medical Research Institute (1951–1953) Most famous for her work on enzyme kinetics with Michaelis Also invented the azo-dye coupling reaction for alkaline phosphatase, which is still used in histochemistry Inducted in 1998 into The Canadian Medical Hall of Fame Although Menten did most of her research in the United States, she retained her Canadian citizenship throughout her life Michaelis–Menten Kinetic Parameters Are Obtained From Experimental Data Relative Concentrations of Reaction Components Change Over the Course of an Enzyme Reaction Determining the Michaelis– Menten Parameters Meaning of Vmax and Km (1 of 2) The Michaelis–Menten Equation and the Michaelis–Menten equation Basis for most single-substrate enzyme kinetics Variables 𝑉 max [ S] ν0 = Initial reaction velocity [S] = Initial substrate concentration 𝑣0= Vmax and Km = Kinetic parameters 𝐾 m +[ S] Crucial assumptions “Quasi-steady-state assumption” – that the [ES] is essentially constant The total enzyme concentration ([Et]) does not change over time Initial rates used because [S] is very close to the initial [S] and product concentration [P] is very close to zero (no reverse reaction) Meaning of Vmax and Km (2 of 2) The Michaelis–Menten Equation and the Very low [S] [S] > Km 𝑣0= ≈ ≈ 𝑉 max 𝐾 m +[ S] [S ] Zero-order region Rate not influenced by [S] [S] = Km 𝑉 max [S] 𝑉 max [S ] 𝑉 max 𝑣0= ≈ ≈ 𝐾 m +[ S] 2[S] 2 Definition of Km Km = Michaelis constant Why are Km and Vmax so important? (1 of 2) Michaelis constant (Km) Indicates how “good” an enzyme is Lower Km lower [S] that it is effective Km tends to be similar to cellular [S] Vmax Represents the maximum rate achieved by the system but depends on total enzyme concentration [Et] Vmax directly proportional to [Et] Used to calculate the turnover number Why are Km and Vmax so important? (2 of 2) Turnover number (kcat) Indicates how “fast” an enzyme is Equivalent to the number of substrate molecules converted to product molecules in a given unit of time by a single enzyme molecule when the enzyme is saturated with substrate kcat = Vmax / [Et] where [Et] = [E] + [ES] Comparing Catalytic Mechanisms and Efficiencies Kinetic parameters kcat and Km Useful for study and comparison of enzymes Reflects cellular environment, normal substrate concentration and chemistry of the reaction Both needed to evaluate kinetic efficiency Specificity constant (kcat/Km) The best way to compare the catalytic efficiencies of different enzyme is to compare the ratio kcat/Km for the two reactions enzymes or the turnover of different substrates by the same Upper limit of kcat/Km set by diffusion limits In aqueous solutions 108 to 109 M-1 s-1 Enzyme Sensitivity Sensitivity to pH Most enzymes are only active within 3 – 4 pH units Usually due to presence of charged amino acids in the active site and/or substrate Sensitivity to temperature The rate of enzyme-catalyzed reactions increases with temperature until protein denatures Optimum temperature varies with organism Optimal pH and Temperature of an Enzyme Reaction 7.5 Regulation of Enzyme Activity Enzyme regulation is mediated by two mechanisms: Bioavailability of enzyme The amount of enzyme in different tissues and cellular compartments Control of enzyme catalytic efficiency by protein modification Covalent and non-covalent chemical bonds Types of regulation Stimulatory – overall increase in enzyme activity e.g., Increased enzyme synthesis, increased catalytic efficiency Inhibitory – overall decrease in enzyme activity e.g., Decreased enzyme bioavailability, disturbance of protein structure Enzyme Bioavailability and Catalytic Efficiency Are the Two Main Enzyme Regulatory Mechanisms Mechanism of Enzyme Inhibition Reversible inhibition Due to the noncovalent binding of small biomolecules or proteins to the enzyme subunit Irreversible inhibition Inhibitory molecule forms a covalent bond with catalytic groups in the active site of the enzyme Less commonly forms very strong noncovalent interactions Reversing inhibition The effect of reversible inhibitors can be decreased by diluting the enzyme reaction Irreversible inhibitors are unaffected by dilution Diisopropylfluorophosphate (DFP) Is an Irreversible Inhibitor of Enzymes Three Distinct Classes of Reversible Enzyme Inhibitors Have Been Characterized E = enzyme; I = inhibitor; P = product; S = substrate; EI = enzyme–inhibitor complex; ES = enzyme–substrate complex; ESI = enzyme–substrate–inhibitor complex Structure of an HIV Protease Dimer With the Competitive Inhibitor Indinavir Bound to the Active Site Aspartate Transcarbamoylase (ATCase) Activity Is Feedback Inhibited by CTP ATCase Is a Cooperative Enzyme and Is Allosterically Regulated by ATP and CTP Allosteric Regulation of ATCase Activity by CTP and ATP CTP Binding Shifts ATCase From an Active R State to an Inactive T State Covalent Modification Can Act As an On/Off Switch for Enzyme Activity Regulation by Proteolytic Cleavage Chymotrypsin Is Generated by Proteolytic Processing of Chymotrypsinogen Into Three Polypeptide Chains Regulation of Digestive Enzymes by Proteolytic Processing Enteropeptidase (membrane-bound)