Chapter 24: Molecular Biology DNA & Genes PDF
Document Details
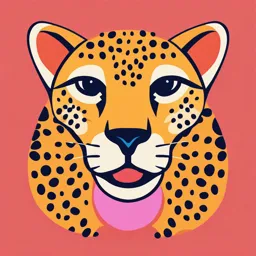
Uploaded by RecommendedMountRushmore
Daniella Aleshinloye
Tags
Summary
This document explains the central dogma of molecular biology, detailing how genetic information flows from DNA to RNA to proteins. It compares and contrasts the characteristics of eukaryotic and prokaryotic genomes, discussing concepts like chromosomes, DNA structure, and gene organization. The document also touches on aspects of DNA replication and other related genetic concepts.
Full Transcript
Daniella Aleshinloye Chapter 24 1. Explain the Central Dogma of Molecular Biology and describe how genes have evolved The Central Dogma of molecular biology describes how genetic information moves. It describes that information moved into a protein cannot be reversed....
Daniella Aleshinloye Chapter 24 1. Explain the Central Dogma of Molecular Biology and describe how genes have evolved The Central Dogma of molecular biology describes how genetic information moves. It describes that information moved into a protein cannot be reversed. DNA is replicated and transcribed into RNA, then translated into proteins. Transcription describes how one strand of the double-stranded DNA acts as a template for the synthesis of RNA via mRNA. Translation describes how a triplet codon of nucleotide in mRNA binds to triplets in tRNA that are complementary. One amino acid represents a triplet, and they are part of the tRNA molecules. Amino acids are arranged in peptides and the sequence determines its function. Genes were thought to be responsible for the phenotype or genotype. Then, through mutation studies, each gene was thought to code for one enzyme. Currently, genes are described as segments of DNA that code for RNA or protein that are different from regulatory sequences, and a gene can code for different products. 2. Compare & contrast the genes and genomes of Eukaryotes and Prokaryotes Compare: Both of them undergo translation and transcription for replication. Contrast: Eukaryotes Prokaryotes The somatic cells of humans are diploid, Each cell contains only one and they contain 22 pairs of chromosome, which contains only one chromosomes and X/Y chromosomes, gene copy. which are 46 chromosomes in total. The DNA is arranged into multiple The DNA is arranged into nucleoids chromosomes in the nucleus The DNA is linear The DNA is circular The DNA is packed around proteins The DNA binds to proteins for a short called histones. period of time. Contains telomeres, and introns It does not contain telomeres and introns It is less compact. It is more compact. Contains 50% of repetitive DNA Does not contain repetitive DNA 3. Compare & contrast the DNA and genes in the cytosol, nucleoids, nucleus, mitochondria, & chloroplasts Cytosol: Observed in prokaryotes, it contains the nucleoid, which contains the DNA. Nucleus: Observed in eukaryotes, it contains DNA arranged into chromosomes. Nucleoids: Observed in prokaryotes, it contains the circular bacterial DNA Mitochondria: Contains double-stranded circular DNA that encodes its own mitochondrial tRNA, rRNA, and proteins. Chloroplast: Contains double-stranded circular DNA that encodes for proteins in the chloroplast. 4. Define DNA, chromosomes, genes, genome, centromeres and telomeres, and complexity DNA: It is deoxyribonucleic acid that contains all the genetic information for an organism. Chromosome: A large molecule composed of DNA and proteins that contain genes for storing and transporting. Genes: It is a sequence of DNA or RNA that codes for a particular function. Centromeres: A region in the chromosomes that holds two daughter chromosomes during mitosis, which is important for equal and orderly sharing of chromosomes. It is 130bp long, and it is abundant in A=T pairs. Telomers: A region that ends the eukaryotic chromosome. The telomere repeat sequence in humans is TTAGGG, which is shortened in each round of replication. Complexity: The length of DNA or the number of chromosomes in an organism does not relate to the complexity of the organism. This is due to the fact that most eukaryotic DNA is noncoding. 5. Summarize the composition of the Human Genome The human genome contains a small amounts of genes that encode proteins; they are called exons. The non-coding DNA includes introns, the region of genes that are transcribed but not translated as they are spliced out before protein translation. Non-coding DNA regions regulate the gene expressions via promoters and termination signals. Noncoding DNA can also encode for small regulatory RNA, and some can be junk (unwanted genes). Half of the human genome is repetitive and is not fully static due to sequences called transposons that can move around the cell's genome. They contain simple sequence repeats, including satellite DNA, which is repetitive non-coding DNA, which is associated with centromeres and telomeres. 6. Describe DNA supercoiling and relate it to twist, writhe, and linking number DNA supercoiling is a level of organization that allows for the packing of DNA molecules in a cell and allows for proteins to access for reading information in the DNA sequence. Supercoiling is tightly regulated as it influences the transcription and replication of DNA. DNA that is not supercoiled is referred to as being in a relaxed state. DNA is most stable in the B-from structure. When DNAs have no breaks, they are closed-circular DNAs. 1. Twist is the number of turns or twists of the helix in the DNA. 2. Writhe is the number of supercoils, which is usually negative. 3. The linking number is Twist + Writhe that remains constant until the DNA is broken (via topoisomerases) 7. Differentiate the two type of topoisomerases and describe how each type works Topoisomerase catalyzes changes in the linking number required for unwinding and rewinding DNA during transcription and replication. a) Type I (topoisomerase I and III) function is to remove negative supercoils in order to relax the DNA. They cut one DNA strand transiently, using single-strand breaks. b) Type II (topoisomerase II and IV) function is to relax negative and positive supercoils in humans (cannot introduce them). They cut the two DNA stands transiently using ATP and double-strand breaks. In bacteria (E.coli), DNA gyrase can introduce negative supercoils. 8. Clinical Correlation: Explain how topoisomerases are targets for antibiotics and chemotherapy Antibiotics: Coumarines inhibit ATP binding of bacterial type II topoisomerases. Quinoles inhibit DNA strand breaks from reselling (the last step of the topo reaction) and target bacterial enzymes. Chemotherapy: Anticancer drugs target cancer cells because they are rapidly growing cells, and rapidly growing cells express topoisomerase. Eukaryotic type I topo inhibits function by trapping the enzyme-DNA complex in its cleaved state. 9. Describe and summarize the changes in eukaryotic chromosome structure during the cell cycle Go is the non-dividing state, and interphase includes G1(gap 1), S (synthesis), and G2 (gap 2), in which the chromatin is undefined and dispersed randomly. In the S phase, the chromatin in its undefined state is replicated, and each chromosome produces two sister chromosomes that are still joined together. Then, in the prophase of mitosis, the chromosomes become condensed, and the pairs of sister chromatids form. 10. Define DNA packing, nucleosomes, histone, and chromosomal scaffold as well as how each one interacts w/DNA DNA packing is the process by which DNA strands are tightly packed into the nucleus. DNA is tightly wrapped around proteins called histones, which form nucleosomes. The nucleosomes are coiled into chromatin containing DNA fibers, histone proteins, and a little RNA. Nucleosomes look like beads on a string containing 147bp of DNA wrapped around 8 histones, which form a left-handed solenoid. Histones are small basic (Lys, Arg) proteins that can be modified by acetylation, SUMOylating, ubiquitination, ADP-ribosylation, methylation, phosphorylation, and glycosylation via their amino-terminal tails. Chromosomal scaffold is involved in a loop of DNA and it is associated with topoisomerase and structural maintenance of proteins (SMCs), which are important for maintaining the structure of the chromosomes. 11. Compare and contrast the behaviors of chromosomes in mitosis and meiosis Compare: They are both cell cycles. Mitosis is for growth, repair, and asexual reproduction, and it produces two identical daughter cells that contain the same number of chromosomes as the parent cell. Meiosis is for sexual reproduction. It produces four genetically different daughter cells that contain half the number of chromosomes as the parent cell. During meiosis, modification of histones doesn’t disappear. Instead, they become hereditary information. 12. Describe cohesins and condensins and explain their roles in the cell cycle. They are types of SMC proteins that keep the chromosomes organized during the cell cycle stages. Cohesin's primary role is to connect sitter chromatids together after replication. This is important for chromosomes to separate properly during cell division. Condensins role is to condense chromosomes as they enter mitosis. This is important as it creates positive supercoils, which is when the DNA becomes overwound. Cohesins are added during G1, and they join the two chromatids together. Then, during prophase (the first stage of mitosis), condensins are added to condense and maintain the chromatids. Then, during anaphase, cohesins are removed, the chromatids separate, condensins are removed, and the chromosomes return to their uncondensed state. Chapter 25 1. Summarize the mechanism of DNA replication and why discontinuous synthesis is required. DNA replication is semi-conservative, meaning each DNA strand serves as a template for two new DNA molecules containing a new stand and new strand. DNA replication starts from the origin and proceeds bidirectional. DNA polymerase is synthesized in the 5’ to 3’ direction by adding nucleotides. Discontinuous synthesis is required for the lagging stand that is synthesized in Okazaki fragments (short) that are added in the 5’ to 3’ direction. 2. Describe the Meselson-Stahl Experiment and its importance Bactria cells were grown in heavy nitrogen (15N isotope), then the cells were switched to a 14N medium and allowed to divide, which produced a hybrid DNA containing 15N and 14N. The experiment provided the hypotheses of the semiconservative replication nature of DNA. 3. Define the following terms as they apply to DNA replication: leading strand, lagging strand, continuous 5’-3’ synthesis, discontinuous 5’- 3’ synthesis, Okazaki fragments, DNA polymerase, primase, ligase, and initiator proteins. Leading strand: It is synthesized continuously from 5’ to 3” on the same direction of the replication fork. Lagging strand: It is synthesized discontinuously in short pieces called Okazaki fragments from the 5’ to 3’ in the opposite direction of the replication fork. Continuous 5’-3’ synthesis: Occurs in the leading strand as nucleotides are added to the 3’ in the direction of 5’ to 3’. This occurs in the same direction of the replication fork. Discontinuous 5’- 3’ synthesis: Occurs in the lagging stand as short fragments of nucleotides are added to the 3’ in the direction of 5’ to 3’. This occurs in the opposite direction of the replication fork. Okazaki fragments: DNA strands synthesized in short pieces on the lagging strand. DNA polymerase: Enzymes that add nucleotides using two Mg2+ ions. It has 3 domains (palm, fingers, and thumb) and a pocket with two regions (insertion site and post-insertion site). The insertion site is the region where entering nucleotides bind, and the post-insertion site is the region where the made base pairs stay as the enzyme moves forward. Primase: This strand that is complementary to the template has a free 3’ hydroxyl group to which the DNA polymerase can add nucleotides. Ligase: The 5’ has PO4, which AMP must activate when it is attached. The 3’ has OH as a nucleophile and attacks the phosphate, removing the AMP. Initiator proteins: They are used to initiate the DNA replication process. 4. Describe the primary events of replication occurring in the replication bubble with regard to: direction of synthesis, action of DNA polymerase, requirements of single strand binding proteins, actions of helicase, and action of topoisomerase (plus types of topoisomerases). The direction of DNA synthesis is from 5’ to 3’, in which DNA polymerase adds nucleotides to the 3’. Single-strand binding proteins stabilize the single-stranded DNA after it is unwound by helicase. Helicase is an enzyme that plays a role in unwinding the double helix of the DNA by breaking the hydrogen bonds using ATP. Topoisomerase is an enzyme used to relieve stress that is caused by the unwinding of the DNA helix. 1. Type I topoisomerases cut one DNA strand transiently, using single-strand breaks. 2. Type II topoisomerases transiently cut the two DNA strands using ATP and double-strand breaks. 5. Describe and be able to apply the rules that govern DNA replication. DNA replication is a semi-conservative process. Replication begins with a specific origin. Replication proceeds bidirectionally (5’ to 3’). DNA replication is semi discontinuous. 6. Identify the similarities and differences of DNA replication in Prokaryotes & Eukaryotes. Similarities: 1. Both DNA polymerase has 3’ to 5’ exonuclease activity. 2. Both have primers that are removed by RNase. Differences: Prokaryotes Eukaryotes Occurs in the cytoplasm It occurs in the nucleus. Has DNA polymerase I, II, III, IV (3 Has DNA polymerases ε, δ, and α. (It main types which are III, II, I) has 15) Less complex More complex It does not have cyclin-dependent Regulation is due to cyclins and kinases and cyclins to regulate the cyclin-dependent kinases. cycle. It needs DNA gyrase. It does not need DNA gyrase. Chromosomes are circular Chromosomes are linear Does not have nucleosomes Has histones in nucleosomes Does not have telomeres It has telomeres Has a single point of origin It has multiples points of origin 7. Discuss the different types of DNA polymerase in both Prokaryotes & Eukaryotes. Prokaryotes: 1. DNA polymerase I: Most abundant, not used for replication, but for cleanup. Low processivity. 2. DNA polymerase II, IV, and V are involved in DNA repair. 3. DNA polymerase III: This is the main polymerase in replication. Eukaryotes: 1. DNA polymerase alpha α: Makes RNA primers for the Okazaki fragments. 2. DNA polymerase delta δ: Makes the lagging stand. 3. DNA polymerase epsilon ε: Makes the leading strand and might be involved in repairs. 8. Define the Ames test, mismatches, abnormal bases, pyrimidine dimers, and Backbone lesions. Amest test: It is used to identify a compound's mutagenic potential. It uses mutated the salmonella strain and a sound to see if the compound restores the salmonella function (synthesize His). Mismatches: Occurs from the addition of incorrect nucleotides. Abnormal bases: Occurs from the spontaneous removal of the amino group (deamination), chemical alkylation, or exposure to free radicals. Pyrimidine dimers: Occurs when DNA is exposed to UV light. Backbone lesions: Occurs when DNA is exposed to ionizing radiation and free radicals. 9. Describe the different DNA repair mechanisms and know all enzymes involved in each mechanism. Also, know diseases associated with an inability to repair DNA lesions. Mismatch repair system: This recognizes normal bases that aren’t forming the Waston-Crick base pairs. Cleves non-methylated DNA stand and it is replaced with DNA polymerases III and DNA ligase Base-excision repair: Involves DNA glycosylase enzymes that cleave glycosidic bon between altered base and ribose. They identify DNA lesions and remove the base pairs affected by cleaving the N-glycosyl bond. Nucleotide-excision repair: Involves DNA damage due to UV radiation or carcinogenic substances. It removes bulky DNA lesions and pyrimidine dimers, and DNA polymerase I and DNA ligase are used to replace the DNA and close the gap. Transcription-coupled repair: During transcription, when the RNA polymerase stops when it finds a region damaged in the DNA template, the excision proteins repair the damaged region so that the RNA polymerase can continue. Direct repair: Not found in mammals, as photolyase enzymes use light energy to repair pyrimidine dimers. Unrepaired DNA can cause cancer and genetic diseases. 1. Accelerated aging and retarded growth (Werner syndrome): Hyper combination, defective DNA initiation, and elongation caused by mutated WRN gene. 10. Summarize why mutations in DNA repair systems can lead to genetic diseases, including certain types of cancer. Mutations in the DNA repair system mean that any damaged DNA won't be effectively repaired, which can lead to genetic disease and cancer. Xeroderma pigmentosum (mutated nucleotide-excision repair). 11. Describe the damage to DNA caused by UV light and how it is recognized by the repair enzymes, and the mechanisms to repair the damage. UV light forms pyrimidine dimers, and they are repaired with nucleotide excision repair. The repair systems find the damaged region, cut the DNA strand, remove 12 nucleotides, and use DNA polymerase and ligase to fill the gap. 12. Describe the different mechanisms of DNA recombination and genetic rearrangements. Homologous recombination: It involves genetic exchange with two DNAs that have identical sequences. It occurs in meiosis during crossing over. It yields genetic diversity in eukaryotes and repair in bacteria. Site-specific recombination: It involves genetic exchange only at a particular sequence of DNA. Combining gene clusters, trans lesion polymerase, and NHEJ produces antibodies in B cells. DNA transposition: It involves get big engine in which a short segment of DNA moves from one chromosome to another. 13. Describe the double-stranded, helical, and antiparallel chain structure of DNA and how it relates to the processes of DNA replication, recombination and repair. DNA consists of two double statins that are twisted out from a helix. The two strands run in the opposite direction, which is important for base pairs during replication, replication, and repair. Chapter 26 1. Summarize the central dogma of molecular biology, & cite exceptions to the model It states that genetic information in DNA is replicated and transcribed into RNA, then translated into proteins. Exceptions include: a) Reverse transcription: RNA is made from DNA. b) RNA virus. c) Prions d) RNA editing 2. Define the following terms as they apply to RNA synthesis (transcription): template strand, coding strand, promoter sequence, primary transcript (hnRNA), upstream/downstream of transcription initiation site. Template strand: Is it used as a template for RNA polymerase to act on. Coding strand: It is a non-template stand with the same base sequence as the RNA transcribed, where T is replaced with U. Promoter sequence: It is the DNA sequence that RNA polymerases bind to direct the direction of transcription. Primary transcript (hm RNA): RNA molecules that are newly synthesized. Upstream/ downstream of transcription: Upstreams are located at the beginning of transcription, and they contain regulatory proteins. Downstream is located after the start of transcription. 3. Discern the coding strand from the template strand and be able to recognize the mRNA species that is derived from a given dsDNA molecule. Also, be able to deduce the DNA species from which a given mRNA was derived. The coding strand moves from a 5’ to 3’ direction, and it is a non-template stand with the same base sequence as the mRNA, where T is replaced with U. The template strand moves into 3’to 5’ direction, but the RNA polymerase synthesizes the mRNA in the 5’ to 3’ direction. 4. Identify the function of the following transcription signals: proximal and distal elements, cis-acting elements, trans-acting factors, enhancer and silencer elements. Plus compare each in prokaryotic and eukaryotic transcription. Proximal and distal elements: Proximal elements are transcription signals found at the start of transcription. Distal elements are found in the downstream. Cis-acting elements: Serves as a binding site for promoters, enhancers, and silencers. They are located in the same DNA molecule they regulate. Trans-acting elements: Bind to the cis-acting elements to regulate gene expression. They regulate genes that are found in different DNA molecules. Enhancer and silencer elements: Enhancer elements increase the rate of transcription, while silencer decreases the rate of transcription. 5. Compare and contrast the different eukaryotic RNA polymerases Compare: they all have a regulation mechanism. Contrast: RNA pol 1 is responsible for synthesizing pre-ribosomal RNA, and RNA pol II is for making mRNA as they are fast and can recognize a lot of promoters, but they are inhibited by α-amanitin (mushroom toxin). RNA pol III is responsible for making tRNAs and small products of RNA. Plants have RNA pol IV used to synthesize small interfering RNAs (siRNAs), and the mitochondria have RNA polymerase. 6. Describe the events that occur during the four steps leading to mature mRNA: synthesis of hnRNA; 5’-capping; poly-A tail addition; and intron removal by the spliceosome. Synthesis of hnRNA: Also called pre-mRNA. They are transcribed in the nucleus by pol II. 5’-capping: 7-methyguasonine is added on the 5’ end of RNA to protect from ribonuclease (endonuclease) which degrades the RNA. Poly-A tail addition: Poly-adenylation is added to the 3’end to make mRNA stable. Intron removal by spliceosome: Introns are removed from mRNA by spliceosome (splices mRNA). 7. Identify the four classes of introns and how each one works. Group I, group II, Spliceosomal introns, and tRNA introns (protein-catalyzed) Group I and II introns splice on their own without using proteins or ATP. They are found in the genomes of nuclear, mitochondrial, and chloroplast that encode for mRNA, rRNA, and tRNA Spliceosomal introns are the most common introns, and spliceosomes splice them. proteins catalyze tRNA introns and uses ligase and splicing endonuclease. 8. Discuss the different classes of pre-mRNA splicing defects that cause disease as well as splicing related diseases to each splicing defect. Alternative splicing: Defect can cause spinal muscle atrophy. 9. Identify the function of eukaryotic pre-mRNA, mature mRNA, pre-rRNA, rRNA, pre- tRNA, and tRNA plus know where each originates in the cellular organelles. Also, know the different steps in maturation of mRNA, rRNA, and tRNA. mRNA, messenger RNA I. Pre-mRNA: Made in the nucleus via RNA pol II. It has exons and introns to make mature mRNA. II. Mature mRNA: made from pre-mRNA in the nucleus and moved to the cytoplasm to serve as a template for the synthesis of protein. III. Maturation steps: Adding 5’cap, adding poly A-tail, and splicing of introns to join exons. rRNA, ribosomal RNA I. Pre-rRNA: Contains 18S, 5.8s and 28S rRNAs. Used to make ribosomal RNA. Made via RNA pol I. II. rRNA: Made from ribosomes and synthesizes proteins. tRNA, transfer RNA I. Pre-tRNA: Made by RNA pol II. Used to make tRNA. II. tRNA: made from pre-tRNA in the nucleus and moved to the cytoplasm to transport AA to ribosomes. Maturation steps: Adding 5’cap, adding poly A-tail, and splicing of introns to join exons. 10. Identify base modification in tRNA and rRNA & explain the role of each tRNA and rRNA are modified in post-transcriptional reactions: Pseudouridine, Thiouridine, and Dihydrouridine. 11. Define miRNA & RNAi and describe its role in regulation of gene expression MicroRNAs (miRNAs): These are short, noncoding nucleotides that have approximately 22 nucleotides. Binds to specific regions of mRNA in order to affect translation by cleaving or blocking (from translation) the mRNA. They are synthesized from endoribonucleases Drosha and Dicer. RNA interference (RNAi): is the mechanism for processing miRNAs to silence the gene expressions. 12. Describe Ribozymes, Retroviruses, and Reverse Transcriptase plus drugs that target each of them Ribozymes: These RNA molecules catalytically cleave themselves or other RNA. Examples include self-splicing group I introns, RNase P (cuts the precursor to tRNAs ), and Hammerhead ribozyme (cuts RNA of circular RNAs called virusoids) Retroviruses: RNA viruses that have the ability to convert RNA to DNA via reverse transcriptase. Drugs: protease inhibitors (Indinavir, Saquinavir). Reverse Transcriptase: an enzyme that converts RNA to DNA in retroviruses. Drugs: reverse transcriptase inhibitors (Zidovudine, Didanosine) 13. Discuss Telomeres & Telomerases plus the mechanism of the enzyme and how it relates to aging Telomeres are a region that ends the eukaryotic chromosome, and telomerase extends the ends of the chromosome. Suing the RNA of the enzyme as the primer, telomerase extends the 3’ end, and DNA polymerases fill the gap on the other stand. Telomerase serves as a reverse transcriptase that has an internal RNA template to end the telomeres. Telomeres shorten as the cell divides, and at a very short length, they undergo apoptosis, which catalyzes aging. Chapter 27 1. Describe the universal flow of genetic information from DNA -> RNA -> Protein (and the exception to this rule) It states that genetic information in DNA is replicated and transcribed into RNA, then translated into proteins. Exceptions include reverse transcription, RNA virus, Prions, and RNA editing. 1. Identify that the genetic code is degenerate, unambiguous, nonoverlapping and almost always universal Degenerate: When multiple codons can code for the same amino acids. Unambiguous: When a specific codon only codes for a specific amino acid Nonoverlapping: When each nucleotide base is only part of that one codon. It doesn’t share nucleotides. Universal: Codons that are the same code for most species. 2. Describe the interaction of codons and anticodons plus know their relationship to mRNA and tRNA Codon sequence consists of three nucleotides on the mRNA, which is complementary to the anticodon sequence that contains three nucleotides on the tRNA. It is paired via hydrogen bonds in which the two RNAs are aligned antiparallel to one another. 3. Describe the different types of mutations that can occur in both RNA & DNA plus diseases related to both Large segment deletions remove a whole gene which makes it nonfunctional, seen in α-thalassemia. Mutations in splice sites: Changes the number of nucleotides in a splicing site, leading to β- thalassemia, Tay-Sachs disease, and Gaucher disease. Triplet repeats expansion: An increase in the number of triplets in the gene, which makes it longer than normal and produces an unstable protein. If it occurs in the coding region it leads to Huntington disease (CAG repeat in huntingtin, HTT gene), spinobulbar muscular atrophy (CAG repeat). If it occurs in the non-coding region it leads to Fragile X syndrome (CGG repeat), myotonic dystrophy (CUG repeat). Point mutation: I. Silent mutations: This is when one of the nucleotides in the codon has changed, but it still codes for the same amino acid. It does not affect the function of the protein. a. Conservative substitution: This is when a mutation in the first nucleotide of the codon changes the amino acid but has similar functions. II. Missense: The changed codon species for a different amino acid which affects the function. Seen in sickle cell anemia (GAG to GTG) III. Nonsense: the changed codon sequence stops the codon and its nonfunctional. IV. Frameshift: The deletion or addition of a nucleotide on a codon which makes it nonfunctional 4. Describe the three phases of translation: i) Initiation ii) Elongation iii) Termination. Initiation: Firstly, the tRNA is amino acylated to activate it and then in the. initiation phase, mRNA and the activated tRNA is bind to a small ribosome, followed by binding to a larger ribosome subunit. Elongation phase: Cycles of aminoacyl and tRNA binding allows for peptide bond formation to occur until the ribosome reaches a stop codon. Termination: Once the stop codon is recognized, translation stops. Then, the mRNA and protein leaves and the ribosomal subunits are recycled. 5. Describe formation of aminoacyl-tRNA as well as peptide formation Aminoacyl- tRNA formation: Amino acid is activated via aminoacyl tRNA synthase with ATP to yield Amino acyl-AMP. The enzyme then transfers the amino acid that is activated to the 3’ end of the tRNA to form an ester bond which links them forming Aminoacyl-tRNA. Each enzyme is specific for one amino acid. Peptide formation: Aminoacyl-tRNA enters the A site of the ribosome and formation peptide bond with the Met on the tRNA on the P site. The growing peptide chain is transferred to the A site. Peptidytransferase a rRNA catalyzes the formation of each peptide bond 6. List the posttranslational modifications that occur on select amino acids Acetylation: adding an acyl group to lysine in on the N-terminal amino acids to change the charge from positive to neutral. This is the on switch for other reactions. Methylation: Adding methyl group to lysine to change the charge from neutral to positive. This is the off switch for other reactions. ADP-ribosylation: Adding ADP-ribose to arginine, glutamate, and aspartate. Carboxylation: Adding a carboxylic group to glutamate which is important for blood coagulation. Fatty acylation: Adding fatty acid to cysteine, lysine, and glycine. Glycosylation: adding a carbohydrate group to the nitrogen of arginine or asparagine, or adding it to the oxygen of serine, threonine, or tyrosine. Prenylation: Adding hydrophobic molecules to protein for protein-protein interactions. Hydroxylation: Modifies proline and lysine for stabilization. Phosphorylation: Adding or removing phosphate group from serine, tyrosine, or threonine. 7. Discuss eukaryotic ribosome synthesis and ribosomal assembly plus know the processes involved in each and the diseases related misassembly. Ribosome synthesis begins in the nucleolus, where pre-rRNA is transcribed from rDNA via RNA polymerase I. Mature rRNAs are produced via a series of cleaves in the 45S transcript using processing factors that are found in the nucleus and cytoplasm. Assembly a) rRNA from the nucleolus, 5S rRNA from the nucleus, recycled ribosomal proteins from the cytoplasm and assembly factors are assemble into ribosomal subunits. b) Via diffusion ribosomal subunits move from the nucleus to the cytoplasm, where they will remain until protein synthesis. Diseases include Treacher Collins syndrome, Dyskeratosis congenital, Cartilage hair hypoplasia, Diamond blackfin anemia Sq-Syndrome, and Schwachman Diamond syndrome. 8. Describe events of proteins synthesized on RER and polysomes Protein synthesis in RER a) Step 1.Translation of the protein starts in the cytosol as ribosomes are being attached to the mRNA. A signal sequence/peptide attached to the protein direct it to the RER. b) Step 2. A signal recognition particle (SRP) binds to the signal peptide and prevents further synthesis of the protein. c) Step 3. SRP bind to the SPR receptor found in the RER membrane, which attaches (docks) the ribosome to the RER. d) Step4. SRP is released, and the synthesis of proteins resumes. e) Step 5. Signal peptidase removes signal peptide in the RER, f) Step 6. Nascent protein chains continue its elongation/synthesis, and then the completed protein is released into the lumen of the RER. g) The synthesized proteins (from ribosomes attached to the ER) travel in vesicles to the cis-Golgi bodies to which they are transported to lysosomes, cell membrane, or cell secretion. Protein synthesized from cytosolic ribosomes are released to the cytosol or transported to the mitochondria and nucleus. A polysomes are made of mRNA joined with two or more ribosomes. a) Multiple ribosomes attached to a single mRNA in which each ribosome synthesize its own protein, and proteins are released after translation is completed. 9. Describe the events in protein targeting and how defects in phosphotransferase activity can lead to I-cell disease Signal sequences direct the synthesized protein to its destination. a) Step 1. Protein targeting starts in the initiation phases of protein synthesis attached to a ribosome. b) Step 2. The signal sequence is synthesized at or near the N-terminus. c) Step 3. The signal sequence and the ribosome are joined to a large signal recognition particle (SPR). Then the SPR binds to GTP which stops polypeptide elongation when it is 70 amino acids long. d) Step 4. The GTP-bound SRP directs the ribosomes and the incomplete polypeptide to GTP-bound SRP receptors in the surface of the ER. Then the polypeptide is delivered to a peptide translocation complex in the ER as it directly interacts with the ribosome. e) Step 5. The SPR leaves the ribosome, and hydrolysis of GTP occurs in the SPR and SPR receptors. f) Step 6. Elongation of the polypeptide continues via ATP by the translocation complex, adding to the growing polypeptide into the lumen of the ER until the protein is synthesized. g) Step 7. The signal sequence is removed via signal peptidase in the ER lumen. h) Step 8. The ribosome leaves. i) Step 9. The ribosome is recycled. I-cell disease is a protein-targeting disorder. Defects in the activity of phosphotransferase prevent the proper tagging of lysosomal enzymes which they are secreted outside the cell inside of the lysosome. This leads to the accumulation of undigested materials. A deficiency of the enzyme GlcNAC phosphotransferase causes this. 10. Clinical correlations: Identify the site of action of the following antibiotics that selectively inhibit bacterial protein synthesis: Streptomycin, tetracycline, chloramphenicol, and erythromycin. Streptomycin: Bind to 30s subunit causes codes to be misread in bacteria in low concentrations of it, and in higher concentrations, it inhibits protein synthesis. This prevents initiation. Tetracycline: Blocks the A site on the ribosome to prevent aminoacyl-tRNAs from binding, which inhibits protein synthesis. Binds to 30s ribosomal subunit to inhibit elongation. Chloramphenicol: Binds to 50s ribosomal subunit blocks peptidyl transfer to inhibit protein synthesis in mitochondria, chloroplast, bacteria, but not in eukaryotes. Erythromycin: Binds to 50s ribosomal subunit to inhibit translocation. Chapter 28 1. Identify the mechanisms for regulating gene expression in both prokaryotes & in eukaryotes. Prokaryotes: RNA polymerase access to promoters in which they can bind and initiate transcription in the absence of activators or repressors. Eukaryotes: a) Chromatin hinders promoters, and when transcription is activated, structural changes occur it the chromatin. ▪ Euchromatin is less condensed chromatin that is most time transcriptionally active. In contrast, heterochromatin associated with centromeres are transcriptionally inactive. b) Presence of positive (important) and negative regulatory mechanisms. c) Long non-coding RNAs (lnc RNAs) are common and regulated via hormone receptors. d) Has a larger and complex regulatory protein e) Transcription in nucleus occurs separately from translation in the cytoplasm. 2. Understand and know regulation of operons Operons are clusters of genes that share a promoter and regulatory sequences. They rely on repressors (deactivate gene expression) and inductors (activate gene expression). For the Lactose (lac) operon to be inducted, high lactose must be present to form allolactose, which binds to the repressor and decreases repression (activates transcription). Also, glucose must be low to increase cAMP and allow CRP to bind to the promoter to cause the activation of transcription. Lactose (lac) operon has: a) β-galactosidase (lacZ) cleaves lactose to produce glucose and galactose b) Lactose permease/ galactosidase permease (lacY) transports lactose into the cell. c) Thiogalactoside transacetylase (LacA) 3. Describe the following transcriptional regulatory process in prokaryotes: Induction; Repression; Positive Control; Catabolic Repression; Attenuation Induction: A process when gene expression increases in response to a change in a regulatory protein. The gene products that increase in concentration are called inducible. Repression: A process when gene expression decreases in response to a change in a regulatory protein. The gene products that decrease in concentration are called repressible. Positive Control: It induces transcription though protein activators. Transcription is induced when signals allow for activators to bind to DNA. Catabolite repression: Prevents the expression of genes that are needed for the catabolism of sugar in glucose (lactose, arabinose, etc.) even in the presence of secondary sugars. In the presence of glucose, genes for lactose are turned off. It is mediated by cAMP and cAMP receptor protein(CRP) Attenuation: Depends on the availability of tryptophan, where transcription starts and stops before operon genes are transcribed. a) High Trp means that tRNATrp is high, which leads to attenuation. Trp binds to the repressor, which then binds to the operator, which slows transcription. Segment 1 is transcribed too fast, and Segment 2 is coated with ribosomes, which prevents it from pairing with Segment 3. Then, segment 3 pairs with segment 4 on the mRNA and forms a hairpin structure, which is the attenuation signal and stops transcription. b) Low Trp means tRNATrp is low. The repressors do not bind to operator because of scarce Trp, which allows for transcription to proceed. The ribosome will halt at the Trp codons in segment 1, which allows for segment 2 and 3 to pair which allows for transcription. 4. List the difference in genomic construction between prokaryotes & eukaryotes Prokaryotes: Circular chromosomes, less complex, one chromosome, lack introns, have exons, lack histones, and have operons. Eukaryotes: Linear chromosomes, more complex, 23 chromosomes, have introns/exons, have histones, and have positive/ negative regulators. 5. Describe the following regulatory processes in eukaryotes and know examples of Gene Alteration, Transcriptional Control, Posttranscriptional Control, Translational Control, and Posttranslational Control. Gene alteration: These are changes made in the gene sequence that affects the genes expressed. Transcriptional control: Regulates expression of genes during transcription. Posttranscriptional control: The expression of genes is regulated after transcription before translation. This includes RNA splicing, alternative splicing, and RNA editing. Translational control: Regulates the expression of genes during translation. Posttranslational control: Newly translated/ synthesized proteins from the mRNA are modified via enzymes to their active conformation. 6. Describe the regulation of the SOS response and RNA-mediated regulation. SOS response is when an excessive damage in the chromosome of bacteria triggers an induction of genes involved in stopping DNA replication and repair. Rec A proteins and Lex A repressor are the regulatory proteins. Damaged DNA produces a lot of single-stranded DNA in which RecA (co-protease) binds to it to facilitate cleavage and inactivate Lex A repressor. The inactivated Lex A repressor allows for SOS genes and RecA to repair the damage. RNA-mediated regulation: When bacteria require proteins for cell growth, they produce more ribosomes (r-protein). The genes that encode for these r-proteins are in operons (r-operons), which are regulated via translational feedback. In translational feedback, each operon for one r-protein encodes a translational repressor that bids to mRNA, which blocks translation. This repressor has a higher binding affinity to rRNA than to its mRNA, which represses translation when the level of r-protein is more than the levels of rRNA. 7. Discuss Eukaryotic gene regulation and Hormonal regulation of transcription. Eukaryotic gene regulation needs the remodeling of chromatin, activators, coactivators, architectural regulators, and transcriptional factors. a) Histones are covalently modified through methylation (activates transcription), phosphorylation., acetylation (decreases histones affinity for DNA), ubiquitination, and SUMOlation. Hormonal regulation of transcription is when hormones bind to receptors, and via HREs, they affect transcription. It is also regulated by peptide hormones, which activate a second messenger to activate transcriptional factors. a) Type I hormone receptor is a nuclear receptor of sex hormones and glucocorticoids. It is found in the nucleus, and once the hormone is bound to the receptor, it moves to the nucleus to be a transcriptional activator. b) Type II hormone receptors are thyroid hormone receptors that are found in the nucleus, and once the receptor is bound to the hormones, it activates transcription. 8. Explain the roles of the Iron Responsive Element (IRE) and the Iron Responsive Factor (IRF) in regulating the synthesis of transferrin receptors and ferritin. Predict the consequences of iron deficiency and hemochromatosis on synthesizing transferrin receptor and ferritin. Iron regulatory protein/ iron responsive factor binds to sections in the mRNAs that encode for ferritin and transferrin receptors. IRE are hairpin structures found on the ends of mRNA (5’ for ferritin and 3’ for transferrin receptor), which are the sections IRF binds to. When iron is low, IRP binds to IRE, which stabilizes the mRNA and increases the synthesis of transferrin, blocks translation, and decreases the synthesis of ferritin. When iron is high, IRP does not bind to IRE, which degrades the mRNA and decreases the synthesis of transferrin, blocks translation, and increases the synthesis of ferritin. Iron deficiency leads to increased iron uptake and decreased storage of iron. Iron regulatory proteins increasingly bind to iron-responsive elements, to which the synthesis of transferrin is increased, which leads to decreased synthesis of ferritin. Hemochromatosis (too much iron) leads to decreased iron uptake and increased storage of iron. Iron regulatory proteins do not bind to iron-responsive elements, which decreases the synthesis of transferrin, which leads to increased synthesis of ferritin. 9. Discuss the role of stem cells in regulation of gene expression. Stem cells can differentiate into various tissues, and their main function is to replenish themselves and/or differentiate, which is regulated by signals. Embryonic stem cells are pluripotent, meaning they can differentiate into many tissues used for medical research. 10. Clinical Correlation: describe the mechanism of drug resistance with the use of methotrexate. Cancer cells are rapidly undergoing DNA/RNA synthesis and diving quickly. Methotrexate inhibits dihydrofolate reductase, which prevents it from producing an active form of folic acid (tetrahydrofolate), which is required for purine and pyrimidine synthesis for DNA/RNA synthesis. This kills the cancer cells. 11. Clinical Correlation: describe the two mechanisms of action by which interferon inhibits gene expression. Interferons are signaling proteins released in the presence of viruses. a) They activate protein kinases to prevent viral proteins from synthesizing, which stops virus replication. b) They can activate ribonuclease to break down the RNA (rRNA and tRNA) of the virus, which stops viral replication by reducing the availability of ribosomes needed for protein synthesis.