DM308 Production Techniques 2 Lecture 6 PDF
Document Details
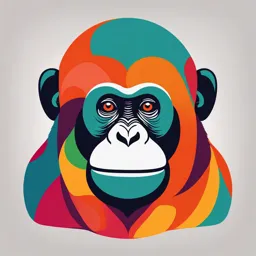
Uploaded by SafeDiscernment
University of Strathclyde
Dr. Vassili Vorontsov
Tags
Summary
This document provides a lecture on aluminium alloys and their processing, including topics such as property control, element abundance, energy consumption, recycling, uses, and various alloying methods and treatments. It also discusses different types of casting methods.
Full Transcript
DM308 Production Techniques 2 Lecture 6 – Aluminium alloys and their processing Dr. Vassili Vorontsov Department of Design, Manufacture and Engineering management, Faculty of Engineering, University of Strathclyde [email protected] Property control in Al alloys Element no. 13 abundance...
DM308 Production Techniques 2 Lecture 6 – Aluminium alloys and their processing Dr. Vassili Vorontsov Department of Design, Manufacture and Engineering management, Faculty of Engineering, University of Strathclyde [email protected] Property control in Al alloys Element no. 13 abundance Aluminium is the third most abundant element in the Earth’s crust. Aluminium alloys are the second most widely used alloy system today. Oxygen 46.60% Silicon 27.72% Aluminium 8.13% Iron 5.00% Calcium 3.63% Sodium 2.83% Potassium 2.59% Magnesium 2.09% Data: USGS Report 1953 Energy consumption to process Al Importance of recycling Given the high cost of aluminium reduction, large scale recycling is attractive from a commercial standpoint. The cost of recycling aluminium is only ≈5% of the cost of extracting aluminium from ore. Recycled aluminium forms 1/3 of the global Al consumption and 1/2 of Al consumption in the European Union. Demand for recycled aluminium exceeds supply. Uses of aluminium alloys Electrical systems Transportation White goods Machinery Construction Food packaging What can we alloy Al with? Most metals can alloy with aluminium. Not many have sufficient solid solubility in Al to be major alloying additions. Element Temperature (ºC) Zinc Maximum solid solubility (wt.%) (at.%) 443 82.8 66.4 Silver 566 55.6 23.8 Magnesium 450 17.4 18.5 Lithium 600 4.2 16.3 Germanium 424 7.2 2.7 Copper 548 5.65 2.40 Silicon 577 1.65 1.59 Manganese 658 1.82 0.90 Titanium 665 1.3 0.74 Chromium 661 0.77 0.40 Solid solution strengthening of Al Annealed high purity Al has a very low yield strength of 7-11MPa. Manganese and Copper have a strong solid solution hardening effect, but start to precipitate intermetallic phases above 0.2-0.3%. Magnesium is the best solid solution strengthening element on a relative weight basis, with high solid solubility. (175MPa yield with 6% Mg) Zinc gives little solution strengthening, despite high solubility in Al. Work hardening and annealing Al alloys fall into two groups: 1). Mechanical properties are controlled by work hardening 2). Mechanical properties are controlled by annealing e.g. cold rolled alloy N.B. White blobs show nucleation sites of recrystallized grains. deformation/transition band shear band e.g. cold worked alloy with intermetallic particles Work hardening of Al Deformation structures in strain hardened Alloy 6060 - AlMgSi0.5 Work hardening characteristics Introducing strain by coldforming (such as coldrolling) can substantially increase the strength of an aluminium alloy. Here we see increases in both in the UTS (top) and the 0.2% yield stress (middle) as a function of the amount of strain introduced by cold rolling (bottom). Temperature and work hardening Cold working temperature has a very pronounced effect on the resulting strength. At greater temperatures the rate of dynamic recovery processes is higher. This means dislocations are either annihilated or rearranged to form a cellular structure. Cryogenic deformation slows dynamic recovery allowing a greater increase in strength at the expense of ductility. Data shown for alloy 1100 Alloying and work-hardening Alloying can also be used to improve strain hardening. Here the solution hardening effect of Mg is also exploited to slow down the rate of dynamic recovery compared to pure Al. If a cellular structure is desired in Al-5%Mg the alloy would have to be worked at a higher temperature. E.g. hot rolling. Annealing of Al alloys The pre deformation properties can be partially or fully restored by annealing at elevated temperature. Below: annealing behaviour of commercially pure Al. Age hardening 1. Solution heat treatment at high temperature in single phase region. 2. Rapid quenching to obtain a supersaturated solid solution (SSSS). 3. Controlled decomposition of SSSS to form finely dispersed precipitates by heat treatment in the multiphase region at intermediate temperatures 1 3 2 Precipitation in the Al-Cu system GP Zones in Al-2.5%Cu SSSS decomposition occurs in stages, via formation of metastable intermediate precipitates. Initially small clusters of atoms may form and act as nuclei for Guinier-Preston (GP) zones. GP zones are ordered solute-rich groups of atoms 1or 2 atom planes in thickness. They are coherent with the matrix, but usually have a coherency strain (lattice misfit). Precipitation in the Al-Cu system Eventually much larger intermediate precipitates form that have a more defined chemical composition. Such precipitates are usually only partly coherent with the matrix. Precipitates can nucleate from or at the site of a GP zone. However heterogeneous nucleation can also take place at lattice defects such as dislocations. Al2Cu Maximum hardening requires an optimum dispersion of precipitates. (i.e. size + volume fraction) Precipitate-free zones No PFZ PFZ PFZ in Al-4Zn-3Mg Al-4Zn-3Mg + 0.3% silver All precipitation hardened alloys can form precipitate depleted zones adjacent to the grain boundaries. Solute diffusion is faster at the grain boundaries and GBs are also good heterogeneous nucleation sites for precipitates. Solute atoms are therefore leached from the matrix surrounding the grain boundaries as they diffuse to form precipitates there. PFZ are thus devoid of strengthening precipitates and act as stress concentrators. The width of the PFZ can be controlled through heat treatment and alloying. Combining ageing and deformation Al-5.3Cu-1.3Li-0.4Mg-0.4Ag-0.16Zr without (left) and with 6% cold work (right) prior to the ageing heat treatment. The microstructure produced during ageing can be controlled by employing cold-work in conjunction with the heat treatment. This allows a coarser but more uniform precipitate distribution. Corrosion of Al Aluminium is a very reactive metal, but it is quite resistant to corrosion because it is covered in a protective oxide film. However, when in contact with an electrolyte and a less reactive metal it is highly susceptible to galvanic corrosion. The aluminium acts as a sacrificial anode and can corrode quite rapidly. Certain alloying additions can adversely affect the corrosion resistance. Al-Cu alloys have poor corrosion resistance since copper is less reactive than aluminium. (Cu has the worst effect of all alloying elements.) Single-phase solid solutions are the most corrosion-resistant form of alloys. They are less susceptible to localised attack (pitting) than multi-phase alloys. Anodising Aluminium can be further protected from corrosion by anodising. Anodising increases the thickness of the protective oxide film. The aluminium is used as an anode in an acid electrolyte bath. Application of a direct electric current breaks down the water in the electrolyte down releasing oxygen. The oxygen reacts with the anode surface to form Al a porous oxide. The very regular pore structure readily accepts dyes. Wrought Al alloys Wrought aluminium products Sheet/Plate Foil Wire Tube/Rods 75-80% of aluminium is used for wrought products These products are produced from cast ingots Complex Extrusions The structures of the ingots are altered significantly by various mechanical working processes and heat treatments. Vertical direct chill (DC) casting Vertical DC casting is the most commonly used process for ingot production Molten alloy is poured into one or more fixed water cooled moulds with a retractable base. Solidification is a two stage process: (1) solidification of alloy adjacent to chilled mould wall, (2) solidification of the remainder during submould heat removal by spray cooling. Vertical DC casting is a semicontinuous process capable of producing ingots up to 15 tonnes in mass. Problems with DC casting The problem with DC casting methods is the rippled surface finish they produce. This occurs due to stick-slip contact as they move past the sides of the mould when solidification first occurs This can lead to surface tears and microstructural inhomogeneities such as inverse segregation in the surface regions. The surfaces of DC ingots therefore have to be machined or scalped prior to rolling or extrusion, which adds to the cost. Improving DC ingot quality The surface quality can be improved by reducing the rate of heat transfer from solidifying ingot to the mould. One method is to direct high pressure air along the metal/mould interface. Alternatively an electromagnetic casting mould where a water-cooled induction coil repels the liquid metal from the mould. Thus metal solidification only occurs when the metal is leaving the mould to be spray cooled. This not only improves the surface finish dramatically, but also refines the grain structure of the ingot. Eliminating the need for scalping. A smaller grain structure is a result of: (1) fast cooling provided by direct spray cooling, (2) convection currents induced in the liquid metal by the electromagnetic field. Horizontal DC casting Horizontal DC has a number of advantages over vertical methods: All machines are on the same level – lower capital investment costs Better conjunction with other manufacturing processes (e.g. raw material feeding, rolling, heat treatment, product storage) More convenient operation for the personnel Graphite moulds: low wettability, high CTE, high thermal conductivity, high thermal shock resistance, self-lubricating, easily machinable Horizontal DC casting + Travelling mould casting Single-roll caster Twin-roll caster Twin-belt caster Travelling moulds have revolutionized casting of lower strength Al alloys. Mould is “endless” – i.e. zero relative movement between the mould and casting surfaces. Castings have a surface with a low defect density. This allows casting of thin rod, slab and sheet. The castings may be coiled and/or subjected to further processing (e.g. rolling) without the need for surface machining. Travelling mould casters Principal alloy families Al Mn Al-Mn Al-Mg-Mn Si Al-Mg Non age hardening alloys Zn Al-Zn-Cu-Mg Cu Mg Al-Zn-Mg Al-Mg-Si Age hardening alloys Al-Cu-Mg Alloy nomenclature e.g. Al 7075 T6 Temper designations 4 digit designation Major alloying elements 1xxx 99.00% Al min. F – as fabricated 2xxx Cu O – annealed wrought products 3xxx Mn 4xxx Si 5xxx Mg 6xxx Mg,Si 7xxx Zn 8xxx Others 1. First digit defines alloy group 2. Second digit indicates modifications to the alloy 3. Last two digits identify the aluminium alloy or indicate the aluminium purity Suffix letter 1st suffix digit H – cold worked (strain hardened) 1 – cold worked only 2 – cold worked & partially annealed 3 – cold worked & stabilised T – heat treated (stable) 1 – partial solution + natural ageing 2 – annealed cast only 3 – solution + cold work 4 – solution + natural ageing 5 – artificial ageing only 6 – solution + artificial ageing 7 – solution + stabilising 8 – solution + cold work + artificial ageing 9 – solution + artificial ageing + cold work 2nd suffix digit 2 – ¼ hard 4 – ½ hard 6 – ¾ hard 8 – hard 9 – extra hard Non heat-treatable alloys The NHT family encompasses wrought compositions that do not respond to strengthening by heat treatment. They comprise of three chief sub-groups: pure aluminium grades, alloys with Mn or Mg as the main alloying element. Approximately 95% or rolled aluminium products are made from these alloys belong to the above groups. Major uses of NHT alloy sheet are: packaging, construction and transportation Selection criteria: (1) structural capability, (2) formability and (3) surface quality. 1xxx series Electrical conductors Lithographic plates This group includes super-purity (SP) aluminium (99.99%) It also includes commercial-purity (CP) grades CP grades contain up to (1%) impurities or alloying additions Tensile properties are low (e.g. 7-11MPa proof stress in SP aluminium) Chief uses: electrical conductors (best electrical conductivity) Other uses: chemical process equipment, foil, decorative architectural products and lithographic plates (i.e. good surface finish and no preferential chemical attack on secondary phases) 3xxx series - Al-Mn and Al-Mn-Mg 3003 – foil, cooking utensils, roofing sheet 3004 – beverage cans Moderate strength (3003 - 110 MPa, 3004 – 180MPa) High ductility Excellent corrosion resistance Mn forms fine Al6Mn particles that contribute to strengthening Mg added as a solid solution strengthener 5xxx series - Al-Mg Pressure vessels Boat hulls Liquid transport tanks 5xxx alloys comprise the bulk of all Al sheet products 0.8-5% Mg can be used - good solid solution strengthening A range of strengths (e.g. 40MPa yield in 5005 vs. 500MPa in 5456 H19) The softer alloys usually exhibit good ductility (up to 25% elongation) Good corrosion resistance Uses: welded applications (liquid transport tanks, pressure vessels, boat hulls, armour) Heat treatable alloys phase precipitates in Al-4wt%Cu Cu containing precipitates in Al-Mg-Si-Cu These are wrought alloys that respond to strengthening by heat treatment. They rely on precipitation of secondary and tertiary intermetallic phases in the alpha solid solution matrix phase. There are three series: 1. 2xxx (Al-Cu and Al-Cu-Mg) 2. 6xxx (Al-Si-Mg) 3. 7xxx (Al-Zn-Mg and Al-Zn-Mg-Cu) The alloys can also be divided into two groups based on properties: 1. Medium strength weldable (Al-Mg-Si and Al-Zn-Mg) 2. High strength with limited weldability (Al-Cu, Al-Cu-Mg and Al-Zn-Mg-Cu) 2xxx series - Al-Cu and Al-Cu-Mg Al-Cu-Mg alloys like Duralumin (Al-3.5Cu-0.5Mg-0,5Mn) have been widely used by the aircraft industry since the era of the Zeppelin airships, where they were used for the rigid airframe. Their use quickly spread to aeroplanes. Today Duralumin has been superseded by newer derivative systems like 2014 and 2024 with 0.2% proof stresses as high as 320 and 490MPa respectively. However, a close derivative of Duralumin, 2017, is still used in aircraft rivets. 2xxx alloys are typically produced as sheet roll clad with Al-1Zn for corrosion resistance In addition to high strength, 2xxx also possess good creep resistance for Al alloys. This led to development alloys like 2618 (Al-2.2Cu-1.5Mg-1Ni-1Fe) for supersonic aircraft. 6xxx series - Al-Mg-Si Medium strength Al-Mg-Si alloys are used for the majority of Al extrusions Good weldability Corrosion resistance Immunity to stress corrosion cracking The strength is proportional to Mg and Si content Precipitation processes in Al-Mg-Si alloys are recognised as the most complex. Clustering of silicon atoms can occur before GP zone formation. Precipitates that nucleate on these clusters tend to be coarser which adversely affects strength. Therefore, it is important to control the clustering process by alloying. 7xxx series - Al-Zn-Mg & Al-Zn-Mg-Cu The Al-Zn-Mg alloys have the greatest potential for age hardening, allowing a wide variety of thermo-mechanical processing routines to optimise the properties. Alloys based on this system can have a UTS as high as 600MPa (7075-T6, 7178-T6). They have the highest strength to weight ratio of all Al alloys. While the alloys are very strong, they tend to be readily susceptible to stress corrosion cracking (SCC). Some aerospace manufacturers reverted to heavier Al-Cu-Mg system. Copper is added to reduce the propensity for SCC. However, alloys without Cu, have a much better weldability. SCC resistance is improved by careful processing and microalloying. Major uses: Transportation industry Superplastic alloys Processing route to obtain very fine grained 7475 Superplastically formed car tailgate panel Hot superplastic forming in action Superplasticity is the ability of a material to accommodate abnormally large extensions (as great as 1000%) without necking or fracturing. An alloy has to satisfy two requirements: (1) consist of two stable phases in roughly equal proportions (e.g. eutectoid), (2) have a very fine grain size (1-2m). Some aluminium systems exhibit superplasticity: 5085 SPF (Al-4.5Mg-0.7Mn-0.15Cr), Al6Cu-0.5Zr, but have limited applications. Current emphasis is on developing superplastic behaviour in high-strength 7xxx series alloys via thermo-mechanical processing (e.g. 7475). The alloy can accommodate elongations in excess of 500%, but forming strain rates are very low, the forming temperature must be suitably high, and the alloy is expensive as a consequence. Cast Al alloys Anodising Engine Block Transmission Casing Cookware The ratio of cast aluminium to wrought is constantly increasing This is mainly due to more castings used in automobile industry 70% of all Al castings in Western Europe are for automotive In 2004 the Cast:Wrought ratio in USA automotive use was 1:2 Castings divided into “primary” prepared from new metals and “secondary” which use recycled stock and have more impurities Most common systems are: Al-Si, Al-Si-Mg and Al-Si-Cu When to use Al castings? Advantages Low density Low melting point Low gas solubility (except H) Better fluidity than wrought compositions Good surface finish Allow control of grain size Allow artificial ageing Complex product shapes (in fewer steps) Superior creep properties to wrought alloys Disadvantages Inferior mechanical properties to wrought alloys Low ductility Susceptible to casting defects High hydrogen solubility High shrinkage (difficult to control geometry) Importance of Al stock Aluminium is supplied to foundries in the following stock forms: en.wikipedia.org Dross from Al smelting/casting. www.atherm.com 1. Primary metal ingots from a smelter supplied as 99.50-99.85% purity Al or pre-alloyed. 2. Secondary metal ingots from recycled aluminium alloy products with composition adjusted according to needs 3. Molten metal delivered directly to foundry from an external smelter facility. 4. In-house scrap returns. Increasing the use of secondary Al increases the loss of metal to dross – a mass of solid impurities/metal oxides floating on or dispersed in the melt. Transportation of molten aluminium by road. However, due to low cost of secondary aluminium, foundries may implement de-gassing and filtering of the melts prior to casting. What defines castability? The castability of an alloy depends on a combination of factors: Fluidity Mould filling ability Volume shrinkage Succeptibility to hot tearing Porosity forming characteristics Surface quality Dross forming characteristics The melt temperature will affect some of these factors and is therefore an important variable to consider. In addition to alloy castability, it is also important to consider the castability of a component’s geometric design. E.g. Vc/Vb where Vc and Vb are volume of component and volume of smallest box it fits in. (Smaller ratio is easier to cast.) Fluidity Fluidity is usually measured using a spiral mould and measuring the length run out by the molten metal. Fluidity depends strongly on the composition since it affects: viscosity, surface tension, freezing range and solidification mode. Impurities in Al reduce fluidity, by widening the freezing range, forming intermetallics, and changing planar front solidification to mushy modes. Fluidity is greatest at eutectic compositions. Left: Fluidity of a metal matrix composites based on alloy LM6 containing different fractions of silicon carbide particles. The carbide particulates act to decrease the fluidity. Precipitation of intermetallic inclusions during solidification has similar effects. American Journal of Materials Science 2012, 2(3): 53-61 DOI: 10.5923/j.materials.20120203.04 Volumetric shrinkage Total volumetric shrinkage is strongly dependent on composition. Each phase present has its own density characteristics. Pure aluminium exhibits 6% shrinkage whereas hypereutectic Al-Si exhibits 1-2%. Shrinkage may only be manifested as an evident contraction in the solid. It can also result in a number of defects: surface defects such as pipes (a), large isolated voids a.k.a. gross porosity (b), interconnected porosity, such as centerline (c) and interdendritic microporosity (d). Porosity Shrinkage micropore in alloy 319 https://www.nde-ed.org Radiogram showing entrapped gas porosity. Most casting processes have some associated porosity. Turbulent flow can result in entrapment of gases, forming bubbles that freeze as pores. Entrapped gases can also be a result of moisture, dissolved hydrogen or combustible lubricants. Evacuating the mould can overcome entrapped gas porosity problems. Porosity also arises from volume shrinkage. Moulds are designed to concentrate porosity in risers or to redistribute it uniformly as less harmful micropores. Hot tearing Hot tearing occurs when the stresses generated within a casting as it solidifies are too great. It is evident as tearing or cracking in the casting. The phenomenon occurs at locations where the shrinking casting is physically restrained. Alloys with wide freezing ranges are more susceptible. Alloys with low volume fraction of eutectics are also more susceptible since the eutectic composition liquid can fill and repair hot-tears as they form. Al-Si based alloys have the greatest resistance. Al-Cu and Al-Mg systems are most prone. Die soldering Die soldering is a particular problem in die casting of Al alloys. The molten Al alloys can react with steel dies causing the casting to solder to the die surface. Iron and molten aluminum have a high affinity for one another. Rapid interatomic diffusion can result in formation of aluminide intermetallics at the die surface, which facilitate bonding. This makes automatic ejection from the die impossible and castings must be removed manually, causing delays. To minimise likelihood of soldering: (1) add iron to the alloy (2) use lowest possible melt injection temperature (3) use die surface coatings (e.g. boron nitride) Relative castability Below are the relative castabilities of Al alloys ranked according to their main alloying additions: Best Worst 3xx > 4xx > 5xx > 2xx > 7xx Si+Cu/Mg Si Mg Cu Zn Nomenclature (USA) >99.00% Al 1xx.x Cu 2xx.x Si with added Cu or Mg 3xx.x Si 4xx.x Mg 5xx.x Zn 7xx.x Sn 8xx.x Other 9xx.x Unused series 6xx.x The first digit identifies major alloying element or alloy group. In 1xx.x alloys the next two digits indicate minimum Al percentage. E.g. 150.x is 99.5% Al. In 2xx.x to 9xx.x the 2nd two digits only serve to identify individual alloys in the group The last digit to the right of the decimal point indicates the product form: 0 = casting and 1 = ingot. The system used to denote temper is the same as that for wrought alloys. Al-Si alloys Alloys with Si as the major addition are the most important casting alloys. Si addition substantially increases the “fluid life” of the melt. This is because Si has a much higher heat of fusion than Al, (1810 vs. 395kJ/kg). Thus, the significant latent heat keeps the metal molten for longer. Other advantages: Corrosion resistance Good weldability Reduced CTE Reduced shrinkage Disadvantages: Difficult to machine due to hard Si particles Uses of binary alloys: castings where strength is not a prime consideration: cookware, pump casings, manifolds. Al-Si alloys microstructure The eutectic composition is 12.6 wt.% Si. Hypoeutectic alloys are more commonly used. “Fluid life” is better in hypereutectic alloys. Al-9.8wt.%Si Al-19wt.%Si Slow solidification (e.g. sand casting) produces a very coarse microstructure comprising large silicon plates in an Al solid solution matrix. Rapid cooling (e.g. permanent mould casting) produces a more refined microstructure. The silicon adopts a fibrous morphology, improving ductility and strength. Alloy modification can also be used to refine the eutectic. Modification of Al-Si Light Alloys – I. Polmear A357 (a) Unmodified, (b) 300 ppm Sr, (c) 2400 ppm Sr. Light Alloys – I. Polmear Condition UTS (MPa) Elongation (%) Hardness (Rockwell B) Sand cast 125 2 50 Modified sand cast 195 13 58 Chill cast 195 3.5 63 Modified chill cast 220 8 Mechanical properties of Al-13%Si alloys. 72 Addition of very small amounts of alkali metals, such as Na or Sr, is used to refine the eutectic microstructure. Mechanical properties are thus improved. It is likely that modification alters the nucleation and growth of silicon during solidification. Excessive modification does revert the microstructure to a coarser morphology. Intricate castings possible with Al-Si The high fluidity of Al-Si alloys allows very intricate thin-walled castings to be produced. Al-Mg-Si alloys Microstructure of Al-12.7Si-0.7Mg Arrows show phase in alloy 357 together with smaller eutectic Si particles. Some particles have cracked. Small additions of Mg induce a significant age hardening effect. This is due to precipitation of disperse intermetallic phases (e.g. Mg2Si). 356 (Al-7Si-0.3Mg) and 357 (Al-7Si-0.5Mg) are examples of alloys widely used for sand and permanent mould castings. The T6 temper of these alloys have yield strengths that are double those than the equivalent alloys without magnesium. Higher Mg contents tend to lead to formation of undesirable phases such as (Al9FeMg3Si5) which are brittle and crack preferentially. Uses of Al-Mg-Si Light Alloys – I. Polmear Precision cast fighter aircraft pylon. Due to their mechanical strength alloys such as 356 and 357 are precision cast to produce premium castings for the aerospace industry. The Al-Si-Mg alloys also have excellent corrosion resistance, due to a low galvanic potential between the elements. Fatigue performance, however, is insensitive to ageing treatment since crack nucleation preferentially occurs at casting defects. Al-Si-Cu alloys More complex compositions have been developed for specific applications. A332 (Al-12Si-1Cu-1Mg-2Ni) – has improved high-temperature strength arising from Ni-containing intermetallic precipitates. A390 (Al-17Si-4Cu-0.55Mg) is a wear-resistant alloy used to produce allaluminium automotive cylinder blocks. This has allowed to move away from cast iron cylinder blocks. A lightweight engine has been designed by BMW using an A390 cylinder block core in a magnesium alloy jacket that is sand cast around it. BMW N52 engine block. Other castable alloys Al-Cu Al-Mg Al-Zn Age hardenable Corrosion resistant Age hardenable Prone to hot tearing Machinable Natural ageing Not age hardenable Largely obsolete Troublesome casting Silver additions have allowed production the strongest possible castings using premium techniques UTS of 550MPa and elongations of 10%. Expensive Ag used only in military A20X aerospace casting E.g. hydrogen evolution during sand casting Prone to SCC Used in sacrificial anodes Poor casting characteristics High eutectic melting point allows brazing of castings. Sand casting Sand casting is a low-cost method for producing aluminium castings. A solid pattern is encased in sand mixed with a special binder. Removing the pattern creates the cavity into which molten metal is cast. When the molten metal has solidified, the compacted sand is removed to release the finished casting. The method does not allow for complex casting geometry. The final surface finish of the castings is also comparatively rough and depends on the coarseness of the sand. Permanent mould casting Also known as gravity die casting, this method employs a re-usable mould for higher-volume production of castings. Metal fills the mould under the force of gravity, which limits geometric complexity of castings. A finer surface finish is possible with this method. Pressure die casting To improve the molten metal’s die-filling ability, it can be fed into the die under pressure. This is usually achieved using a piston or a screw to feed the molten metal into the die cavity. Using cooled metal dies and mechanical ejectors allows for high-volume production output. The pressure exerted on the alloy allows more complex moulds to be filled. The metal die allows for a smooth finish in the castings. Squeeze casting Improvements in mechanical performance of 7010 subjected to squeeze casting. Squeeze casting allows us to overcome problems associated with shrinkage during solidification. Pressures around 200MPa are applied to the melt. Flow of melt into incipient shrinkage pores is facilitated. Entrapped gases are forced to remain in solution. Direct squeeze casting = a moving die is used to exert pressure. Indirect squeeze casting = a piston is used to exert pressure. Allows casting of more complex alloys traditionally limited to wrought processing. Can be used for MMC manufacture by adding fibres or particulates. Semi-solid processing Cast dendritic structure. The casting is usually a near-finished product and cannot be mechanically worked. Grain refiners are an option but can lower the mechanical properties. One option is to break up the dendrites during solidification creating more grain nuclei in the melt. This is semi-solid processing. Fluidity persists with up to 60% solid. Cast globular structure. How does one refine the grain size of an aluminum casting? Semi-solid slurries are thixotropic – i.e. their viscosity decreases with stirring. Commercialisation is still at an early stage. Semi-solid processing Two established methods exist: rheocasting and thixoforming. Thixoforming requires specialised billets to be produced which is expensive. Rheocasting alows the use of existing alloys. They slugs are heated electromagnetically to a mushy Liquid+Solid state before high-pressure die casting. Summary Aluminium alloys are the second most used alloy system after steels. Aluminium is the most abundant metal in the earth’s crust, but requires considerable energy to extract from the ore. Recycling is thus very important. The alloys have a comparatively low melting point and are thus relatively easy to process which, contributes to their widespread use. They are quite a representative metallic system and provide a good introduction to the processing of engineering alloys. Aluminium alloys show a good combination of physical properties. Particularly, they have a comparatively high strength-to-weight ratio. The mechanical properties are either controlled by deformation (work-hardening and grain refinement) or by ageing (precipitation of strengthening phases). Age-hardenable aluminium alloys are typically stronger than strain-hardenable aluminium alloys but are less weldable. There are two families of aluminium alloys: wrought and cast. Wrought alloys start as castings, but are made into their final products using plastic deformation. Cast aluminium alloys are cast into the final product geometry. The castability of an alloy refelcts its suitability for cast applications and is a combination of a number of factors: viscosity, fluidity, shrinkage...