Dentine Basic Structure and Composition - PDF
Document Details
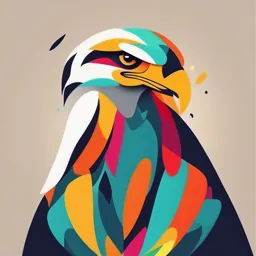
Uploaded by HandierMemphis
London South Bank University
Leo Tjaderhane et al
Tags
Summary
This article reviews the basic structure and composition of dentin, including its collagenous components, minerals, and specific features, like the dentin-enamel junction (DEJ), mantle dentin, inter- and peritubular dentin, and pulp stones. It discusses the role of odontoblasts in dentin formation and function, and explores variations in dentin composition across different parts of the tooth.
Full Transcript
bs_bs_banner Endodontic Topics 2012, 20, 3–29 2012 © John Wiley & Sons A/S All rights reserved ENDODONTIC TOPICS 2012...
bs_bs_banner Endodontic Topics 2012, 20, 3–29 2012 © John Wiley & Sons A/S All rights reserved ENDODONTIC TOPICS 2012 1601-1538 Dentin basic structure and composition—an overview LEO TJÄDERHANE, MARCELA R. CARRILHO, LORENZO BRESCHI, FRANKLIN R. TAY & DAVID H. PASHLEY Dentin is the most voluminous structural component of human tooth. Dentin protects pulp tissue from microbial and other noxious stimuli. It also provides essential support to enamel and enables highly mineralized and thus fragile enamel to withstand occlusal and masticatory forces without fracturing. Furthermore, it is the first vital tissue to meet external irritation, and instead of being merely a passive mechanical barrier, dentin may in many ways participate in dentin–pulp complex defensive reactions. Even though dentin is usually considered as an entity, different parts of dentin may have special qualitative properties that help dentin to meet all of its required demands. The aim of this review is to provide an overview of the basic structure and composition of dentin, including the collagenous components of the dentin organic matrix and minerals. We also describe the specific structural and functional features of the dentin–enamel junction (DEJ), mantle dentin, inter- and peritubular dentin, and pulp stones. Received 21 August 2010; accepted 25 January 2011. Dentin, which comprises the bulk of teeth, is mineral- Dentin is formed by highly specialized, terminally ized connective tissue that bears a strong resemblance differentiated cells—odontoblasts—that are believed to bone. Dentin has phylogenetically been thought to to be almost exclusively responsible for the constitu- be derived from bone (1). In this view, the most primi- tion of dentin. For decades, the sole function of the tive hard tissue was mesodentin, a cellular tissue with odontoblasts was believed to be the formation and unpolarized cell processes reminiscent of cellular bone. maturation of dentin, but recent years have revealed That was followed by semidentin in which odontoblasts that odontoblasts may have much more diverse remained entrapped within the mineralized matrix, but functions. Odontoblasts may, for example, participate the cell processes were strongly polarized in a single di- in the dentin–pulp complex innate immune defense rection; and finally by orthodentin, the most advanced and transmittance, and in the regulation of pulpal structure. It has also been suggested that the evolution pain. For the sake of clarity, the dentin–pulp inter- of bone may stem from dentin-like tissue (2–4). Both of face, including the various possible roles suggested these theories have recently been questioned. There is for the odontoblasts, are discussed in detail in no phylogenetic evidence to support Ørvig’s model of another article in this issue. Also the numerous and dentin evolution since all grades of dentin are manifest diverse dentin non-collagenous proteins, partici- among the earliest skeletonizing vertebrates (5). Hard pating in various tasks from the regulation of den- tissues (bone, dentin, enamel/enameloid, and carti- tin mineralization to non-specific defenses against lage) in primitive vertebrate skeletons are fundamen- microbes, deserve reviews of their own which are tally distinct from their first inceptions (5,6), although found in the next issue. The aim of this review is the reason for the high diversity early in vertebrate mainly to present the constitution and structural phylogeny remains to be answered (5). components of dentin. 3 Tjäderhane et al. a b Fig. 1. (a) Scanning electron microscope (SEM) image of the pulp chamber dentinal wall of mouse molar. Pulp tissue, odontoblasts, and predentin have been mechanically removed, exposing the tubule openings. Magnification = 1,000 ¥; bar = 10 mm. (b) Number and radius of tubules with respect to the coronal dentin depth in human teeth. Data adapted from Pashley, 1996 (8). Constitution of dentin Structurally, dentin can be described as a nanocrys- talline reinforced composite, whereas enamel would be The composition of dentin may be described in two a dense ceramic with impurities, even though recent ways: extracellular dentin consists of a mineralized research indicates that enamel behaves more in a organic extracellular matrix (mechanical approach), metal-like manner in terms of elastic and plastic prop- while functional dentin includes predentin and dentin- erties (9–11). The composition and structure of forming cells (odontoblasts) with their cytoplasmic dentin varies between the different parts of the tooth processes penetrating mineralized dentin, and dentinal (12,13). Because of the tubular structure, with more fluid (the biological entity). The mineral phase com- or less patent tubules surrounded by intertubular prises approximately 70% of the weight percentage and dentin (Fig. 1a), dentin is a highly permeable structure 45% of volume, and the organic matrix about 20% and in which not only outward flow of dentinal fluid but 33%, respectively, the remaining fraction being water also inward movement of, for example, microbial (7). However, since water is located primarily in den- components may occur. Dentinal tubules radiate from tinal tubules, and the tubule diameter increases signifi- the dentin–pulp border through the entire dentin, cantly from the dentin–enamel junction toward the with the exception of the outermost layers in mantle pulp (for details, see below), these percentages are dentin and in the dentin–enamel junction (DEJ) and only average values. The water content—or wetness— adjacent to the cementum. The tubular width is largest of dentin is not uniform, but varies approximately close to the pulp, and decreases toward the enamel 20-fold from superficial to deep dentin (8). (Fig. 1b). Consequently, the volume of the dentin 4 Overview of dentin structure occupied with open dentinal tubules decreases toward dentin. The presence of enzymes (16,17) and growth the DEJ. factors such as fibroblast growth factor-2 (FGF-2) Dentin can be divided into several types according to (16) suggests that the DEJ region represents an area of the site, function, and origin of the dentin. Broad biological activity. It may liberate and activate the discrepancy in terminology exists; Cox et al. (14) stored growth factors and other potentially bioactive reported 20 different terms of dentin used in 154 components that may exert their effects at a location articles or book chapters. Most commonly, dentin is distant from the DEJ (16). Based on phylogenetic, divided into five different types according to the for- developmental, structural, and biological characteris- mation phases: dentin–enamel junction, mantle tics, it has been suggested that instead of the dentin– dentin, primary dentin, secondary dentin, and tertiary enamel junction, this structure should be termed the dentin. Tertiary dentin, representing defensive dentin dentin–enamel junctional complex (16). formation to protect the pulp tissue, can be further The DEJ in human teeth is not smooth, but wavy or divided into reactionary and reparative dentin depend- scalloped (18–22) (Fig. 2). This kind of an interface is ing on the cells forming the dentin (primary or believed to improve the mechanical interlocking replacement odontoblasts, respectively). between dentin and enamel. The size of the scallops Mineralized extracellular dentin is further divided ranges between 25 and 50 mm, and they are deeper into intertubular and peritubular dentin. Intertubular and larger at the dentin cusps and incisal edges, lev- dentin is formed by odontoblast during dentinogen- eling down toward the cervical region (18,21,23). esis, and it forms through predentin mineralization. This is in accordance with finite-element studies dem- Intertubular dentin comprises most of the dentinal onstrating that the mechanical interlocking between volume. Peritubular dentin is formed by mineraliza- enamel and dentin is weaker in the cervical region tion inside the walls of dentin tubules within mineral- (24). In addition, smaller (0.25 to 2 mm) “secondary ized dentin, and may be totally absent near the pulp in scallops” within the “primary” scallops have been human teeth. demonstrated (21,23), and upon close inspection the intermingling ridges of dentin and enamel, less than 1 mm wide, are clearly visible. It is generally thought Dentin–enamel junction that the scalloping structure of the DEJ can be The dentin–enamel junction (DEJ) has traditionally explained as required for the tooth to withstand func- been thought to be merely a simple anatomical inter- tional stress (7). This assumption has been questioned, face between enamel and dentin, seen on sections as a though, as humans are among very few species in scalloped line between two mineralized structures. which the scalloped form of the DEJ has been dem- However, recent studies have demonstrated that the onstrated (23,25). DEJ may be much more than an inactive border In addition to the scalloped morphology of the DEJ, between two different hard tissues. With laser-induced there are basically two possibilities to increase the autofluorescence and emission spectroscopy, the DEJ mechanical interlocking between enamel and dentin: appears as a 7 to 15 mm-wide structure distinct from the continuity of mineral crystals from dentin to both enamel and dentin, and composed of large enamel, and organic interlocking material (25). The amounts of organic and mineral matter (15). It has continuity of mineralization crystals between develop- also been suggested that the DEJ forms a complex of ing dentin and enamel at the DEJ has been a matter of two unique, thin adjacent layers: the inner aprismatic debate for a long time. It has been suggested that enamel, which differs to some extent from the pris- enamel crystals grow epitaxially on the pre-existing matic enamel; and the mantle dentin, which also is dentin crystals because of an apparent high continuity similarly related but still distinct structurally compared between enamel and dentin crystals (26,27). Others to circumpulpal dentin (16). have claimed that enamel crystals are formed at a given Even after dentin and enamel formation and miner- distance from the dentin surface (28) and could either alization are well underway, specific biological events grow into contact with dentin crystals (29) or remain may still occur at the DEJ, suggesting that the cross- distant (30,31). However, enamel and dentin have talk between enamel and dentin continues throughout been demonstrated to be linked by 80–120 nm diam- the formation of prismatic enamel and circumpulpal eter collagen fibrils inserted directly into the enamel 5 Tjäderhane et al. a b c d Fig. 2. Dentin–enamel junction (DEJ) in human primary molar. (a) Enamel cap has been removed, revealing the scalloped structure of the DEJ. The size of individual scallops is approximately 25 to 50 mm. (b–c) Lower primary canine of a human fetus: the box and arrow indicate the area examined at higher magnification in (c), where the ridge-like interface (dotted line) between enamel and dentin is clearly visible in the cross-section of the DEJ. (d) In “primary” scallops, smaller “secondary” scallops (0.25 to 2 mm) are located, further increasing the irregularity of the DEJ. Reproduced with permission from Radlanski et al., 2007 (23). and merging with the interwoven fibrillar network of cal requirements of the junction. The collagen the dentin matrix (32) (Fig. 3). Immunogold labeling network could provide efficient stress transfer from indicated that the fibrils contain type I collagen, but it enamel to dentin and resistance to the tensile and shear is not impossible that these coarse fibers would actually forces developed during masticatory function (Fig. 3). be so-called von Korff fibers, consisting of type III collagen and fibronectin (7), and frequently reported Mantle dentin to occur during the initial phases of dentinogenesis. In any case, these findings indicate that the DEJ connec- The mantle dentin is a layer of 5 to 30 mm in thickness tion may be textural and structural, rather than bio- in humans (12) and differs from the rest of the dentin chemical, reinforced with fibrils traveling from dentin in that its organic matrix is more irregular. The von to enamel across the width of the DEJ. The authors Korff fibers have been frequently reported in mantle suggest that the DEJ could be regarded as a fibril- dentin (25). These fibers consist of coarse, bundled reinforced bond mineralized to a moderate degree collagen fibrils of type III, with a minor portion of (32), which is believable due to the high biomechani- type I (33), and run with their long axis parallel to that 6 Overview of dentin structure a b e c d Fig. 3. Dentin–enamel junction (DEJ). (a) TEM image of rat incisor DEJ, where the inner aprismatic enamel (IAE) and the mantle dentin (MD) meet. Magnification = 21,600 ¥. From Goldberg et al., 2002 (16), reproduced with permission. (b) Field emission-SEM (FE-SEM) image of human DEJ, following surface decalcification with EDTA. The image illustrates the scalloping outline of DEJ (asterisk). E, enamel; D, dentin. Original magnification = 1,000 ¥; bar = 10 mm. (c) Detailed image of DEJ, demonstrating the penetration of fibrillar structures (arrowheads) into the enamel (E). Collagen fibrils of the DEJ area exposed after partial decalcification with EDTA. Original magnifica- tion = 10,000 ¥; bar = 1 mm. (d) The collagen fibrils have a cross-banding appearance and are 80–120 nm in diameter (small arrows). These coarse fibrils merge with finer fibrils from the dentin matrix (arrowhead) and split before entering into the enamel (large arrows). The fibrils were identified as type I collagen with immunogold labeling (not shown). E, enamel. Original magnification = 50,000 ¥; bar = 200 nm. Figures b to d are from Lin et al., 1993 (32), reproduced with permission. (e) The proposed collagen structure from dentin through the DEJ into mineralized enamel. Modified from Lin et al., 1993 (32). of the odontoblast processes (7). Mantle dentin is also The totally different mode of formation of mantle different in biochemical composition (12): for dentin compared to the rest of dentin is clearly seen in example, it seems to be absent of phosphoproteins patients with hypophosphatemic vitamin D-resistant (34,35). The mineral content of mantle dentin has rickets, in which dentin is mainly globular, but mantle long been thought to be lower than that of circumpul- dentin is not affected (16,40–43). Mantle dentin also pal dentin, but it has also been suggested that the differs from circumpulpal dentin as it does not contain differences in the degree of mineralization are not dentinal tubules, only sometimes thin tubular limited to mantle dentin but may be more gradual branches (44). However, the atubular structure of (36,37) (Fig. 4a). This seems to be contradicted by mantle dentin does not result in a lack of permeability studies which demonstrate that the content of mineral (45–47). The multiple branching of the odontoblast elements does not vary markedly between mantle and processes indicates that the mantle dentin matrix is circumpulpal dentin (38). This apparent discrepancy heterogeneously secreted by differentiating or newly may be explained by the differences in dentin consti- differentiated odontoblasts, which may initially lack tution. The volume percentage of peritubular dentin odontoblast processes that create patent tubules. It increases dramatically from the DEJ toward the pulp, must be noted, however, that the fate of the proteins while that of intertubular dentin decreases (8) of the degraded basement membrane between differ- (Fig. 4b). As peritubular dentin is much harder than entiating ameloblasts and odontoblasts is not known, intertubular dentin, and dentinal hardness is inversely and these degradation products may well be, at least related to tubule density (39), this change may con- partially, integrated into mantle dentin ground sub- tribute to the overall hardness differences seen in stance prior to mineralization. Also mineralized globu- dentin. lar structures, about 2 mm in diameter, can be seen 7 Tjäderhane et al. a b Fig. 4. The gradual increase in mineralization, indicating the existence of a “resilience zone” beneath mantle dentin. (a) Back-scattered electron (BSE) image of human premolar slice. BSE image demonstrates the degree of minerali- zation in a manner similar to radiography: the higher the mineralization, the whiter the image. Enamel (top and right of the image) is all white; 150 to 200 mm of dentin under the DEJ appears darker, indicating a lower level of mineralization, with gradual increase in the mineralization level toward the pulp. Reproduced with permission from Wang et al., 1998 (36). (b) The relative proportion of intertubular dentin (ITD), peritubular dentin (PTD), and dentinal tubules filled with fluid in human teeth with respect to dentin depth. *: Peritubular dentin is not present at the immediate pulpal surface, but begins close to it. Data are from Pashley, 1996 (8) (see also Fig. 8). embedded in a network of interglobular dentin in primary dentinogenesis, dentin formation continues as crown mantle dentin (12). secondary dentin, which is formed at a much slower It has been proposed that mantle dentin is largely rate. To date, the absolute point of change in odon- responsible for the elastic properties of teeth, allowing toblast activity reflecting the change from primary to relatively high occlusal loads without fracture of secondary dentinogenesis is ill-defined and constitutes enamel or dentin. However, recent findings indicate a terminological controversy. It has been postulated that this “resilience zone” is not clear-cut. Instead, it that primary dentinogenesis ends when the crown is has been suggested to also include varying depths of complete. This assumption is supported by the finding sub-DEJ circumpulpal dentin (36,37,48,49). In this that cell organelles undergo atrophy at that point in rat zone, both changes in collagen fibril direction in inter- molars (50). Primary dentinogenesis has also been tubular dentin (49) and a gradual increase in miner- timed to end when teeth becomes functional (12) and alization from the DEJ toward the pulp (36,37) have when root formation is complete (7,51). The time been detected, which may contribute to the mechani- span is large; for example, in human first upper molars cal properties in this area. The mechanical properties it is longer than 6 years if counted from the comple- of dentin are discussed with detail elsewhere in this tion of the crown at the age of 2.6–2.7 years to issue. the closure of the root apex at the age of 9.2–10.1 years (52). The concept of a distinct change from primary to Circumpulpal dentin secondary dentinogenesis could also be challenged. Johannessen (53) calculated the dentin formation rate Primary and secondary dentin in molars of young albino rats and noticed that the The main portion of dentin is called primary dentin, increment of dentin in the mid-occlusal surface of the and it is formed rapidly during tooth formation. There lower molars during weeks 0–3, 3–6, and 6–9 was are several differences in primary and mantle dentin 10.2, 7.3, and 5.1 mm, respectively. Thus, dentin for- formation: the organic matrix is completely formed by mation seems to slow down gradually, even though all odontoblasts and the collagen matrix is more compact. of the molars reach occlusion at 3–4 weeks of life. Primary dentin forms the bulk of the tooth and gives Also, the dentin formation rate in young rat molars it the size and form determined genetically. After (3–7 weeks) is about 10 times faster than that in older 8 Overview of dentin structure (15–30 weeks) rats (54,55). Overall, these studies Type III collagen, widely seen in soft connective indicate that the dentin formation rate decreas- tissues, is not normally seen in intertubular dentin es gradually, at least in rat molars. The reduction matrix. In developing human teeth, Lukinmaa et al. in dentinogenesis activity is also accompanied by a (67) demonstrated the expression of type III pro-alpha marked change in the odontoblast gene expression collagen mRNA, and type III collagen immunoreac- profile (56). tivity was observed in early predentin and again in Secondary dentin differs only slightly from primary predentin toward the completion of dentinogenesis, dentin: the curvature of dentinal tubules may be when mRNA was no longer detected via in situ slightly different and the tubular structure may be less methods. However, mature human odontoblasts regular. The deposition of dentin may also be uneven, express type III collagen mRNA detected with PCR, as in human teeth the greatest dentin deposition is and produce the protein in tissue culture (68). Several frequently seen in the floor and roof of the pulp studies (69–71) have found that type III collagen chamber, especially in molar teeth (7). localizes in dentinal tubules. Since it has frequently been related to von Korff fibers (70,72), it is possible that the staining seen in deeper areas of dentin (69,71) Composition of dentin ECM may represent intact or fragmented remnants of these The organic extracellular matrix (ECM) of dentin is fibers. similar to soft tissue ECM and especially to bone With other collagens, the data are much more incon- ECM, but in some respects it is unique. The high level sistent. A small amount of type V collagen is synthe- of collagen cross-linking in mineralized tissue (57,58) sized by odontoblasts (63); it has been shown in rat and virtual absence of type III collagen are the main and hamster predentin and dentin in developing teeth differences with soft tissue collagens. Generalized con- (73), and is weakly stained in human predentin (74), clusions of the significance and function of the differ- but it is absent in mineralized dentin (69,74). Similarly ent ECM components in dentin are caused by the conflicting results have been presented for type VI findings that demonstrate differences in dentin ECM collagen. Becker and co-workers (69) reported a type between species and even between the different parts VI collagen staining distribution essentially similar to of the tooth. that of type III collagen, with relatively strong inten- About 90% of the dentinal organic matrix is colla- sity in predentin and occasional fibrous staining in gen. The major component of dentin collagen is type dentinal tubules. However, Lukinmaa et al. (74) could I (59,60), of which the majority is a heteropolymer not detect type VI collagen in dentin. Type IV colla- with two a1(I) chains and one a2(I) chain (60). gen has not been seen in normal dentin (71). These Odontoblasts also synthesize and secrete a type I col- conflicting findings may reflect differences, for lagen homopolymer consisting of three a1(I) chains example, between species or in section pre-treatment, and commonly called type I trimer (60–64). The antibodies, or detection methods. experiments with rat incisor odontoblast organ culture Becker et al. (69) suggested that the role for colla- (61) and analysis of rat incisor predentin (63) indicate gens other than type I collagen in mineralized tissues that approximately 25 to 30% of the synthesized type may be related to ECM (bone and dentin) remodeling I collagen would be the type I trimer. However, in before hard tissue calcification. This is in accordance bovine extracts containing odontoblasts and preden- with the expression of various ECM protein-degrading tin, only about 3% of the type I collagen was type I enzymes, matrix metalloproteinases (MMPs) (75), and trimer (62). The presence of type I trimer in mineral- cathepsins (76) (for details, see the article on dentin ized dentin has only been shown in lathyritic rat inci- non-collagenous proteins in the next issue). The role sors in which the collagen cross-linking was inhibited of enzymatic regulation of collagenous and non- by dietary lathyrogen (b-aminopropionitrile) (65,66), collagenous components in the control of dentin and it is not known if type I trimer would be present in ECM maturation prior to mineralization has also been normal dentin (60,65,66). Butler (60) concludes that suggested by Butler et al. (60,77). Type III collagen is type I trimer is important for dentin formation but also present in dentinogenesis imperfecta (78) and in that it may be involved in the maturation of predentin reparative dentin under carious lesions (79,80), which and degraded prior to cross-linking. may, at least partially, be related to the disturbances of 9 Tjäderhane et al. a b Fig. 5. (a) Intensive branching of dentinal tubules close to DEJ (arrows). (b) Intensive branching of dentinal tubules in the middle part of dentin. The tubules are visualized with Alizarin red. Bars = 20 mm. Reproduced with permission from Kagayama et al., 1999 (82). the enzymatic or other reactions controlling the 90 degrees. Slightly further (0.5 mm) into dentin, no matrix maturation rather than the expression of type more tilting or curling occurred, presumably because III collagen. of odontoblast crowding (81). In addition, a differ- About 10% of the dentin organic matrix consists of ence in tubule orientation relative to the DEJ was proteoglycans and other non-collagenous proteins, observed between upper and lower teeth (81). While and less than 2% is lipids. Non-collagenous proteins these findings require confirmation, it can be specu- are also produced by odontoblasts; they are distributed lated that the difference in tubule orientation between between the collagen fibrils and accumulate along the upper and lower teeth can affect the response to teeth dentinal tubule walls, and are supposed to serve loading, which might, for example, cause differences in important functions in the mineralization process of the deformation of the crown under mastication (81). dentin (7). The presence and potential roles of non- The number of dentinal tubules in different locations collagenous proteins in dentin and dentinogenesis are in relation to the DEJ or cementum does not vary discussed in detail in other articles in this double issue. except under the cuspal area, where the number of dentinal tubules close to the DEJ is significantly higher (44). This may relate to the regulation of the pulp– Dentinal tubules dentin defensive systems against wear (for details, Tubularity is a central characteristic of dentin, affect- please see the “Tertiary dentin” section below). ing, for example, its mechanical properties, its ability In addition to the main tubule, dentinal tubules have to withstand occlusal forces, and its behavior in den- numerous branches and ramifications (Fig. 5). The tin bonding. The understanding of dentin three- number of branches is higher in areas where the dimensional structure may have a significant impact on density of the main tubules is low (44,82), forming an optimal cavity design and restorative procedures. abundant anastomosing system of canaliculi very much The common belief that dentinal tubules extend at like osteocytes in bone (83) (Fig. 6). Mjör & Nordahl right angles from the DEJ and run a fairly direct—or (44) identified three types of tubular branches: major, slightly S-shaped—course through the dentin was fine, and microbranches. Major branches (0.5 to recently questioned in a study utilizing 3D phase- 1.0 mm diameter) are abundant peripherally while fine contrast microtomography (81). In dentin immedi- branches (300 to 700 nm diameter) are abundant in ately beneath enamel (within 0.3 mm), a wide range of areas where the density of the tubules is relatively low. tubule angular tilts (up to 75% of the tubules tilting Microbranches (25 to 200 nm diameter) extend at more than 10 degrees) was seen. Within this area, the right angles from the tubules in all parts of the tubules also seemed to twist or curl, occasionally up to dentin (44). 10 Overview of dentin structure Fig. 6. SEM images of resin-embedded, acid-etched dentin (a) and mandibular bone (b), demonstrating marked similarity between the odontoblast process and osteocyte lacuno-canalicular networks. Reproduced with permission from Lu et al., 2007 (83). An interesting finding of bamboo-like dentinal the degree of communication between the intertubu- tubules with many nodules in the longitudinal sec- lar dentin and odontoblasts via dentinal fluid (84). tions, which appear as circular tubules surrounding the main tubule, was seen with Alizarin red staining (82). Peritubular dentin The circular tubules of the nodules adhere to one side of the dentinal tubules and resemble that of the peri- The commonly used name “peritubular” is actually tubular dentin (Fig. 7). The nodules correspond to misleading, since the prefix “peri” (“around”, “sur- the tubular branching penetrating through thick peri- rounding”, “enclosing”) refers to material formed tubular dentin observed with SEM (44). Peritubular around the tubules. Since peritubular dentin in most dentin, even though highly mineralized, is porous and species (including humans) is deposited on the inner the nodules observed with Alizarin red indicate a sug- surface of the tubular lumen by the odontoblasts only gested function for peritubular dentin in regulating after the formation of intertubular dentin, a more 11 Tjäderhane et al. a c b d Fig. 7. (a) Longitudinal section of dentinal tubules in the middle part of dentin observed with Alizarin red demonstrates numerous circular nodular structures around main tubules. (b) In cross-section the staining is located at the interface between peritubular and intertubular dentin, with occasional branches penetrating into intertubular dentin. The nodules were absent in dentin close to DEJ, at the pulpal part of dentin (c and d), and in teeth extracted from young patients. Bar = 5 mm, applies to all figures. Reproduced with permission from Kagayama et al., 1999 (82). correct phrase would be intratubular dentin (12,13). mately 1 mm (Fig. 8). Concomitant with this thicken- However, “peritubular” is still most commonly used, ing, the tubular density per unit volume increases and therefore will also be used in this review. (8,49). Peritubular dentin is sharply demarcated from inter- The mineral content of human peritubular dentin is tubular dentin. It is considered to be more mineralized approximately 40% higher compared to intertubular and practically free of collagenous matrix, although dentin (7). The differences in mineral structures both symmetry and degree of mineralization may vary between inter- and peritubular dentin indicated in significantly. The deposition of peritubular dentin earlier studies (85,86) have been, at least to some causes a progressive reduction in the tubule lumen extent, questioned by more recent studies indicating (dentin sclerosis). During environmental stimulation little difference in the nature, size, and organization of and irritation, the formation of peritubular dentin may the mineral phase between inter- and peritubular be accelerated (12,13). dentin (84,87–89). Peritubular dentin is spatially more Within mantle dentin, where dentinal tubules termi- homogenous than intertubular dentin, with different nate in small branches, very little peritubular dentin is hardness (39), elastic properties (90), optical aniso- present and the tubules appear as empty channels pen- tropy (91), and fracture properties (92). All of these etrating the intertubular dentin. A thin lining of peri- observations indicate completely different mechanical tubular dentin may already be present at 20 mm from and structural properties of these intimately associated the DEJ (49) (Fig. 8). A gradual thickening of peritu- forms of mineralized dentin structures. bular dentin occurs with increasing distance from the The formation of peritubular dentin begins in den- DEJ until it reaches the normal thickness of approxi- tinal tubules close to (but not at) the mineralization 12 Overview of dentin structure a sent true peritubular dentin, and may represent atypi- cal intratubular calcification, which has been suggested to be a non-vital process (96). Indeed, one of the main differences between inter- and peritubular dentins is that peritubular dentin is essentially collagen-free (13,84,88,97). Overall, peritubular dentin is very low in organic components, which are a mixture of acidic proteins, phospholipids, or possibly proteolipid com- plexes, with small amounts of glycoproteins and pro- teoglycans. Even though the sulfate content of peritubular dentin proteins seems to be low, the pres- ence of chondroitin 4-sulfate (CS-A), chondroitin b 6-sulfate (CS-C), and dermatan sulfate (CS-B) types have been indicated (84). Peritubular dentin is perforated—in addition to tubular branches (44)—by many small pores and fen- estrations (84,94), allowing the passage of tubular fluid and intertubular dentin components across the peritubular dentin (Fig. 9). Based on the analysis of the organic components of peritubular dentin (84), there may be a potential for the calcium-phospholipid- proteolipid components of peritubular dentin to be involved in the signaling and ion transport process- es. Thus, peritubular dentin may also have direct Fig. 8. (a) Thin peritubular lining inside the tubules role(s) in active transport and other regulatory activi- approximately 20 mm from the DEJ in fractured dentin ties between vital intertubular dentin matrix and seen with SEM. (b) Coronal dentin 800 mm below the odontoblasts, participating in retaining the vitality of DEJ. Highly mineralized peritubular dentin (P) is dentin. The active processes in mineralized intertu- clearly distinguishable from intertubular dentin. A crack bular dentin would offer an explanation of some pre- in the right-hand side of the tubule is frequently seen in SEM preparations of tooth crown dentin and most likely viously poorly understood findings regarding the represents an artifact due to sample handling. Modified age-related changes in dentin, e.g. the disappearance with permission from Zaslansky et al., 2006 (49). of matrix metalloproteinase-2 (MMP-2) from intertu- bular dentin with age (76,98) (discussed in more detail in the next issue). This proposal offers a completely front (93), presumably with the accumulation of non- new view not only on the role of peritubular dentin collagenous proteins along the tubule walls (7) (usually thought to act as a passive blockage in den- (Fig. 9). Recent studies with combined SEM and tinal tubules), but also on the vitality of mineralized time-of-flight secondary ion mass spectroscopy (TOF- dentin as a whole. If signaling and active transport SIMS) analyses show that peritubular dentin is a sepa- between intratubular structures and components rate phase from intertubular dentin, forming a distinct (odontoblast processes, tubular fluid) and intertubular annulus within each tubule (84,94), thus contradict- dentin actually occur, the nature and function of ing previous studies which have indicated that crystal- dentin as a tissue should be revisited. lization within the peritubular dentin would be mediated by a collagenous matrix interaction as in Dentin sclerosis intertubular dentin (88,89,95). The contradictory results may, at least partially, be caused by the heter- The main dentin response under carious lesions and ogenous intratubular mineralization occasionally restorations is reactive dentin sclerosis, seen as a trans- shown to contain collagenous and other proteins (13). lucent or transparent zone. The first phase of its for- However, those types of mineralization do not repre- mation in the initial stage of dentinal caries seems to be 13 Tjäderhane et al. a b Fig. 9. (a) Field emission scanning electron microscope (FE-SEM) image of a cross-section of a mouse second lower molar. Pulp tissue with odontoblasts, odontoblast processes, and predentin have been mechanically removed. Dense collagen network covers the tubular walls immediately below the orifice. In most intertubular dentin ridges, the individual collagen fibrils cannot be seen, presumably due to mineralization (arrows). The ridges thus represent the mineralization front. Collagenous mesh is apparent just below the tubule orifice. Slightly deeper, the collagen fibrils are masked with more homogenous structure, representing either non-collagenous proteins or the initial formation of peritubular dentin. Magnification = 10,000 ¥; bar = 1 mm. (b) Higher magnification of the area marked with a square in (a). Relatively sharp borderline between visible fibrils and homogenous surface of non-collagenous proteins or peritubular dentin indicates highly regulated process in this area. The fibril diameter varies between approximately 20 and 100 nm. Globular structures in connection with fibrils and in the homogenous surface—either uniform layer of non-collagenous proteins or the outermost layer of mineralized peritubular dentin—are readily seen (arrowheads). Few pores with approximately 70 nm diameter or less (arrows) are visible, potentially representing the peritubular dentin porosity suggested by Gotliv & Veis (84,94). Magnification = 35,000 ¥; bar = 1 mm. dependent on odontoblastic processes (99). However, (107), while most tubules in the translucent zone may acid etching in vivo and in extracted teeth produces a be totally occluded (108). It has been proposed that transparent layer, suggesting that it is not a vital defen- physiological sclerosis should be regarded as being sive reaction (100). Instead, calcium phosphate dis- different from the tubular sclerosis seen in relation to solved from apatite crystals diffuses more deeply into caries (100,109). Physiological dentin sclerosis may the dentin tubules and precipitates (100–103), at least even reduce the formation of tertiary dentin, possibly at the side of the lesion where the irritation of associ- by reducing the permeability of dentin before the irri- ated odontoblasts is not so intense (100), into what tation occurs, whereas reactive dentin sclerosis does has been termed “caries crystals.” not prevent tertiary dentin formation. Physiological Massler (104) proposed that superficial sclerosis is dentin sclerosis also develops in areas without carious due to the re-precipitation of minerals from carious lesions or irritation, for example on the floor of the lesions and saliva, while deeper sclerosis requires pulp chamber and root canals. In roots, dentin sclero- calcium from the pulp. The degree of sclerosis is sis progresses with age from the apex toward the cer- directly related to the success in arresting the lesion vical portion (Fig. 10) (106,110,111). progression (103), but after the successful arrest of the lesion, mineral uptake from the saliva is very limited Tertiary dentin (105). Reactive dentin sclerosis occurs at all ages but increases both in prevalence and intensity with age, Tertiary dentin forms as a response to external and is reported to be seen more often in males than in irritation—attrition, abrasion, erosion, trauma, caries, females (106). or cavity preparation—in order to increase the thick- Similar to tertiary dentin formation, sclerosis is a ness of the mineralized tissue barrier between the oral non-specific reaction and physiological dentin sclerosis microbes and the pulp tissue. It has also been called especially occurs in older teeth (106–108). It is typi- irritation dentin, irregular dentin, irregular secondary cally observed to decrease in the direction of the pulp dentin, etc. (14). Defensive reactions can be observed 14 Overview of dentin structure very early, with enzymatic changes being seen during less related to the modern diet containing excess the earliest stage of enamel caries both in the dentin– refined sugars, which favors caries formation. More pulp border (104,112–115) and in dentin itself natural, coarse diets are prone to cause abrasion and (104,116–119), but not in pulpal tissues (120,121). attrition, and it is tempting to speculate that tertiary The dentinogenic potential of the dental pulp, including different ex vivo and in vivo experimental models and the chemical and biomolecular agents used in these experiments, has very recently been a extensively reviewed by Tziafas (122). Therefore, ter- tiary dentin is only briefly reviewed here. The aim of tertiary dentin is to protect pulpal tissue by increasing the thickness of dentin between the pulp and external wear or irritation (Fig. 11). Attrition is suggested to be more prone to induce tertiary dentin formation than caries (106). From a physiological point of view, this is acceptable, since caries is more or b Fig. 10. The classical image demonstrating, for the first time, the age-related root dentinal sclerosis advancing from the apical part toward the crown. Longitudinal central 0.5 mm section of an adult tooth photographed by transmitted light shows the transition between trans- parent apical root dentin (dark) and opaque dentin (white). Reproduced with permission from Nalbandian et al., 1960 (110). c 䉴 Fig. 11. (a) Pulp chamber obliteration in a lower first molar by tertiary dentin formation, as seen in the apical radiograph. The radio-opaque area around the calcifica- tion in the second molar (black arrows), as well as irregular calcification in the distal wall of the second premolar pulp chamber (white arrow), indicate the pres- ence of pulp stones rather than tertiary dentin formation in these teeth. (b) Removal of the filling from the first molar exposed the site of the original pulp chamber, with a clear demarcation line between primary/ secondary dentin and tertiary dentin (arrows). (c) Removal of the tertiary dentin, following the outline of the demarcation line, exposed the pulp chamber floor and allowed instrumentation of the root canals. 15 Tjäderhane et al. dentin formation—as a defensive reaction to increase ity with secondary dentin, while the structure, organi- the dentinal width between the pulp and the oral zation, and mineralization of reparative dentin vary cavity—is “designed” to protect the pulp from exten- significantly. Since reparative dentin is generally atu- sive wear. According to Stanley et al. (106), the preva- bular, it may form a relatively impermeable barrier lence and intensity of tertiary dentin formation under between tubular dentin and pulp tissue. The regularity caries is proposed to be independent of age in humans. of reparative dentin is supposed to be inversely related However, this conclusion can be criticized because the to the degree of irritation (122). group of young teeth in that particular study con- The goal of pulpal treatment procedures aimed at tained only three specimens and the age scale was wide preserving pulp vitality could be the reduction of (11 to 19 years). In young (3 to 8 weeks) rat molars dentin permeability beneath the injury, thus isolating with ongoing primary dentinogenesis, a non-linear the pulp from further irritation. The junction between relationship (123) or even a negative correlation (124) primary and reparative dentin is considered to act as a between dentin formation and extension of dentinal protective barrier against carious stimuli (109,132). It caries has been observed, indicating that the tertiary can, however, also be argued that the complete isola- dentin-like response does not occur in teeth with tion of the pulp by non-tubular reparative dentin is primary dentinogenesis. not necessarily desirable. Pulpal sensory nerve fibers The form and regularity of tertiary dentin depends undergo extensive sprouting in response to injury, and on the intensity and duration of the stimulus. Gener- it is commonly accepted that pulpal nerves are pro- ally, two forms of tertiary dentin are recognized: tective in nature and are involved in the recruitment reactionary dentin (produced by original primary of inflammatory and immunocompetent cells to the odontoblasts) and reparative dentin (produced by injured pulp (133). The degree and state of the newly differentiated replacement odontoblasts) (109, response seem to be highly dependent on the changes 125–128). In addition, it has been suggested that a in dentin permeability. Interestingly, the number of third type of mineralization be distinguished as a dentinal tubules close to the DEJ is significantly merely defensive non-specific production of mineral- higher in the cuspal area than in other parts of dentin ized matrix. This would be produced by so-called (44). Under the cusps, the dentinal tubules are also fibrodentinoblasts, and the product would be called straighter and the odontoblast processes penetrate fibro- or osteodentin (122). In fact, Taintor et al. deeper to the dentin–pulp border (134,135) or even (129) already challenged the term “reparative” dentin to the DEJ (136). Since the cusps are the first area to in 1981. They argued that since repair, by definition, be worn due to abrasion or attrition, it is tempting to consists of the replacement of damaged tissue, the speculate that the reason may be related to the regu- irregular dentin formed in response to external irre- lation of defensive mechanisms in the dentin–pulp versible damage would be comparable to scar tissue complex. The dentinal tubules may be more direct formation (129,130), and should rather be called and odontoblast processes may penetrate more deeply “irritation” or “irritational” dentin (129). While this in order to deliver the message of dental wear and would be, strictly speaking, a more exact term, the induce tertiary dentin formation so as to maintain the present terminology (tertiary dentin and reactionary hard tissue barrier between dental pulp and the oral or reparative dentin) is commonly accepted and is cavity. The coronal dentin–pulp border has other dis- therefore also used in this review. tinctive histological features: a dense innervation of In clinical situations, tertiary dentin usually contains inner dentin and the odontoblast layer (137), pulp atypical fibrodentin, reparative dentin, and reactionary cells producing nerve growth factor and its receptor dentin. Since the criteria for the assessment of differ- (137), and an extremely dense capillary network entiated odontoblast-like cells (or the level of differ- (138,139). The co-localization of these tissue compo- entiation) are not well defined (131), the presence of nents together with the straight tubules and long different types of tertiary dentin at the same site may odontoblast processes may indicate a role in sensing reflect the process of odontoblast-like cell differentia- the external irritation and controlling defensive reac- tion from non-specific, hard-tissue forming cells into tions. The connection of pulpal histological features fully differentiated odontoblast-like cells. In general, with odontoblasts and their processes is at least reactionary dentin has a more or less tubular continu- indirectly supported by the finding that the dense 16 Overview of dentin structure innervations normally seen under the odontoblast vascularity) and mechanical support with a favorable layer (the subodontoblastic plexus of Raschkow) with surface for cell attachment (such as dentin, or suffi- some axons passing into dentinal tubules (7) is not ciently solid calcium hydroxide, or—perhaps even seen in the case of reparative dentin (140). Since the better—MTA in the case of pulp capping) are con- nociceptive fibers contribute to normal homeostatic sidered absolute requirements for appropriate tertiary regulation and vasoregulation and to healing after dentin formation (122). The dentin–pulp complex injury (133,137), this significant reduction in inner- may possess a remarkable capacity to survive even vations may affect the inflammatory and immune intensive dentinal damage, with reactionary and responses under reparative dentin. As well, the initial reparative dentin formation occurring under a re- immunodefensive reaction (measured as the accumu- maining dentin thickness of as thin as 0.5 mm lation of antigen-presenting cells) occurs beneath the (144,145). In the absence of microbial infection, dentinal tubules communicating with superficial caries injuries to the pulp coincident with dentinal injuries lesions (141,142). However, after substantial forma- are presumed to be reversible. After experimental tion of sound reparative dentin, the inflammatory exposure of dentinal tubules, repair and healing of the response to the microbial burden subsides (142,143). pulp occurs in spite of continuous exposure of cut The re-accumulation of antigen-presenting cells dentinal cavities to the salivary microflora (143), sug- occurs only after bacterial invasion of reparative gesting that dentin is able to oppose bacterial threats dentin, close to the pulp (142). We conclude that this even when a small rim (ⱖ1.5 mm) remains. Clinically, supports the concept that the junction between the the dentin–pulp complex is a target for repeated primary and reparative dentin may act as a barrier to microbial, mechanical, and chemical insults such as prevent carious stimuli (109,132). However, even if primary and secondary caries, replacement of restora- reparative dentin prevents microbial component pen- tions, and attrition. Empirical clinical experience has etration into the pulp, it also allows caries progression indicated that the pulpal healing potential is reduced close to the pulp without inducing normal defensive by a repeated series of stimuli. For example, the reactions occurring in the dentin–pulp complex tendency for teeth restored with full crowns (146– (142). Therefore, the formation of reactionary den- 150) or traumatized teeth with pulp canal oblitera- tin with tubular continuity is preferable (122). In tions (151–155) to develop pulpal necrosis has been summary, it must be emphasized that the understand- observed in numerous studies. It is likely that ing of the mechanisms regulating reparative dentin repeated dentinal irritation affects the mechanisms for formation is still limited. Excessive dentin repair may reactionary dentin synthesis and replacement of odon- also be detrimental when the response is not limited toblasts as well as causing pulpal scarring and loss of to the site immediately below the dentinal injury but perivascular stem cells. While there is ample evidence results in generalized root canal system calcification demonstrating increased pulpal cell proliferation, col- (129) (Fig. 11). The decrease of vital pulp tissue may lagenous protein deposition, and reparative dentin reduce the defensive potential of the pulp (for details, formation after a single dentinal injury (cavity prepa- see next paragraph), and obliteration of the root canal ration) (156–163), after a double injury, the increase system certainly makes endodontic procedures more in cell proliferation and collagenous protein deposi- challenging. tion is significantly less than after the single injury The dentin matrix contains biologically active (163). Although the response may have been molecules such as growth factors (for details, see the decreased by pulp–dentin defensive reactions to the article on dentin non-collagenous proteins in the next first injury (such as occlusion of dentinal tubules or issue), which can induce dentinogenic events both in impermeable reactionary dentin formation), the study vivo and in vitro. These dentin matrix bioactive factors still supports the decreased ability of the dentin–pulp are supposed to possess a similar inductive potential complex to respond to repeated insults. The author for tertiary dentin formation as seen by the enamel suggests that the timing of sequential episodes of den- epithelium and basement membrane during physi- tinal irritation could be used to minimize pulpal ological dentinogenesis in embryonic conditions damage after extensive restorative dental treatment (122,128). An appropriate pulpal environment (such (163). However, more research is needed to validate as the absence of severe inflammation and adequate this suggestion. 17 Tjäderhane et al. Root dentin a The epithelial cells of Hertwig’s epithelial root sheath (HERS) initiate odontoblast differentiation very similar to that in the coronal area (7,12). In principle, root dentin forms in a manner similar to coronal dentin, even though some differences may exist. For example, the coarse fibrils of mantle dentin at the cementum– dentin junction (CDJ) are usually parallel to the basal lamina, not perpendicular as often seen in the crown (25). The terminology and even the structure of root mantle dentin are also controversial: it has been called the “hyaline layer” and reported to be absent in some animals and to vary in thickness in others (25). Struc- turally, the human CDJ represents a region of inter- spaced 15 to 30 mm-wide collagen fibril bridges b (25,164) formed after the breakdown of HERS (165). In the cementum, the collagen fibril bridges from the dentin intermingle with collagen fibers parallel to the root surface, ensuring a tight attachment of cementum to the dentin (Fig. 12). The CDJ also contains pores, possibly representing HERS remnants (164). The granular layer of Tomes is located in the outermost part of the root dentin. It is believed to represent the mantle dentin in the root surface or be located immediately below the root mantle dentin (25). Similar to coronal mantle dentin, it also displays thin canaliculi and poorly fused globules (12,25). However, the granular struc- tures can only be seen in ground sections, not in c histological staining or electron microradiographs (7). Most likely they represent the mineralization pattern in the initial phases of root dentin and cementum forma- tion. The density of tubules in root dentin is still a somewhat controversial issue. Some studies have indi- cated a rather moderate decrease in tubular density from the cemento–enamel junction (CEJ) toward the apex (44,166) while others have indicated a more pronounced decrease (167). The reason for the differ- ences may be the different methods or different teeth used for the analyses, as Schellenberg et al. (167) found markedly fewer tubules in mesio-distal than bucco- lingual surfaces of premolars, but not in third molars. The main part of the root dentin is rich with both fine Fig. 12. FE-SEM images of human cement–dentinal tubular branches and microbranches, with occasional junction (CDJ). (a) 10 to 15 mm cementum layer in intimate contact with dentin. Magnification = 500 ¥; major branching (Fig. 13) (44). bar = 10 mm. (b,c) Higher magnification demonstrates the mineralized collagen fiber continuity from cemen- Apical dentin tum to underlying dentin. Magnifications = 2,500 ¥ (b) and 5,000 ¥ (c); Bars = 10 mm (b) and 1 mm (c). It has been suggested that the dentin in the most apical part of the roots differs from the rest of the 18 Overview of dentin structure dentin also differs in tertiary dentin formation (168). Since there should not be external irritation causing the formation of reparative dentin, the dentin formed after primary dentinogenesis should, by definition, be secondary dentin. However, a distinct border a between primary and “secondary” dentin, with dis- continuity of dentinal tubules, has been demon- strated. The apical portion of human teeth also shows other marked variations in structure, such as acces- sory root canals, areas of resorption and repaired resorption, and cementum-like tissue lining the apical root canal wall. The causative factors for these kinds b of dentin formation could be, for example, pulp inflammation or a response to occlusal loading. In addition, age-related root tubular sclerosis that starts in the third decade of life from the apical region and advances coronally (111) has recently been suggested to be the main factor influencing the permeability of root dentin (170,171) (Fig. 14). Root dentin seems to have regional differences in its permeability: buccal and lingual curvatures of c root canals show patent tubules that take up dyes, while the mesial and distal pulpal borders seem to be occluded with minerals (170,171) (Fig. 15). One wonders how this pattern of tubule patency corre- sponds to local stress distributions in the functioning of these roots, and whether these stress distributions translate into regional differences in dentinal fluid shifts (172). Pulp stones d Pulp stones are discrete or diffuse pulp calcifications Fig. 13. Dentinal tubule branching in root dentin. (a) that can be classified structurally as well as based on Typical major branching, with numerous fine branches location (173). Structurally, there are “true” and (Fb). (b) The dentinal tubule in the center has numerous fine branches, giving it the appearance of an interdental “false” pulp stones, the distinction being morphologi- toothbrush. H-E staining of a premolar from a 12-year- cal. A third type, “diffuse” or “amorphous” pulp old. (c) Dentinal tubules in cervical area showing varia- stones, are more irregular in shape than false pulp tions of fine branches from the same tooth as in (b). (d) stones and occur in close association with blood vessels Variation in branching of dentinal tubules in root dentin. Hematoxylin-eosin (H-E) staining (a–c); Masson (174). The cells forming the pulp stones may also vary, staining (d). Reproduced with permission from Mjör & as “true” pulp stones contain dentinal tubules and are Nordahl, 1996 (44). lined with odontoblasts (or rather odontoblast-like cells), while “false” pulp stones with atubular calcifi- cation have been considered to be formed from degen- root dentin in many respects. The number and erating cells of the pulp that mineralize. The regularity of tubules markedly decreases in the apical distinction between the “true” and “false” pulp stones root (44,166,168,169). Apical primary dentinal may be somewhat artificial, as both tubular and atu- tubules may have an irregular direction and density, bular dentin can be found in a single pulp stone and in some areas they are missing (168,169). Apical (Fig. 16). 19 Tjäderhane et al. Fig. 14. Relative mean dye penetration (in percentage of complete dentin area) after extensive (60 day) incubation of Methylene blue in instrumented root canals. Data are from Thaler et al., 2008 (171). Fig. 15. (a) A light microscope view of a cross-section of a human tooth, showing the typical bucco-lingual barbell shape and dye penetration pattern of Patent blue. Magnification = 16 ¥. (b–d) Back-scattered electron micrograph of areas with (b) and without (c,d) dye penetration, demonstrating patent dentinal tubules in (b) and tubular sclerosis in (c,d). Magnifications = 1,000 ¥ (b,c); 3,000 ¥ (d). Reproduced with permission from Paqué et al., 2006 (170). 20 Overview of dentin structure a b c d e f Fig. 16. Heterogenous structure of pulp stone. (a) Pulp stone in human dental pulp, stained with hematoxylin-eosin staining. The pulp stone appears as a solid calcified mass. The width of the stone is approximately 3 mm. Original magnification = 50 ¥. (b) Adjacent section stained with Toluidine blue. The pulp stone appears much more heterog- enous. Original magnification = 50 ¥. (c) Higher magnification of the lower left corner of (a). Odontoblast-like cells line the lower border of the pulp stone, while the right side is devoid of odontoblast-like cells. Original magnifica- tion = 100 ¥. (d) Same area stained with Toluidine blue demonstrates tubular structure in the pulp stone at the site of the odontoblast-like cells, while the area without cells is essentially free of tubules. Original magnification = 100 ¥. (e) Higher magnification of (d). Well-formed longitudinally cut tubules are on the left side, while the tubules on the right side are cut across the tubule direction and appear less organized. Original magnification = 200 ¥. (f) In another part of the pulp stone, tubules appear more sparse and with numerous fine branches and microbranches. Original magnification = 400 ¥. 21 Tjäderhane et al. a b c d Fig. 17. Pulp stones. (a) Pulp stone obliterating most of the coronal pulp chamber in a lower molar. (b) Higher magnification of (a). The pulp chamber contains both loose pulp stone “a” originally surrounded by pulpal tissue, and pulp stone attached to the pulp chamber wall “b”. (c) Pulp stone filling a premolar pulp chamber. The uneven form of the pulp stone indicates that several pulp stones have developed independently and grown in size until being united. (d) The size of the pulp stone presented in (a) and (b), with some necrotic pulp tissue still attached to the stone. It must be noted that some material was lost as the pulp stone had to be drilled out of the pulp chamber. Therefore, the original size of the stone may have been even larger. A single tooth may contain one or several pulp Words of caution: dentin stones of varying size, most often found in coronal differences between species pulp but sometimes also present in radicular pulp. Despite a number of studies, the exact cause of such It is important to realize that differences in the struc- pulp calcifications remains largely unknown. External ture and chemistry of dentin between different irritation (caries, attrition) has been suggested as a species have long been recognized (12). For cause, but pulp stones also appear in teeth with no example, the cellular junctions between odontoblasts, apparent cause (e.g. impacted third molars). Pulp the amount of peritubular matrix, the structure and stones have also been noted in relation to systemic or mechanism of formation of peritubular dentin, genetic diseases, including dentin dysplasia [especially and the presence and thickness of root mantle dentin dentin dysplasia type II (175,176)], and certain syn- vary between species (25). These differences may dromes (176). Large pulp stones in the pulp chamber affect the usability of certain animal models. Because may obstruct the canal orifices (Fig. 17), and in the many (maybe most) biochemical studies have been root canal they may complicate access to the apical performed on bovine and rat dentin, available data canal. For a comprehensive description of pulp stones, often directly concern only these species. In general, the reader is referred to a recent review article by Goga in terms of protein composition of the organic et al. (176). matrix, dentin species can be divided into two main 22 Overview of dentin structure groups: (a) continuously growing rodent teeth; ondary cementogenesis continues throughout life in and (b) teeth with limited primary dentinogenesis order to keep the tooth functional in spite of attrition (such as bovine, porcine, rat molar, and human (180). Therefore, rodent (especially rat) molars have dentin). Therefore, care must be exercised when been extensively used for caries research (181), includ- extrapolating findings from one species to another ing the response of the dentin–pulp complex to exter- (12,25). nal irritation. Mouse models (both in vivo and developing tooth Bovine teeth have been frequently used in in vitro organ cultures) have been used to study tooth devel- and in situ experiments to study mechanical proper- opment and abnormalities [reviewed by Fleischman- ties and to simulate human tooth behavior. Bovine nova et al. (177)], and transgenic and gene knockout teeth have been extensively used in studies involving techniques have allowed more sophisticated methods dental erosion and abrasion (182–185). Bovine of studying the roles of single gene products on tooth coronal dentin is similar to human dentin with morphogenesis and development. Even though these respect to the number and diameter of tubules (186) models have occasionally led to breakthrough discov- and hardness (187), but has a slightly higher radi- eries of the role and interference of a single protein in odensity, possibly due to the greater amount of peri- tooth development, in many cases the effects of a tubular dentin in bovine teeth in age-related samples single gene deletion are minor or non-existent. This (187). The use of bovine incisors as substitutes for demonstrates the presence of “back-up systems” that human teeth in studies involving erosion/abrasion is are able to compensate for the function of a defective well accepted because dentin wear in human third gene. molars and bovine incisors is quite similar (188). In research on mineralized tissues, the rat has long Bovine dentin close to the enamel–cement junction is been considered as a particularly good experimental a viable substitute for human dentin in in vitro den- model (178). The same mechanisms control gains and tin permeability experiments (189). Bovine incisors losses in bone mass in young and aged rats and can also be used as substitutes for human teeth in humans. The rat model is not as time-consuming as immediate composite adhesive bond strength tests those of larger animals. Osteopenic and osteoporotic (190,191). changes in rat bones copy the human forms. Mechani- cal usage and drug effects as well as bone healing can easily be studied on rats (178). Rodent incisors grow Concluding remarks continuously and therefore the entire sequence of enamel and dentin development, including the lifecy- Unlike bone, dentin does not turn over. That is, its cle of the amelo- and odontoblasts, may be studied in external surface is far removed from the cells which one tooth. Continuously growing teeth, however, are produced it developmentally, precluding coronal disadvantageous when extrinsic factors affecting the dentin repair. Unlike bone, dentin functions in a tissues are studied. In rat incisors, only intertubular septic, hostile environment with great temperature but not peritubular dentin is present (12). The main and chemical challenges. It has evolved into a dynamic regulatory factor in dentinogenesis and odontogenesis tissue that is able, within biological limits, to react to in rat incisors is the attrition rate (179). Because of the environmental stresses in a protective, reactive manner. constant wear of incisors, enamel and dentin are con- The microscopic nature of the dentin–enamel and stantly renewed and therefore incisors do not provide cemento–dentin junctions has delayed the study of a permanent record of mineral metabolism in dentin their mechanical and fracture properties as well as their (53). Also, caries does not occur in rodent incisors biochemical composition. The recent application of, and therefore caries experiments are impossible in rat for example, atomic force microscopy, nanoDynamic incisors. Mechanical Analysis (nanoDMA), patch-clamp elec- Rodent molars, however, are in many ways similar to trophysiology, and molecular biology probes holds human molars. The crown is multi-cuspal and fissures great promise for a new understanding of how dentin bear a great similarity to those in human molars. The responds to various stimuli. Hopefully this will lead to rat molar has a limited growth and enamel and dentin therapeutic advances in Operative Dentistry and formation resembles that in humans, even though sec- Endodontics. 23 Tjäderhane et al. References 18. Whittaker DK. The enamel–dentine junction of human and Macaca irus teeth: a light and electron 1. Ørvig T. Phylogeny of tooth tissues: evolution of some microscopic study. J Anat 1978: 125: 323–335. calcified tissues in early vertebrates. In: Miles AEW, ed. 19. Habelitz S, Marshall SJ, Marshall GW Jr, Balooch M. Structural and Chemical Organization of Teeth. Aca- The functional width of the dentino–enamel junction demic Press, 1967: 45–110. determined by AFM-based nanoscratching. J Struct 2. Urist MR, Mizutani H, Conover MA, Lietze A, Fin- Biol 2001: 135: 294–301. erman GAM. Dentin, bone, and osteosarcoma tissue 20. Oliveira CA, Bergqvist LP, Line SR. A comparative bone morphogenetic proteins. In: Dixon AD, Sarnat analysis of the structure of the dentino–enamel junc- BG, eds. Factors and Mechanisms Influencing Bone tion in mammals. J Oral Sci 2001: 43: 277–281. Growth. Alan R. Liss, Inc., 1982: 61–81. 21. Marshall SJ, Balooch M, Habelitz S, Balooch G, 3. Smith MM, Hall BK. Development and evolutionary Gallagher R, Marshall GW. The dentino—enamel origins of vertebrate skeletogenic and odontogenic junction—a natural, multilevel interface. J Eur Ceram tissues. Biol Rev Camb Philos Soc 1990: 65: 277–373. Soc 2003: 23: 2897–2904. 4. Sire JY, Huysseune A. Formation of dermal skeletal 22. Radlanski RJ, Renz H. Developmental movements of and dental tissues in fish: a comparative and evolution- the inner enamel epithelium as derived from micro- ary approach. Biol Rev Camb Philos Soc 2003: 78: morphological features. Eur J Oral Sci 2006: 114: 219–249. 1–6. 5. Donoghue PC, Sansom IJ, Downs JP. Early evolution 23. Radlanski RJ, Renz H. Insular dentin formation of vertebrate skeletal tissues and cellular interactions, pattern in human odontogenesis in relation to the and the canalization of skeletal development. J Exp scalloped dentino–enamel junction. Ann Anat 2007: Zool B Mol Dev Evol 2006: 306: 278–294. 189: 243–250. 6. Kawasaki K, Weiss KM. SCPP gene evolution and the 24. Goel VK, Khera SC, Ralston JL, Chang KH. Stresses at dental mineralization continuum. J Dent Res 2008: the dentino–enamel junction of human teeth—a finite 87: 520–531. element investigation. J Prosthet Dent 1991: 66: 451– 7. Nanci A. Dentin-pulp complex. In: Nanci A, ed. Ten 459. Cate’s Oral Histology, 7th edn. Mosby Elsevier, 2008: 25. Jones SJ, Boyde A. Ultrastructure of dentin and den- 191–238. tinogenesis. In: Linde A, ed. Dentin and Dentinogen- 8. Pashley DH. Dynamics of the pulpo–dentin complex. esis, Volume I. CRC Press, 1984: 81–134. Crit Rev Oral Biol Med 1996: 7: 104–133. 26. Arsenault AL, Robinson BW. The dentino–enamel 9. He LH, Swain MV. Enamel—a “metallic-like” junction: a structural and microanalytical study of early deformable biocomposite. J Dent 2007: 35: 431–437. mineralization. Calcif Tissue Int 1989: 45: 111–121. 10. Bajaj D, Arola D. Role of prism decussation on fatigue 27. Hayashi Y. High resolution electron microscopy in the crack growth and fracture of human enamel. Acta dentino–enamel junction. J Electron Micros 1992: 41: Biomater 2009: 8: 3045–3056. 387–391. 11. Xie Z, Swain MV, Hoffman MJ. Structural integrity of 28. Diekwisch TG, Berman BJ, Genters S, Slavkin HC. enamel: experimental and modeling. J Dent Res 2009: Initial enamel crystals are not spatially associated with 88: 529–533. mineralized dentin. Cell Tissue Res 1998: 279: 149– 12. Linde A, Goldberg M. Dentinogenesis. Crit Rev Oral 167. Biol Med 1993: 4: 679–728. 29. Takano Y, Hanaizumi Y, Oshima H. Occurrence of 13. Torneck CD. Dentin–pulp complex. In: Ten Cate AR, amorphous and crystalline mineral deposits at the ed. Oral Histology. Development, Structure and Func- epithelial–mesenchymal interface of incisors in the tion, 4th edn. Mosby, 1994: 169–217. calcium-loaded rat: implication of novel calcium 14. Cox CF, White KC, Ramus DL, Farmer JB, Milner binding domains. Anat Rec 1996: 245: 174–185. Snuggs H. Reparative dentin: factors affecting its 30. Dong W, Warshawsky H. Lattice fringe continuity in deposition. Quintessence Int 1992: 23: 257–270. the absence of crystal continuity in enamel. Adv Dent 15. Gallagher RR, Demos SG, Balooch M, Marshall GW Res 1996: 10: 232–237. Jr, Marshall SJ. Optical spectroscopy and imaging of 31. Bodier-Houllé P, Steuer P, Meyer JM, Bigeard L, the dentin–enamel junction in human third molars. Cuisinier FJ. High-resolution electron-microscopic J Biomed Mater Res A 2003: 64: 372–377. study of the relationship between human enamel and 16. Goldberg M, Septier D, Bourd K, Hall R, Jeanny JC, dentin crystals at the dentino–enamel junction. Cell Jonet L, Colin S, Tager F, Chaussain-Miller C, Gara- Tissue Res 2000: 301: 389–395. bédian M, George A, Goldberg H, Menashi S. The 32. Lin CP, Douglas WH, Erlandsen SL. Scanning elec- dentino–enamel junction revisited. Connect Tissue Res tron microscopy of type I collagen at the dentin– 2002: 43: 482–489. enamel junction of human teeth. J Histochem Cytochem 17. Boushell LW, Kaku M, Mochida Y, Bagnell R, 1993: 41: 381–388. Yamauchi M. Immunohistochemical localization of 33. Ohsaki Y, Nagata K. Type III collagen is a major com- matrix metalloproteinase-2 in human coronal dentin. ponent of interodontoblastic fibers of the developing Arch Oral Biol 2008: 53: 109–116. mouse molar root. Anat Rec 1994: 240: 308–313. 24 Overview of dentin structure 34. Nakamura O, Gohda E, Ozawa M, Senba I, Daikuhara dentin and enamel in crowns of human teeth: insight Y. Immunohistochemical studies with a monoclonal into tooth function. J Struct Biol 2006: 153: 188–199. antibody on the distribution of phosphophoryn in pre- 50. Romagnoli P, Mancini G, Galeotti F, Francini F, Pier- dentin and dentin. Calcif Tissue Int 1985: 37: 491– leoni P. The crown odontoblasts of rat molars from 500. primary dentinogenesis to complete eruption. J Dent 35. Takagi Y, Fujisawa R, Sasaki S. Identification of dentin Res 1990: 69: 1857–1862. phosphophoryn localization by histochemical stain- 51. Linde A. Structure and calcification of dentin. In: ings. Connect Tissue Res 1986: 14: 279–292. Bonucci E, ed. Calcification in Biological Systems. CRC 36. Wang RZ, Weiner S. Strain–structure relations in Press, 1992: 269–311. human teeth using Moiré fringes. J Biomech 1998: 31: 52. Ten Cate AR. Physiological tooth movement: eruption 135–141. and shedding. In: Ten Cate AR, ed. Oral Histology. 37. Tesch W, Eidelman N, Roschger P, Goldenberg F, Development, Structure and Function, 4th edn. Mosby, Klaushofer K, Fratzl P. Graded microstructure and 1994: 313–341. mechanical properties of human crown dentin. Calcif 53. Johannessen LB. Dentine apposition in the mandibu- Tissue Int 2001: 69: 147–157. lar first molars of albino rats. Arch Oral Biol 1961: 5: 38. Tjäderhane L, Hietala E-L, Larmas M. Mineral 81–91. element analyses of carious and intact dentin by elec- 54. Hietala E-L, Larmas M. The effect of ovariectomy on tron probe microanalyser combined with back- dentin formation and caries in adult rats. Acta Odontol scattered electron image. J Dent Res 1995: 74: 1770– Scand 1992: 50: 337–343. 1774. 55. Kortelainen S, Larmas M. Effect of fluoride on the rate 39. Kinney JH, Balooch M, Marshall SJ, Marshall GW Jr, of dentin apposition and caries progression in young Weihs TP. Hardness and Young’s modulus of human and old Wistar rats. Scand J Dent Res 1994: 102: peritubular and intertubular dentine. Arch Oral Biol 30–33. 1996: 41: 9–13. 56. Simon S, Smith AJ, Lumley PJ, Berdal A, Smith G, 40. Vasilakis GJ, Nygaard VK, DiPalma DM. Vitamin D Finney S, Cooper PR. Molecular characterization of resistant rickets. A review and case report of an ado- young and mature odontoblasts. Bone 2009: 45: 693– lescent boy with a history of dental problems. J Oral 703. Med 1980: 35: 19–26. 57. Veis A, Schlueter RJ. The macromolecular organiza- 41. Shellis RP. Structural organization of calcospherites in tion of dentine matrix collagen. I. Characterization of normal and rachitic human dentine. Arch Oral Biol dentin collagen. Biochemistry 1964: 3: 1650–1657. 1983: 28: 85–95. 58. Schlueter RJ, Veis A. The macromolecular organiza- 42. Hietala E-L, Larmas M. Mineral content of different tion of dentin matrix collagen. II. Periodate degrada- areas of human dentin in hypophosphataemic vitamin tion and carbohydrate cross-linking. Biochemistry D-resistent rickets. J Biol Buccale 1991: 19: 129– 1964: 3: 1657–1665. 134. 59. Gage JP. Electrophoretic characterization of peptides 43. Larmas M, Hietala EL, Similä S, Pajari U. Oral mani- from normal mature human dentine. Arch Oral Biol festations of familial hypophosphatemic rickets after 1984: 29: 575–580. phosphate supplement therapy: a review of the litera- 60. Butler WT. Dentin collagen: chemical structure and ture and report of case. ASDC J Dent Child 1991: 58: role in mineralization. In: Linde A, ed. Dentin and 328–334. Dentinogenesis, Volume II. CRC Press, 1984: 37–53. 44. Mjör IA, Nordahl I. The density and branching of 61. Munksgaard EC, Rhodes M, Mayne R, Butler WT. dentinal tubules in human teeth. Arch Oral Biol 1996: Collagen synthesis and secretion by rat incisor odon- 41: 401–412. toblasts in organ culture. Eur J Biochem 1978: 82: 45. Sognnaes RF, Shaw JH, Bogoroch R. Radiotracer 609–617. studies on bone, cementum, dentin and enamel of 62. Munksgaard EC, Moe D. Types of collagen in an Rhesus monkeys. Am J Physiol 1955: 180: 408–420. extract of odontoblasts and dentine from developing 46. Byers MR, Lin KJ. Patterns of fluoro-gold entry into bovine teeth. Arch Oral Biol 1980: 25: 485–489. rat molar enamel, dentin, and pulp. J Dent Res 2003: 63. Sodek J, Mandell SM. Collagen metabolism in rat 82: 312–317. incisor predentine in vivo: synthesis and maturation of 47. Ikeda H, Suda H. Rapid penetration of lucifer yellow type I, alpha 1 (I) trimer, and type V collagens. Bio- into vital teeth and dye coupling between odontoblasts chemistry 1982: 21: 2011–2015. and neighbouring pulp cells in the cat. Arch Oral Biol 64. Butler WT, Ritchie H. The nature and functional sig- 2006: 51: 123–128. nificance of dentin extracellular matrix proteins. Int J 48. Wood JD, Wang R, Weiner S, Pashley DH. Mapping of Dev Biol 1995: 39: 169–179. tooth deformation caused by moisture change using 65. Wohllebe M, Carmichael DJ. Type-I trimer and type-I moiré interferometry. Dent Mater 2003: 19: 159– collagen in neutral-salt-soluble lathyritic-rat dentine. 166. Eur J Biochem 1978: 92: 183–188. 49. Zaslansky P, Friesem AA, Weiner S. Structure and 66. Wohllebe M, Carmichael DJ. Biochemical characteri- mechanical properties of the soft zone separating bulk zation of guanidinium chloride-soluble dentine 25 Tjäderhane et al. collagen from lathyritic-rat incisors. Biochem J 1979: 79. Karjalainen S, Söderling E, Pelliniemi L, Foidart JM. 181: 667–676. Immunohistochemical localization of types I and III 67. Lukinmaa PL, Vaahtokari A, Vainio S, Sandberg M, collagen and fibronectin in the dentine of carious Waltimo J, Thesleff I. Transient expression of type III human teeth. Arch Oral Biol 1986: 31: 801–806. collagen by odontoblasts: developmental changes in 80. Magloire H, Joffre A, Hartman DJ. Localization and the distribution of pro-alpha 1(III) and pro-alpha 1(I) synthesis of type III collagen and fibronectin in human collagen mRNAs in dental tissues. Matrix 1993: 13: reparative dentine. Immunoperoxidase and immuno- 503–515. gold staining. Histochemistry 1988: 88: 141–149. 68. Palosaari H, Tasanen K, Risteli J, Larmas M, Salo T, 81. Zaslansky P, Zabler S, Fratzl P. 3D variations in human Tjäderhane L. Baseline expression and effect of TGF- crown dentin tubule orientation: a phase-contrast beta 1 on type I and III collagen mRNA and protein microtomography study. Dent Mater 2010: 26: synthesis in human odontoblasts and pulp cells in e1–10. vitro. Calcif Tissue Int 2001: 68: 122–129. 82. Kagayama M, Sasano Y, Sato H, Kamakura S, Motegi 69. Becker J, Schuppan D, Benzian H, Bals T, Hahn EG, K, Mizoguchi I. Confocal microscopy of dentinal Cantaluppi C, Reichart P. Immunohistochemical tubules in human tooth stained with Alizarin red. Anat distribution of collagens types IV, V and VI and of Embryol 1999: 199: 233–238. pro-collagen types I and III in human alveolar bone 83. Lu Y, Xie Y, Zhang S, Dusevich V, Bonewald LF, Feng and dentine. J Histochem Cytochem 1986: 34: 1417– JQ. DMP1-targeted Cre expression in odontoblasts 1429. and osteocytes. J Dent Res 2007: 86: 320–325. 70. Nagata K, Huang YH, Ohsaki Y, Kukita T, Nakata M, 84. Gotliv BA, Veis A. Peritubular dentin, a vertebrate Kurisu K. Demonstration of type III collagen in the apatitic mineralized tissue without collagen: role of a dentin of mice. Matrix 1992: 12: 448–455. phospholipid–proteolipid complex. Calcif Tissue Int 71. Waltimo J, Risteli L, Risteli J, Lukinmaa PL. Altered 2007: 81: 191–205. collagen expression in human dentin: increased reac- 85. Lester KS, Boyde A. The surface morphology of some tivity of type III and presence of type VI in dentino- crystalline components of dentin. In: Symons NB, ed. genesis imperfecta, as revealed by immunoelectron Dentine and Pulp. Williams and Wilkins, 1968: 197– microscopy. J Histochem Cytochem 1994: 42: 1593– 219. 1601. 86. Schroeder L, Frank RM. High resolution transmission 72. Orsini G, Ruggeri A Jr, Mazzoni A, Papa V, Piccirilli electron microscopy of adult human peritubular M, Falconi M, Di Lenarda R, Breschi L. Immunohis- dentine. Cell Tissue Res 1985: 242: 449–451. tochemical identification of type I and type III colla- 87. Hirayama A. Experimental analytical electron micro- gen and chondroitin sulphate in human pre-dentine: a scopic studies on the quantitative analysis of elemental correlative FEI-SEM/TEM study. Int Endod J 2007: concentrations in biological thin specimens and its 40: 669–678. application to dental science. Shikawa Gakuho 1990: 73. Bronckers AL, Gay S, Lyaruu DM, Gay RE, Miller EJ. 90: 1019–1036. Localization of type V collagen with monoclonal anti- 88. Weiner S, Veis A, Beniash E, Arad T, Dillon JW, Sabsay bodies in developing dental and peridental tissues of B, Siddiqui F. Peritubular dentin formation: crystal the rat and hamster. Coll Relat Res 1986: 6: 1–13. organization and the macromolecular constituents in 74. Lukinmaa PL, Waltimo J. Immunohistochemical human teeth. J Struct Biol 1999: 126: 27–41. localization of types I, V, and VI collagen in human 89. Magne D, Guicheux J, Weiss P, Pilet P, Daculsi G. permanent teeth and periodontal ligament. J Dent Res Fourier transform infrared microspectroscopic investi- 1992: 71: 391–397. gation of the organic and mineral constituents of peri- 75. Palosaari H, Pennington CJ, Larmas M, Edwards DR, tubular dentin: a horse stud