Cytoplasmic Inheritance PDF
Document Details
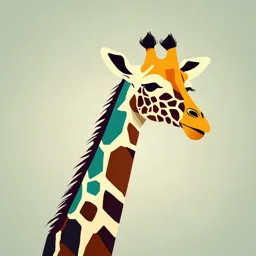
Uploaded by SwiftClavichord
PAU S.S.S. School
Tags
Summary
This document describes cytoplasmic inheritance, a type of non-Mendelian inheritance where traits are passed down through the cytoplasm instead of chromosomes. It covers historical aspects, definitions, and characteristics related to this form of inheritance, focusing on examples and mechanisms seen in plants and animals.
Full Transcript
# Chapter 24 Cytoplasmic Inheritance ## 24.1. Introduction - Inheritance of most of the characters in eukaryotic organisms has the following characteristic features: - The contributions by both male and female parents are equal, - Segregation produces the characteristic 3:1, 1:2:1, 9:3:3:1,...
# Chapter 24 Cytoplasmic Inheritance ## 24.1. Introduction - Inheritance of most of the characters in eukaryotic organisms has the following characteristic features: - The contributions by both male and female parents are equal, - Segregation produces the characteristic 3:1, 1:2:1, 9:3:3:1, etc. ratios. - This inheritance pattern is referred to as Mendelian inheritance. - It is universally accepted that genes are located in the chromosomes of eukaryotic nuclei. - It was consequently demonstrated by Mendel that genes showing Mendelian inheritance pattern, and those showing characters in several organisms do not show Mendelian inheritance pattern, and show the following characteristic features: - There is consistent difference between the results shown in reciprocal crosses; generally, only the trait from the female parent is transmitted. - In most cases, there is no segregation in the F₂ and the subsequent generations. - In many cases, there is some segregation. - Characters showing nonmendelian inheritance may be grouped into the following three broad categories: - Those related to cellular structures and patterns. - Those produced by intracellular parasites, symbionts and viruses. - Those associated with DNA containing cell organelles, i.e., mitochondria and chloroplasts. - The transmission of structures and patterns, e.g., centriole, cell wall biological membranes, etc., involves only facilitation of self-alignment and self-assembly of the concerned monomers, and none of these structures contains DNA. Therefore, these cases cannot be considered as cases of inheritance. The real cases of cytoplasmic inheritance are due to the DNA present in mitochondria and chloroplasts. ## 24.2. Historical - The evidence for cytoplasmic inheritance was first presented by Correns in *Mirabilis Jalapa* and by Baur in *Pelargonium zonale* in 1908, and they termed this as plastid inheritance. - In 1924, Jenkins described the *iojap* leaf variegation in maize, which was later investigated in considerable detail by Rhoades. - Thus, there was some evidence that, at least in plants, the cytoplasm does play a genetic role. - But the examples of such a role were so few that in 1926 Morgan concluded that the cytoplasm "may be ignored genetically." - Rhoades described cytoplasmic male sterility in maize in 1933. - Ten years later, in 1943, Sonneborn discovered kappa particles in *Paramecium*, and described its cytoplasmic transmission. - The presence of DNA in chloroplasts was first demonstrated by Ris and Plaut in 1962 through electron microscopy. - One year later, in 1963, Ris and Plaut demonstrated the existence of DNA in mitochondria through electron microscopy coupled with cytochemical techniques. - In 1954, Ruth Sager described cytoplasmic inheritance (Section 24.7.3). - She showed that 1% of zygotes were exceptional in that they were heterozygous for cytoplasmic genes, i.e., they showed biparental inheritance. ## 24.3. Definitions - In cases of cytoplasmic inheritance, generally the character of only one of the two parents (usually the female parent) is transmitted to progeny. - Such inheritance is also referred to as extranuclear inheritance, extrachromosomal inheritance and maternal inheritance. - Since the term cytoplasmic inheritance is more commonly used, it is preferred to the other terms in this text. - Genes governing the traits showing cytoplasmic inheritance are located outside the nucleus and in the cytoplasm, and are referred to as plasmagenes, cytoplasmic genes, cytogenes, extranuclear genes or extrachromosomal genes. - All the genes present in the cytoplasm of a cell is known as plasmon. - All the genes present in a plastid constitute a plastom, while chondriom denotes all the genes present in mitochondria. - Individuals heterozygous for plasmagenes are referred to as cytohets (from cytoplasmic heterozygotes). - Thus, cytoplasmic inheritance arises due to the plasmagenes located in cell organelles (plastids and mitochondria) that are integral constituents of normal cells. ## 24.4. Characteristics Of Cytoplasmic Inheritance 1. **Reciprocal Differences:** Reciprocal crosses show the same inherited characters governed by cytoplasmic inheritance. Generally the female parent is the only one that transmits characters. 2. **Lack of Segregation:** In general, F₂ and the subsequent generations do not show regular segregation of the cytoplasmically inherited trait. This is because the F₁ individuals generally receive plasmagenes from one parent only. 3. **Irregular Segregation in Biparental Inheritance:** In some cases, plasmagenes from both the parents are transmitted to progeny; this is the biparental inheritance. Biparental inheritance gives rise to irregular segregation ratios in the F₁ generation of higher plants. But in *Chlamydomonas*, often zoospores show 1:1 ratio for the alleles of a plasmagene, but only after they have undergone several mitotic divisions. 4. **Somatic Segregation:** Plasmagenes generally show segregation during mitosis, a feature of rare occurrence in the case of nuclear genes. 5. **Association with Organellar DNA:** Several plasmagenes have been shown to be associated with cpDNA or mtDNA. The demonstration of an association of a plasmagene with organellar DNA is a definite evidence for the trait produced by the former to be cytoplasmically inherited. 6. **Nuclear Transplantation:** If nuclear transplantation reveals a trait to be governed by the genotype of cytoplasm and not by that of nucleus, cytoplasmic inheritance of the trait is strongly indicated. In nuclear transplantation, nucleus of a cell is removed and replaced by a nucleus of another genotype taken from a different cell. 7. **Transfer of Nuclear Genome through Backcrosses:** The nucleus of a variety of species may be transferred into the cytoplasm of another species of variety through repeated backcrossing with the former; the donor of nucleus is used as recurrent male parent. Lines produced in this way are known as alloplasmic lines since they have nuclei and cytoplasms from different species. A comparison of the various characters of alloplasmic lines with those of the corresponding euplasmic lines (lines having nuclei and cytoplasms from the same species) demonstrates cytoplasmic effects, if any, on these traits. 8. **Mutagenesis:** Some mutagens, e.g., ethidium bromide, are highly specific mutagens for plasmagenes, while nuclear genes are not affected by them. Induction of mutation by such agents in a gene indicates it to be a plasmagene. 9. **Lack of Chromosomal Location:** In many organisms, extensive linkage maps of nuclear genes are available. If a gene is shown to be located in one of these linkage groups, it cannot be a plasmagene. 10. **Lack of Association with a Parasite, Symbiont or Virus:** In many cases, a cytoplasmically inherited character is associated with a parasite, symbiont or virus present in the cytoplasm of the organism. Such cases cannot be regarded as real cases of cytoplasmic inheritance. Only those cytoplasmically inherited characters, which are not associated with parasites, symbionts or viruses can be regarded as governed by plasmagenes. ## 24.5. Plastid Inheritance - The inheritance pattern for characters due to plasmagenes located in plastids is known as plastid inheritance. - The first case of cytoplasmic inheritance to be discovered was made independently by Correns and Baur in 1908. - A common case of cytoplasmic inheritance is restriction of chlorophyll variegation (Fig 24.2). - But recent studies have used restriction fragment length polymorphism and transmission electron microscopy to follow plastid inheritance. - Chlorophyll variegation may be produced by: - Some environmental factors, - Some nuclear genes, and - Some plasmagenes. ### 24.5.1. *Mirabilis Jalapa* - Leaves of *Mirabilis Jalapa*, the four o'clock plant, may be green, white or variegated, and some branches may have only green, only white or only variegated leaves. - Correns made reciprocal crosses in all combinations among the flowers produced on the three types of branches; results from these crosses are summarized in Table 24.1. - When flowers from a green branch were used as female; all the progeny were green irrespective of the phenotype (green, white or variegated) of the branch used as male parent. - Similarly, progeny from the crosses involving flowers from white branches are the female parent were all white irrespective of the male flowers being from green, white or variegated branches. - But in the progeny from all the crosses involving flowers from variegated branches as the female parent, green, white and variegated plants were recovered in variable proportions. - Clearly, the phenotype of progeny is the same as that of the female parent, except when the female parent is variegated. - These results may be explained by assuming that the plasmagene producing variegation in *M. jalapa* is located in plastids, an assumption made by Correns himself. - It is now known that chloroplasts differentiate from proplastids and their differentiation is affected by both nuclear and plasmagenes. - Proplastids having the mutant plasmagene develop into abnormal chloroplasts, while those having the normal allele develop into normal green chloroplasts. - The maternal transmission of plasmagenes in higher plants and animals is explained on the basis of unequal contribution by male and female gametes to the cytoplasm, of the zygote. - In general, egg/egg cell has a considerable amount of cytoplasm, which contains several mitochondria and proplastids. - On the other hand, male gametes or sperms may contribute little or no mitochondria and plastids. - Several mechanisms operate to ensure maternal transmission of these organelles. - The generative cell produced by the first pollen mitosis ordinarily receives no plastids, but it nearly always contains numerous mitochondria. - In several species, plastids and mitochondria present in generative cells become modified or they degenerate during later stages. - Mitochondria are lost from sperms since cytoplasm is reduced during sperm maturation. - During fertilization, ordinarily, only the sperm nucleus enters egg cell, and its cytoplasm is left outside the egg cell. As a result, the mitochondria located in these organelles will also be derived from the female parent only. - There is strong microscopic, enzymatic and molecular marker-based evidence that plastid and mitochondrial DNA modification/degradation occurs during pollen maturation in those species that show maternal transmission of these organelles. - But it may be emphasized that these mechanisms, acting either alone or in combination, are not 100% effective all the time. - For example, recent studies reveal that transgenes integrated into chloroplast genome show a low (up to 0.5%) level of pollen transmission in tobacco and some other plant species. ## 24.5.2. Pelargonium zonale - Biparental transmission of plastids was demonstrated in *Pealargonium zonale* (horseshoe geranium) by Baur in 1908 (Table 24.2). - In this case, green, white and variegated progeny were derived from a vast majority of crosses, and the results from reciprocal crosses were almost similar, at least in some cases, but there was a lack of a definite ratio among the three types of progeny. - Further, there was a marked preferential transmission of normal plastids even when the green varieties were used as the male parent. ## 24.5.3. Chlamydomonas reinhardtii - Plasmagenes of *Chlamydomonas reinhardtii* have been extensively investigated by Sager and coworkers. - This green alga is haploid (n), and it has two mating types designated as + and -. - The two mating types are governed by two alleles of a single gene, the alleles being denoted as mt* and mt. - The + mating type is regarded as female, while the - mating type is considered as male. - During conjugation, one mt and one mt cell pair and fuse together, bringing together in the same zygote the cytoplasms from both mt⁺ and mt cells. - But the plasmagenes from the mt parent are inactivated in the zygote leaving only those from the mt* parent in an active state. - The mechanism of inactivation of mt plasmagenes seems to be due to the destruction of mt cpDNA. - The zygote undergoes meiosis to produce 4 or 8 haploid zoospores. - The zoospores show typical 1: 1 segregation for nuclear genes, e.g., mt/mt. - But all the zoospores are identical with each other and with the mt¹ parent for their plasmagenes. - A form of streptomycin resistance is located in cpDNA of *Chlamydomonas*. - Matings between mt streptomycin resistant and mt susceptible cells produce only resistant progeny. - But the reciprocal matings yield allsusceptible progeny (Fig. 24.1). - In both the types of matings, progeny show normal segregation for the nuclear gene mt and mt (ratio 1:1). - In less than 1% (Ca. 0.1%) of zygotes, plasmagenes from the mt parent are not inazizald; as result cytohets, i.e., heterozygotes for cytoplasmic genes, are obtained. ## 24.6. Chloroplast DNA (cpDNA) - Chloroplasts contain naked circular DNA molecules of about 120 kb to 217 kb (in geranium). - But is most angiosperms, the genome size varies within a relatively narrow range of 120 of 160 kb. - A single such molecule constitutes the chloroplast genome in most species. - But in some brown algae, a chloroplast genome may consist of more than one circular DNA molecule. - Each chloroplast has 10-60, in some plants up to 100, copies of its genome; these are arranged in 5-6 specific regions within a chloroplast. - In most plant species, cpDNA contains inverted repeats, but in *Euglena* and *Acetabularia* tandem repeats have been reported. - In higher plants, cpDNA exhibits a characteristic feature; it has a 10 to 24 kb long sequence (the length depends on plant species) that is present in two identical copies per genome as an inverted repeat (Fig. 24.3). - The repeats contain genes, including tRNA genes, and separate the genome into a short and a large single copy sequence (SSC and LSC). - Recombination between the repeats can generate inversion for the SSC with respect to the LSC. - The complete sequence of cpDNA has been determined for > 100 plant species. - Most of the gene products are either components of thylakoid membranes or concerned with redox reactions (Table 24.3). ## 24.7. Mitochondrial Inheritance - Many plasmagenes are believed to be located in mitochondrial DNA (mtDNA). - Generally, mitochondria from only one of the parents are transmitted to the progeny, and genes located in mtDNA show cytoplasmic inheritance. - Several plasmagenes, e.g., some kinds of petites, are located in mtDNA of yeast. - Cytohet recombination mapping of plasmagenes located in mtDNA. - Streptomycin resistance in *Paramecium* is determined by a mitochondrial gene; this has been demonstrated by nuclear transplantation. - In many crop plants, cytoplasmic male sterility is known to occur sporadically in experimental populations; it has also been produced by constructing appropriate alloplasmic lines, e.g., in wheat, tobacco, chillies, etc. - Cytoplasmic male sterility is controlled by mtDNA. ### 24.7.1. Cytoplasmic Male Sterility - Cytoplasmic male sterility (CMS) shows typical cytoplasmic inheritance. - Progeny from CMS and a normal male fertile strain are all male sterile. - As a result, a CMS strain has to be pollinated by a male fertile strain in every generation for its maintenance (Fig. 24.4). - CMS is extensively used in hybrid seed production in crops maize, jowar, bajra, etc. - CMS in maize has been the most extensively investigated. - Three distinct CMS cytoplasms, designated as CMS-T, CMS-C and CMS-S, are known in maize. - Some dominant genes, called restorer genes, nullify the effects of CMS on male fertility so that individuals having a restorer gene in homozygous or heterozygous state are male fertile even in the presence of the CMS cytoplasm (Fig. 24.5). - Most restorer genes are sporophytic in action, i.e., survival and function of pollen depends on the genotype of the plant producing the pollen and not a on the genotype of the pollen itself. - But *Rf*, in maize has a gametic action so that *Rf Rf* heterozygotes produce 50% viable (*Rf*) and 50% dead (*rfs*) pollen grains. - The mechanisms of male sterility and fertility restoration are not clear. - In CMS lines, some unique polypeptides are produed in mitochondria, while some polypeptides ordinarily produced by the normal cytoplasm may be absent (Table 24.4). - Restorer genes prevent the production of additional polypeptide in CMS-T, but they have no apparent effect in CMS-C and CMS-S. - CMS-C and CMS-S have two additional plasmid-like elements not found in the mitochondria of normal cytoplasms; these elements are designated as S₁ and S₂ in the case of CMS-S. - CMS-S shows a relatively high frequency of reversion to male fertility, the reversions being produced by both nuclear and plasmagenes. - In the cytoplasmic reverants of CMS-S, S₁ and S₂ elements are absent from the mitochondria. - In contrast, CMS-T lacks a plasmid-like element ordinarily found in the mitochondria of normal cytoplasms. ### 24.7.2. Mitochondria in Human Disease - Several human diseases are caused by mitochondrial defects, and some of these, e.g. Leber's hereditary optic neuropathy (LHON), have been shown to be due to mutations in mtDNA. - LHON is characterised by the sudden onset of blindness in adults, which is associated with the death of optic nerve due to disruptions in aerobic respiration/oxidative phosphorylation to such an extent that function of optic nerve is destroyed resulting in total blindness. - It is not known why this lethal effect is limited only to the optic nerve; one possibility is that nerve cells are particularly sensitive to disruptions in aerobic respiration/oxidative phosphorylation. - LHON shows strict maternal transmission, and no case of paternal transmission is known. ## 24.8. Mitochondrial DNA (mtDNA) - Generally, the mitochondrial genome comprises a single circular DNA molecule, but in some species multiple circular molecules are found. - In some protists, the genome comprises multiple linear molecules with terminal repeat motifs. - The genome size varies greatly from merely 6 kb in *Plasmodium* to 2 Mb in cucurbits, but it typically ranges from 15 to 60 kb. - Clearly, the mitochondrial genome (Table 24.5) shows a much larger variation in size than that found in the chloroplast genome. - The G + C content of mtDNA shows considerable variation from one species to another, e.g., 18% in yeast and 47% in higher plants. - The mitochondrial genome has only 5 genes in *Plasmodium*, while in some flagellates it has 100 genes, the common number being 40-50 across various eukaryotes. - mtDNA codes for 2 types of rRNAs, 22 (mammals) to 26 (yeast) different tRNAs and 8 different protein molecules. - In yeast, ~4 copies of mitochondrial genome are present per mitochondrion. - In higher plants, mtDNA has short homologous sequences, recombination within which generates smaller, subgenomic circles that are present with the full genome, i.e., the ’master’ genome. - mtDNAs contain relatively small (mammals) to large inverted repeat sequences (higher plants). - The mtDNA encodes all the RNA species and some of the proteins needed for mitochondrial function, the genes being transcribed and translated within mitochondria (Table 24.6). - The encoded proteins are mainly components of the multimeric complexes I-IV. - Plant mitochondria also encode many ribosomal proteins. - In protists, proteins involved in cytoplasm to mitochondria protein trafficking are also encoded by mtDNA. ### 24.8.1. Mammalian Mitochondrial Genome - The mitochondrial genome has been completely sequenced in several mammalian species. - The genome organization is extremely compact: - There are no introns. - Some genes actually overlap. - Only 87 of the 16,569 bp of human mtDNA can be considered to lie in intercistronic region. - In human mtDNA, there are 13 protein coding regions, including cytochrome b, 3 subunits of cytochrome oxidase, 1 subunit of ATPase, and 7 subunits of NADH dehydrogenase. - In addition, 2 rRNA (12S to 16S) genes are located side by side next to the D loop. - Genes for tRNA are located between genes encoding proteins or rRNA; in only one case, two genes transcribed in clockwise direction (ATPase 6 and CO3), are not separated by tRNA genes (Fig. 24.6). - The main features of gene organization are as follows: - In many cases, the last base of one gene lies next to the first base of the next gene. - In many cases, genes overlap, overlap of one base being the most common. - There is on termination codon in 5 of the reading frames. - These frames and with organization is found in other mammals and in *X. laevis*. - In three cases, the termination codon appears to be AGA or AGG. - The initiation codon (AUG, AUA or AUU) lies within 6 bases of the start point. - Mitochondrial mRNAs virtually do not have nontranslated 5' and 3' sequences. - Of the 15 genes that encode protein or rRNA, 14 are transcribed in clockwise direction, while only one (ND6) is transcribed in anticlockwise direction. - The promoters for clockwise and anticlockwise transcriptions are located within the D loop. - Therefore, transcription begins in the D loop and is terminated within the D loop after transcription of the complete circular genome. - Mitochondrial DNA can be separated into a heavy strand (H strand) and a light strand (L strand) due to differences in their density. - In clockwise transcription, the H strand is transcribed, while the L strand is transcribed in anticlockwise direction. - Both clockwise and anticlockwise transcriptions generate a single transcript representing the entire mtDNA, except some regions, designated as D loop. - The clockwise transcript is cleaved on either side of the D loop. - The L strand is also transcribed in greater amounts than mRNA, presumably by premature termination of the giant RNA transcript, which is cleaved to generate a number of monocistronic mRNAS, tRNAS and 8 tRNAS, the rest of the transcript being, presumably, degraded. ## 24.9. Features of Organellar Genomes - They are circular molecules of DNA. - In few exceptional cases, generally in lower eukaryotes, mtDNA is linear. - They are present in multiple copies in each organelle. - In higher plants, cpDNA is present in 20-40 copies per chloroplast. - Yeast cells have ~4 genomes per mitochondrion. - They encode all the RNA species and some of the proteins required for organelle function. - They are transcribed and translated within the organelles. - In cases of biparental inheritance, recombination does take place between organellar genomes. - Organelle DNA is replicated by a different DNA polymerase from that of the nucleus. - The DNA repair systems and other events that impinge on the fidelity of DNA sequences are different in organelles from those in nuclei. - Accumulation of mutation is much faster in mt DNA that in nuclear genome in the case of organelles, while in plants the reverse is the case. - The cpDNA is intermediate between mtDNA and nuclear DNA. - Mammalian MtDNA accumulates mutations at 2-4% per million years, which is more that 10-times faster than the rate for globin genes. - In case of uniparental inheritance in *Chlamydomonas*, genes from one of the parents is degraded shortly after zygote formation, leaving in the zygote cpDNA from one of the parents. - Chloroplast genome is much larger (~140 kb in higher plants and <200 kb in yeast). - In plants, mtDNA has short homologous sequences. - Recombination within these sequences generates smaller, subgenomic circles that coexist with the complete genome. ## 24.10. Recombination in Organellar Genomes - Cytoplasmic heterozygotes are produced in *Chlamydomonas* and yeast; they have been used to investigate complementation, recombination and genomic rearrangements, e.g., deletions, in organellar genomes. - Complementation requires the mixing up of the gene products of two genotypically different mitochondria or chloroplasts contributed by the two parents involved in mating. - Complementation has been demonstrated between mitochondrial gene mutations in yeast; it has been used to classify different mitochondrial gene mutations into complementation groups. - It has been exploited to prepare linkage maps of the mitochondrial genomes in yeast. - In both these organisms, it has been experimentally demonstrated that recombination occurs between chloroplast genomes. - The occurrence of recombination requires the genomes of two totally different organelles to be brought in physical proximity. - This is presumably achieved by a fusion two or more of the organelles together. - Mitochondrial genome is surprisingly fluid, and different strains may differ by up to 10,000 bp in their genome size. - Extensive deletions can occur in mtDNA of *S. cerevisiae* in case of petite mutant. - All petite mutants lack mitochondrial function; they survive in yeast since it can grow anaerobically. - The petite mutations of *S. cerevisiae* are of 3 types: (1) nuclear, (2) neutral, and (3) suppressive petites. - Nuclear petites are produced by nuclear gene mutations that abolish mitochondrial function, and show Mendelian inheritance. - Neutral (rhoº) petites are characterised by the absence of mtDNA; they represent an extreme situation and are recessive in inheritance. - But suppressive (rho¯) petites have much smaller mtDNAs, the complexity of their genomes ranging from only 0.2% to 36% of the normal genome. - These petites may contain any sequence of mtDNA; this sequence is often amplified to generate a large number of copies, which may be present as separate circles, or as a multimeric molecule. - Some petites are stable, while others are unstable, and further rearrangement of their sequences occur. - When a rho¯ petite is crossed with a wild type strain, only the petite genotype is found in certain proportion of the progeny, and the wild type DNA disappears from them. - This effect is called suppression, and the proportion of progeny in which suppression occurs constitutes the degree of suppressiveness. - The cause of suppression in highly suppressive petites is a preferential replication of the petite genome possibly due to the presence of a greater number of origin like sequences, called rep regions, in the petite mtDNA than in the wild type mtDNA. ## 24.11. Promiscuous DNA - Since early 1980’s evidence has accumulated to show that DNA has migrated from chloroplasts to mitochondria, and from both mitochondria and chloroplasts to eukaryotic nucleus. - The DNA segments that have migrated from one organelle into the other are termed as promiscuous DNA. - It is estimated that *Arabidopsis thaliana* nuclear genome has received between 400 and 2,200 genes of its projected 25,000 nuclear genes from the endosymbionts that later became chloroplasts and mitochondria (Section 24.14). - There is evidence that genes have moved from mitochondria to plastids and vice-versa, but this movement seems to be much smaller than that to the nuclear genome. - There is considerable variation in the number of genes present in the organelles of different species. - In addition, there is variation in the gene sequences of organellar genomes from different species. ## 24.12 Transmission Patterns of Plasmagenes - The cytoplasmic organelles (plastids and mitochondria) show non Mendelian inheritance in all eukaryotes. - In case of plants, plastids generally show maternal transmission (Section 24.5), but about one-third of the genera of Angiosperms studied so far show biparental transmission. - While conifers (Gymnosperms), as a rule, exhibit paternal transmission, biparental plastid transmission is known in some families of conifers, e.g. *Araucariaceae*, *Cephalotaxaceae*, etc., show predominantly paternal transmission. - Previously, in some species like *Medicago*, the sperms receive ample number of plastids that are delivered into egg cell during fertilization. - The subsequent fate of paternal plastids depends on the species involved, and even on the genotypes, of given species, that are controlled by both nuclear and plastid genes in the concerned organelle. - It is not well known, but they are controlled by both nuclear and plastid genes. - The frequency of progeny having mixed plastids ranges from the least frequent to intermediate in some species like *Pelargonium*, *Oenothera*, etc. - While others contain plastids from either maternal or paternal parent only. - In the case of *Pelargonium*, the frequency of progeny containing mixed plastids, is more higher in those having maternal or paternal parent only, - It appears that: - Differential multiplication of the maternal and paternal plastids in the developing embryo affects the plastids transmission patterns. - Other factors like (2) sorting out of plastids during subsequent cell divisions, (3) differential cpDNA degradation in the zygote, and (4) degradation of maternal plastids may also affect the biparental plastid inheritance patterns. - Paternal inheritance of plastids in predominant in conifers. - In contrast, paternal transmission of mitochondria is rare, and even those conifers that show paternal plastid inheritance show maternal transmission of mitochondria. - In case of Douglas-fir, the male cytoplasm is included in the egg, and this cytoplasm is rich in plastids and mitochondria. - After the first zygotic division, the two free pro-embryo nuclei are surrounded by primarily maternal mitochondria and paternal plastids; the maternal plastids are modified before fertilization and are not closely associated with the egg nucleus. Thus, the embryonic cytoplasm is derived from a combination of cytoplasm from both the parents, but it includes almost exclusively male plastids and female mitochondria. - This mechanism does lead to some ‘leakiness’ as maternal plastids may be present in some progenies. - In species like *Biota orientalis*, both the plastids and mitochondria show paternal transmission. - In *B. orientalis*, the sperm nucleus remains tightly enclosed by its cytoplasm, which contains both plastids and mitochondria even after it enters the egg. - As a result, the zygote and the pro-embryo cells are surrounded by only the male cytoplasm, and the female cytoplasm degenerates. - This mechanism also may show some ‘leakiness’. - Uniparental inheritance of plastids and mitochondria is the general rule for most eukaryotes, including animals; further, maternal transmission is by far the most predominant. - It has been suggested that biparental transmission of plastids is most probably the primitive condition. - The uniparental inheritance is proposed to be advantageous as it would guard against incompatibility between organellar genomes and between organellar and nuclear genomes. - Similarly, maternal transmission may have evolved as a mechanism that prevents foreign or pathogenic DNA from entering the egg, and paternal organelles just happen to be grouped into this category by the mechanisms involved. - It has been advocated that reduction in sperm cytoplasm may have evolved as an adaptation that facilitates sperm movement and/or translocation within pollen tube, and it may be only indirectly related to maternal transmission of cytoplasmic organelles. ## 24.13. Nuclear-Cytoplasmic Interaction - There is ample evidence that nuclear genes influence the expression of plasmagenes. - A classical example of interaction between nuclear and cytoplasmic genomes is that between CMS and restorer genes in *maize*. - An unusual case of interaction between nuclear and cytoplasmtc genomes is reported in *maize*. - A type of variegation, called *iojap*, is produced by a recessive nuclear gene *ij*. - Plants homozygous for this gene develop the typical *iojap* variegation, i.e. white longitudinal stripes of variable width. - But once the variegation is produced by the nuclear gene *ij*, it shows a typical cytoplasmic inheritance (Fig. 24.8). - Once this mutation is induced in some cpDNA molecules, the variegation is inherited cytoplasmically. - The cross between normal (I I) plants as female and *iojap* (ij ij) plants as male produces all green plants in F₁ with the nuclear genotype Iij. - In the F₂ generation of this cross, 1/4 progeny are *ij ij* and develop the *iojap* variegation; the remaining 3/4 of the progeny are normal green (Fig. 24.7). - But when *iojap* plants are mated as females with normal green plants used as males, green, white and *iojap* progeny are recovered and the ratio between the three types of progeny, however, is quite variable. ## 24.14. Evolutionary Significance of the Organelles - Ever since organellar genomes were discovered, biologists have debated their implications for theories about the evolution of eukaryotic cells. - It has been proposed that mitochondria and chloroplasts were once free living prokaryotes that formed symbiotic relationships with the primitive eukaryotic cells over one billion years ago. - Some of the evidence that supports this hypothesis is as follows: - Mitochondria and chloroplasts are of about the same sizes as bacteria. - These organelles contain circular genomes like bacteria. - Their ribosomes are more similar to those of bacteria than to those of eukaryotes. - Their RNA polymerases are distinct from those of eukaryotes and closer to those of prokaryotes; chloroplast RNA polymerase is similar to that of *E. coli*. - Sequence of some genes of mitochondria and chloroplasts are similar to those of bacterial genes. - For example, mitochondrial genes for rRNA are most closely related to rRNA genes of a group of purple photosynthetic bacteria. - The present-day chloroplast and mitochondrial genomes seem to have been derived from several different symbiotic partnerships that were established independently very long ago. - The genomes of the endosymbionts have been modified by (i) loss of the genes required for independent survival and propagation, and (ii) transfer of DNA to the host nuclear genome that would provide some of the proteins needed for their function. - It is suggested that DNA transfers to host nuclei most likely occurred before the cellular compartments were fully established. - It seems that gene transfers to nucleus from mitochondria in case of animal cells occurred only once. - But in case of plants, the gene transfers may be occurring even today. - The rate of gene transfers from mitochondria to nuclei occurs at the rate of 2 × 10⁻⁵ per, generation, while that from chloroplasts is much higher (6 × 10⁻⁵ per generation). - The rate of gene transfers from nuclei to mitochondria were found to be lower at about 5 orders of magnitude (< 1 × 10⁻¹⁰ per generation). - The chloroplast most likely evolved from cyanobacteria. - The chloroplast rRNA genes are most closely related to those of cyanobacterium. - Further, a protozoan (Cynaphora paradox`) is able to photosynthesize because it contains structures that look like of cyanobacteria, and fragments of bacterial cell wall are associated with it. - Similarly sequence homologies suggest that mitochondria are phylogenetically related to a-purple bacteria, and the obligate parasite Rickettsia is the most closly related. - Most likely, mitochondria and Rickettsia both have evolved from a common ancestor. ## 24.15. Maternal Effects - The development of some characters in many organisms is either governed or markedly influenced by the genotype of the female parent; this is known as maternal effect. - Although, such characters are governed in most cases, disappear in F₂, and (3) a considerably smaller variation in F₂ as compared to that in F₂. - In some extreme cases, there may be no phenotypic segregation in F₂, but it does become evident only in F₃. - Many important characteristics of both plants and animals show maternal effects, of which some examples are described below. ## 24.16. Inheritance Due To Parasites, Symbionts and Viruses - Some of the once classical cases of cytoplasmic inheritance are actually due to the presence of parasites, symbionts or viruses. - Clearly, such particles are not an integral part of the normal organization of the concerned organelle, and cannot be regarded as they are dispensable. - Consequently, the inheritance patterns arising from such agents cannot be regarded as genuine cases of cytoplasmic inheritance. - One of the earliest examples of this type of inheritance was provided by the killer trait of *Paramecium*. - It is produced by Kappa particles; these particles are symbiotic bacteria called Caedibacter taeniospiralis. - These endosymbionts are known in different species of *P. aurelia* group, in other species of *Paramecium*, and in other protozoa, many insects, etc. - Some