Cumulative Immunology Fall 2024 PDF
Document Details
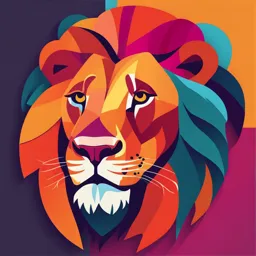
Uploaded by BlitheGallium
Midwestern University
2024
Tags
Summary
This document is a set of notes on cumulative immunology for the fall 2024 semester. It covers topics such as comparing and contrasting the innate and adaptive immune systems, the function of various types of white blood cells, and the roles of primary and secondary lymphatic tissues. It also discusses different types of antigens, epitopes, and haptens.
Full Transcript
Cumulative Immunology Section 1-4 Compare and contrast the characteristics of the innate and adaptive immune system The immune system has two main branches, the **innate** and **adaptive immune systems**, each with distinct characteristics and roles in defending the body against pathogens. **1....
Cumulative Immunology Section 1-4 Compare and contrast the characteristics of the innate and adaptive immune system The immune system has two main branches, the **innate** and **adaptive immune systems**, each with distinct characteristics and roles in defending the body against pathogens. **1. Timing and Response Speed** - **Innate Immune System**: Acts quickly, providing an immediate response within minutes to hours of pathogen exposure. - **Adaptive Immune System**: Takes longer to activate, requiring days to weeks to mount a full response upon initial exposure to a pathogen. However, it responds faster upon re-exposure due to memory cells. **2. Specificity** - **Innate Immune System**: Non-specific; it recognizes broad categories of pathogens (e.g., bacteria, viruses) using generic pathogen-associated molecular patterns (PAMPs). - **Adaptive Immune System**: Highly specific; it recognizes unique antigens on pathogens using specific receptors on B and T cells. **3. Memory** - **Innate Immune System**: Lacks memory; each encounter with a pathogen triggers the same response. - **Adaptive Immune System**: Has immunological memory; upon re-exposure to the same pathogen, memory B and T cells facilitate a faster, stronger response. **4. Key Components** - **Innate Immune System**: - Physical barriers: skin, mucous membranes. - Cells: macrophages, neutrophils, dendritic cells, natural killer (NK) cells. - Soluble factors: cytokines, complement proteins. - **Adaptive Immune System**: - Cells: B cells (produce antibodies) and T cells (helper and cytotoxic T cells). - Antibodies: Immunoglobulins specifically targeting pathogens. **5. Diversity of Response** - **Innate Immune System**: Limited diversity; uses a fixed set of receptors to recognize PAMPs. - **Adaptive Immune System**: Highly diverse; B and T cells undergo genetic recombination to create a vast array of receptors for specific antigens. **6. Role in Immune Response** - **Innate Immune System**: Serves as the first line of defense, managing infections in the early stages, and activating the adaptive immune system. - **Adaptive Immune System**: Provides a long-lasting, targeted response and clears infections that the innate immune response cannot fully resolve. **Summary Table** **Feature** **Innate Immune System** **Adaptive Immune System** ---------------------- ---------------------------------------------------- ---------------------------------------- **Response Speed** Immediate Delayed (days to weeks) **Specificity** Broad, non-specific Highly specific **Memory** No Yes (immunological memory) **Components** Physical barriers, phagocytes, NK cells, cytokines B cells, T cells, antibodies **Diversity** Limited High (receptor diversity) **Primary Function** Initial, rapid response Long-term, targeted pathogen clearance Both systems work together: the innate immune system quickly identifies and contains threats, while the adaptive immune system provides a tailored, long-term defense. Identify the WBCs that express the following CD markers: CD3, CD4, CD8, CD14, CD19, CD20, and CD56 Here\'s a list of the white blood cells (WBCs) that express each of the specified CD markers: 1. **CD3**: Expressed by **T cells** (both helper and cytotoxic T cells). CD3 is a coreceptor involved in T cell activation. 2. **CD4**: Expressed by **Helper T cells** (a subset of T cells). CD4 assists in antigen recognition by binding to MHC class II molecules on antigen-presenting cells. 3. **CD8**: Expressed by **Cytotoxic T cells** (another subset of T cells). CD8 binds to MHC class I molecules and is essential for the function of cytotoxic T cells in targeting infected or abnormal cells. 4. **CD14**: Expressed primarily by **Monocytes** and **Macrophages**. CD14 is a receptor for bacterial lipopolysaccharide (LPS) and plays a role in innate immune responses to pathogens. 5. **CD19**: Expressed by **B cells**. CD19 is part of the B cell receptor complex and is essential for B cell activation and antibody production. 6. **CD20**: Also expressed by **B cells**, particularly during their development and maturation stages. CD20 is involved in B cell activation and calcium signaling. 7. **CD56**: Expressed by **Natural Killer (NK) cells** and a subset of **T cells** (specifically, NKT cells). CD56 is involved in NK cell adhesion and is a marker of NK cell activation. These CD markers help in identifying and differentiating various immune cell types, playing crucial roles in diagnosing and researching immune responses and disorders. Understand the function(s) of each WBC discussed Each type of white blood cell (WBC) discussed plays a distinct role in the immune system, contributing to either the innate or adaptive immune response: 1. **T Cells (express CD3)**: - **Function**: T cells are central to the adaptive immune system. CD3 is a part of the T cell receptor complex that transmits signals to activate T cells upon antigen recognition. - T cells can be subdivided into different types, with unique roles: - **Helper T Cells (CD4+ T cells)**: - **Function**: They coordinate the immune response by releasing cytokines, which activate other immune cells such as B cells, cytotoxic T cells, and macrophages. - **Role**: Helper T cells help in recognizing antigens presented by MHC class II molecules on antigen-presenting cells. - **Cytotoxic T Cells (CD8+ T cells)**: - **Function**: These cells are responsible for directly killing infected, cancerous, or otherwise abnormal cells. - **Role**: They recognize antigens presented by MHC class I molecules and release toxic substances that induce apoptosis in the target cells. 2. **Monocytes and Macrophages (express CD14)**: - **Function**: Monocytes are precursors to macrophages and dendritic cells and play a critical role in innate immunity. - **Role**: Monocytes circulate in the blood and migrate into tissues, where they differentiate into macrophages. Macrophages are phagocytic cells that engulf pathogens, dead cells, and debris. They also produce inflammatory cytokines and present antigens to T cells, helping to bridge innate and adaptive immunity. 3. **B Cells (express CD19 and CD20)**: - **Function**: B cells are essential for humoral immunity within the adaptive immune system. - **Role**: B cells produce antibodies that specifically target antigens on pathogens. CD19 and CD20 are markers involved in B cell activation and development. Upon encountering their specific antigen, B cells can differentiate into plasma cells (which produce antibodies) or memory B cells (which provide long-term immunity). 4. **Natural Killer (NK) Cells (express CD56)**: - **Function**: NK cells are part of the innate immune system, acting as a first line of defense. - **Role**: NK cells identify and kill virus-infected cells or tumor cells without needing prior sensitization. They can recognize cells with decreased MHC class I expression, a common characteristic of infected or cancerous cells. NK cells also release cytokines like interferon-gamma (IFN-γ), enhancing immune responses. Each WBC has a unique role but works in concert with other cells to maintain immune homeostasis and protect the body against pathogens and diseased cells. Know the function of primary and secondary lymphatic tissues The lymphatic system includes **primary and secondary lymphatic tissues**, each with specific roles in immune function: **1. Primary Lymphatic Tissues** - **Function**: Primary lymphatic tissues are responsible for the **production, maturation, and differentiation** of lymphocytes (B and T cells). - **Main Sites**: - **Bone Marrow**: - **Function**: Produces all blood cells, including lymphocytes. B cells mature in the bone marrow, where they undergo development and selection to ensure they can recognize pathogens without attacking the body's own cells. - **Thymus**: - **Function**: The thymus is the site where T cells mature. Immature T cells (thymocytes) migrate to the thymus from the bone marrow and undergo processes of positive and negative selection to ensure they can respond appropriately to foreign antigens while remaining tolerant to self-antigens. - **Role**: Primary lymphatic tissues ensure that lymphocytes are properly developed and educated before they encounter pathogens in secondary lymphatic tissues. **2. Secondary Lymphatic Tissues** - **Function**: Secondary lymphatic tissues provide an environment for **immune cell activation, proliferation, and response** to pathogens. These tissues are where lymphocytes first encounter antigens and become activated. - **Main Sites**: - **Lymph Nodes**: - **Function**: Lymph nodes filter lymphatic fluid and are packed with immune cells, including T cells, B cells, macrophages, and dendritic cells. They capture antigens from the lymph and present them to lymphocytes, facilitating an immune response. - **Spleen**: - **Function**: The spleen filters blood, removing old or damaged red blood cells and detecting blood-borne pathogens. The white pulp of the spleen contains lymphocytes and functions similarly to a lymph node but filters blood rather than lymph. - **Mucosa-Associated Lymphoid Tissues (MALT)** (including tonsils, Peyer's patches in the intestines, and others): - **Function**: MALT sites are strategically located along mucosal surfaces (respiratory, gastrointestinal, and genitourinary tracts) to detect pathogens that enter the body through these routes. They contain lymphocytes and antigen-presenting cells to initiate localized immune responses. - **Role**: Secondary lymphatic tissues facilitate the immune system's ability to detect and respond to pathogens throughout the body, localizing immune responses to sites of infection or injury. In summary: - **Primary lymphatic tissues** are responsible for lymphocyte production and maturation. - **Secondary lymphatic tissues** serve as sites where lymphocytes encounter antigens, become activated, and carry out immune responses. Know and describe antigen (monovalent, multivalent, and polyvalent), epitope, and hapten Understanding antigens, epitopes, and haptens is key to grasping immune system functioning and immune response specifics: **1. Antigen** - **Definition**: An antigen is any substance that can be recognized by the immune system and elicits an immune response, typically by binding to receptors on B cells or T cells. - **Types**: - **Monovalent Antigen**: Has only **one epitope**, meaning it can bind to only one specific antibody or receptor at a time. Monovalent antigens usually trigger a limited immune response due to the single binding site. - **Multivalent Antigen**: Contains **multiple copies of the same epitope**, allowing it to bind to multiple antibodies or receptors of the same specificity. This multivalent nature can enhance immune recognition and response. - **Polyvalent Antigen**: Has **multiple, different epitopes** that can bind to a variety of antibodies or receptors. This diversity enables a stronger and broader immune response since multiple immune cell types can recognize the antigen. **2. Epitope** - **Definition**: An epitope, also known as an **antigenic determinant**, is the specific part of an antigen that is recognized and bound by an antibody, B cell receptor, or T cell receptor. - **Types**: - **Linear Epitope**: Formed by a sequence of amino acids in a row within the protein structure. It is recognized by immune cells based on its primary structure. - **Conformational Epitope**: Created by the three-dimensional folding of a protein, bringing distant amino acids into proximity. Recognition depends on the specific 3D structure and may be disrupted if the protein is denatured. - **Function**: Epitopes are the actual targets of immune recognition. An antigen can have multiple epitopes, allowing multiple immune cells to respond to a single antigen. **3. Hapten** - **Definition**: A hapten is a **small molecule** that, by itself, is not immunogenic (does not elicit an immune response). However, when it binds to a larger carrier protein, it forms a complex that can be recognized by the immune system and elicit a response. - **Example**: Certain drugs, toxins, or chemicals can act as haptens. For instance, penicillin can act as a hapten, binding to proteins in the body and potentially causing an allergic reaction. - **Role in Immunity**: Haptens can be used in vaccine design or allergy research. By studying how haptens induce immune responses when attached to carriers, researchers gain insights into immune system sensitization and responses to small molecules. **Summary:** - **Antigens** are any substances that stimulate an immune response and can be monovalent, multivalent, or polyvalent. - **Epitopes** are the specific regions on antigens recognized by immune receptors. - **Haptens** are small molecules that only become immunogenic when bound to larger proteins, acting as a partial antigen. This understanding is critical in immunology, vaccine development, and allergy research. Understand what pattern recognition receptors (PRRs) are, which cells express PRRs, and how PRRs participate in immune system function **Pattern Recognition Receptors (PRRs)** are specialized receptors on immune cells that recognize **Pathogen-Associated Molecular Patterns (PAMPs)**, which are molecular signatures commonly found on pathogens, such as bacteria, viruses, and fungi. PRRs are crucial to the **innate immune system** because they provide the first line of defense by rapidly detecting and responding to infections in a nonspecific manner. **Key Aspects of Pattern Recognition Receptors (PRRs):** 1. **Types of PRRs**: - **Toll-Like Receptors (TLRs)**: These are membrane-bound receptors found on cell surfaces and within endosomes. Different TLRs recognize various PAMPs, such as bacterial lipopolysaccharides (TLR4), viral RNA (TLR3), and bacterial DNA (TLR9). - **NOD-Like Receptors (NLRs)**: Located within the cytoplasm, NLRs detect bacterial components and cellular stress signals, often triggering inflammatory responses. - **RIG-I-Like Receptors (RLRs)**: These cytoplasmic receptors detect viral RNA within cells, signaling the presence of viral infections and stimulating an antiviral response. - **C-Type Lectin Receptors (CLRs)**: These receptors recognize carbohydrate structures on the surfaces of fungi, bacteria, and some viruses, playing a role in recognizing extracellular pathogens. - **DNA Sensors**: Detect DNA within the cytoplasm, which usually indicates the presence of viral or bacterial infections (e.g., cGAS-STING pathway). 2. **Cells That Express PRRs**: - PRRs are mainly expressed on **innate immune cells**: - **Macrophages** and **Dendritic Cells**: As professional antigen-presenting cells, these cells use PRRs to detect pathogens and initiate immune responses. - **Neutrophils**: Express PRRs to detect and destroy pathogens through phagocytosis and the release of antimicrobial substances. - **Natural Killer (NK) Cells**: While primarily recognizing infected or abnormal host cells, NK cells also have PRRs to detect stress or infection markers. - **Non-immune Cells**: Some non-immune cells, such as **epithelial cells** in the respiratory and gastrointestinal tracts, also express PRRs to detect and respond to pathogens at barrier sites. 3. **Role of PRRs in Immune Function**: - **Pathogen Detection**: PRRs recognize PAMPs that are unique to pathogens but absent in host cells, allowing the immune system to distinguish between self and non-self. - **Signal Transduction**: Upon recognizing a PAMP, PRRs activate signaling pathways within immune cells, leading to the production of inflammatory cytokines, chemokines, and interferons. These molecules coordinate immune responses by attracting additional immune cells to the site of infection and activating adaptive immunity. - **Antigen Presentation**: PRR activation enhances the ability of dendritic cells and macrophages to process and present antigens to T cells, bridging innate and adaptive immunity. - **Phagocytosis and Killing**: Recognition of pathogens by PRRs triggers phagocytosis, where cells like macrophages and neutrophils engulf and destroy pathogens. - **Inflammatory Response**: Some PRRs, especially NLRs, can form complexes called inflammasomes, which activate inflammatory responses to help clear infections but may also contribute to inflammatory diseases if dysregulated. **Summary** PRRs are essential for initiating the immune response against infections. They enable innate immune cells to detect and respond quickly to pathogens, help bridge innate and adaptive immunity, and ensure that immune responses are targeted at pathogens while sparing host cells. Through PRR activation, the innate immune system can react promptly, helping to control infections early and signaling for further immune response activation. Understand antigen receptors expressed by B and T cells, and the type(s) of antigens each can bind (eg, protein, carbohydrate, etc) B cells and T cells, the main lymphocytes of the adaptive immune system, have specialized **antigen receptors** that allow them to recognize and respond to foreign antigens. While both types of cells have antigen receptors, they differ in structure, binding capabilities, and the types of antigens they can recognize. **1. B Cell Receptors (BCRs)** - **Structure**: B cell receptors are membrane-bound **immunoglobulins (Ig)** (antibodies) that are found on the surface of B cells. Each BCR is specific to a particular antigen. - **Types of Antigens Recognized**: - **Proteins**: BCRs can bind to protein antigens, which are often involved in generating strong immune responses. - **Carbohydrates**: BCRs can recognize carbohydrate antigens, such as bacterial polysaccharides. - **Lipids and Nucleic Acids**: In some cases, BCRs can recognize lipid and nucleic acid antigens, especially when these molecules are part of complex structures. - **Binding Characteristics**: - BCRs can bind to **whole antigens** directly in their native form. They do not require the antigen to be processed or presented by other cells. - The antigen-binding site on a BCR can recognize **conformational (3D) or linear epitopes**, depending on the antigen's structure. - **Soluble Antibody Production**: Once a B cell is activated, it can differentiate into a plasma cell, which secretes antibodies that have the same specificity as the original BCR. These soluble antibodies circulate in the blood and lymph, binding to pathogens and marking them for destruction. **2. T Cell Receptors (TCRs)** - **Structure**: T cell receptors are membrane-bound proteins with variable regions that determine antigen specificity. TCRs are composed of **α and β chains** in most T cells, forming a structure that allows binding to specific antigens. - **Types of Antigens Recognized**: - **Peptides (Proteins)**: TCRs primarily recognize short peptide fragments derived from protein antigens. - **Binding Characteristics**: - TCRs cannot bind to free or whole antigens. They require that antigens be **processed and presented** by **Major Histocompatibility Complex (MHC)** molecules on the surface of antigen-presenting cells (APCs). - **MHC Class I** molecules present peptides to **CD8+ cytotoxic T cells**, typically originating from intracellular pathogens like viruses. - **MHC Class II** molecules present peptides to **CD4+ helper T cells**, typically derived from extracellular pathogens like bacteria. - TCRs recognize **linear epitopes** within the peptide antigen. Since the antigen is processed and broken down into peptide fragments, conformational epitopes are not recognized by TCRs. **Comparison of BCRs and TCRs** **Feature** **B Cell Receptors (BCRs)** **T Cell Receptors (TCRs)** -------------------------------------- ------------------------------------------------------------ ------------------------------------------------------------------ **Structure** Membrane-bound immunoglobulins Heterodimer of α and β chains **Antigen Presentation Requirement** Can bind directly to antigens Requires antigen presentation by MHC **Antigen Types Recognized** Proteins, carbohydrates, lipids, nucleic acids Primarily peptides (protein antigens) **Binding Form** Binds to native, unprocessed antigens Binds to processed peptide antigens **Epitope Recognition** Can recognize linear and conformational epitopes Recognizes linear epitopes only **Role After Activation** Can differentiate into plasma cells and secrete antibodies Differentiate into helper or cytotoxic T cells based on function **Summary:** - **BCRs** on B cells bind directly to whole, native antigens and can recognize a variety of molecular structures, including proteins, carbohydrates, lipids, and nucleic acids. - **TCRs** on T cells recognize peptide fragments presented by MHC molecules, requiring antigen processing by antigen-presenting cells and binding only to linear peptide epitopes. These distinctions enable the immune system to recognize a broad range of pathogens and target them with appropriate responses. Understand the difference between acute and chronic inflammation, focusing on the predominant cell type(s) involved in each type of inflammation **Inflammation** is the immune system's response to injury, infection, or harmful stimuli, which can be classified as either **acute** or **chronic**. These two types of inflammation differ in duration, cellular composition, and the nature of the immune response. **1. Acute Inflammation** - **Definition**: Acute inflammation is a **rapid and short-term** response to injury or infection, typically lasting a few hours to a few days. - **Purpose**: It aims to quickly contain and eliminate pathogens or damaged cells and start the healing process. - **Predominant Cell Types**: - **Neutrophils**: These are the most abundant and primary cells in acute inflammation. Neutrophils are recruited rapidly to the site of injury or infection, where they engulf pathogens, release enzymes, and produce reactive oxygen species to neutralize threats. - **Other Cells**: While neutrophils are predominant, other cells, such as **monocytes** and **macrophages**, can arrive later to help clear dead cells and debris and to signal the resolution of inflammation. - **Characteristics of Acute Inflammation**: - Marked by the classic signs of inflammation: redness, heat, swelling, pain, and sometimes loss of function. - Resolves relatively quickly once the initial cause of inflammation is eliminated, allowing tissues to return to normal. **2. Chronic Inflammation** - **Definition**: Chronic inflammation is a **prolonged** inflammatory response that can last weeks, months, or even years. It often arises when the triggering factor is persistent, such as in autoimmune diseases, long-term infections, or chronic exposure to irritants. - **Purpose**: Unlike acute inflammation, which aims to resolve quickly, chronic inflammation may attempt to control or contain a problem that the body cannot completely eliminate, sometimes leading to tissue damage over time. - **Predominant Cell Types**: - **Macrophages**: Macrophages play a central role in chronic inflammation, producing cytokines and growth factors that sustain the inflammatory response and promote tissue repair. However, prolonged activation of macrophages can lead to tissue damage and fibrosis. - **Lymphocytes (T and B cells)**: These cells are more common in chronic inflammation than in acute inflammation. They are often involved in the adaptive immune response, especially in autoimmune or persistent infections where the immune system is continuously stimulated. - **Other Cells**: **Plasma cells** (activated B cells that produce antibodies) and **fibroblasts** (which contribute to tissue fibrosis) can also be involved. - **Characteristics of Chronic Inflammation**: - Chronic inflammation often lacks the classic signs of acute inflammation and may not be as immediately obvious. - It can lead to tissue damage, fibrosis, and scarring due to the prolonged presence of immune cells and cytokines. - Examples of conditions associated with chronic inflammation include rheumatoid arthritis, tuberculosis, and inflammatory bowel disease. **Summary Table** **Feature** **Acute Inflammation** **Chronic Inflammation** --------------------------- ------------------------------------- --------------------------------------------------------- **Duration** Short-term (hours to days) Long-term (weeks to years) **Primary Purpose** Rapid response to eliminate threats Persistent response to control ongoing issues **Predominant Cells** Neutrophils Macrophages, lymphocytes (T and B cells) **Resolution** Quick resolution and healing Ongoing response, often with tissue damage and fibrosis **Associated Conditions** Infections, injury, acute trauma Autoimmune diseases, persistent infections In summary, **acute inflammation** involves a rapid influx of neutrophils to quickly address damage or infection, while **chronic inflammation** involves macrophages and lymphocytes in a prolonged response that can cause tissue damage and fibrosis. These differences reflect the body's attempt to adapt to short-term versus long-term immune challenges. Describe the process of extravasation, including knowing the role of selectins and integrins. **Extravasation** is the process by which immune cells (primarily leukocytes, or white blood cells) move out of the bloodstream and into tissues, especially at sites of infection or inflammation. This process is essential for mounting an effective immune response and involves several key steps and molecules, including **selectins** and **integrins**. **Steps of Extravasation** 1. **Rolling** - **Role of Selectins**: Selectins are adhesion molecules expressed on the endothelial cells lining blood vessels. In response to inflammatory signals, endothelial cells upregulate selectins (such as E-selectin and P-selectin), which bind weakly to carbohydrates on leukocytes. This interaction causes leukocytes to \"roll\" along the vessel wall rather than flowing freely with the bloodstream. - **Process**: The rolling is a low-affinity interaction that allows leukocytes to slow down and make contact with the endothelial surface without immediately adhering firmly. 2. **Activation** - **Chemokine Release**: Chemokines are signaling molecules released by cells at the site of inflammation. These chemokines diffuse to the blood vessel wall, where they bind to receptors on the surface of rolling leukocytes. - **Leukocyte Activation**: The binding of chemokines to their receptors on leukocytes activates the leukocytes, leading to a change in the conformation of integrins (another type of adhesion molecule) on the leukocyte surface, preparing them for stronger binding. 3. **Firm Adhesion** - **Role of Integrins**: Integrins are adhesion molecules on leukocytes that mediate firm adhesion to endothelial cells. Once leukocytes are activated by chemokines, their integrins (such as LFA-1 and VLA-4) change conformation, increasing their affinity for their corresponding ligands (ICAM-1 and VCAM-1) on endothelial cells. - **Process**: This interaction results in firm adhesion, effectively \"stopping\" the rolling leukocyte and anchoring it to the endothelium. 4. **Transmigration (Diapedesis)** - **Process**: After adhering firmly to the endothelial cells, leukocytes extend through gaps between endothelial cells, entering the underlying tissue in a process called **diapedesis**. - **Mediators**: Various enzymes and cell junction proteins facilitate this process by loosening endothelial cell junctions, allowing leukocytes to squeeze through and migrate into the tissue. 5. **Migration to Site of Infection** - Once in the tissue, leukocytes follow a gradient of chemokines and other signals to move toward the site of infection or injury. **Summary of Key Molecules in Extravasation** **Molecule** **Location** **Role in Extravasation** ---------------- -------------------------- -------------------------------------------------- **Selectins** Endothelial cells Mediate rolling of leukocytes along vessel walls **Integrins** Leukocytes Facilitate firm adhesion to endothelial cells **Chemokines** Tissue and blood vessels Activate leukocytes and direct migration **Summary of Extravasation Process** Extravasation allows immune cells to exit the bloodstream and enter tissues to combat infection or injury. **Selectins** initiate the rolling interaction, **chemokines** activate the leukocytes and prepare them for binding, and **integrins** mediate firm adhesion, leading to transmigration through the endothelial barrier. This orchestrated process ensures immune cells reach sites of inflammation quickly and efficiently. Describe how corticosteroids and NSAIDs work to dampen inflammation **Corticosteroids** and **Non-Steroidal Anti-Inflammatory Drugs (NSAIDs)** are two types of medications commonly used to reduce inflammation, albeit through different mechanisms. Here's how each of them works: **1. Corticosteroids** - **Mechanism of Action**: - **Gene Regulation**: Corticosteroids, such as prednisone or dexamethasone, are synthetic forms of cortisol, a hormone produced by the adrenal glands. They work by entering cells and binding to **glucocorticoid receptors** in the cytoplasm. The corticosteroid-receptor complex then moves into the nucleus, where it binds to specific DNA regions and regulates the transcription of numerous genes involved in inflammation. - **Inhibition of Inflammatory Proteins**: Corticosteroids reduce the production of **pro-inflammatory cytokines** (e.g., IL-1, IL-6, TNF-α) and enzymes (e.g., cyclooxygenase-2 or COX-2, and phospholipase A2). By blocking these mediators, corticosteroids prevent the recruitment and activation of inflammatory cells. - **Reduction of Immune Cell Activity**: Corticosteroids inhibit the activity and migration of various immune cells, including lymphocytes, macrophages, and neutrophils, further dampening the immune response. - **Effects**: - **Decreased Production of Prostaglandins and Leukotrienes**: By inhibiting phospholipase A2, corticosteroids prevent the production of arachidonic acid, a precursor to prostaglandins and leukotrienes, which are key mediators of inflammation. - **Suppression of Immune Response**: By reducing cytokine production and immune cell activity, corticosteroids lower the inflammatory response in a broad and potent manner, making them effective for treating autoimmune and inflammatory conditions. - **Applications**: Corticosteroids are used to treat a range of inflammatory conditions, such as asthma, rheumatoid arthritis, and autoimmune diseases. However, due to their broad immune suppression, they can lead to side effects, especially with long-term use. **2. Non-Steroidal Anti-Inflammatory Drugs (NSAIDs)** - **Mechanism of Action**: - **Inhibition of Cyclooxygenase (COX) Enzymes**: NSAIDs, such as ibuprofen, aspirin, and naproxen, work by inhibiting **cyclooxygenase (COX)** enzymes, specifically COX-1 and COX-2. COX enzymes are involved in the conversion of arachidonic acid into **prostaglandins**, which play a central role in promoting inflammation, pain, and fever. - **COX-1**: This enzyme is constitutively active and involved in producing prostaglandins that protect the stomach lining, maintain kidney function, and support platelet function. - **COX-2**: This enzyme is induced primarily during inflammation, leading to increased production of pro-inflammatory prostaglandins. - **Blocking Prostaglandin Production**: By inhibiting COX enzymes, NSAIDs decrease the production of pro-inflammatory prostaglandins, reducing inflammation, pain, and fever. - **Effects**: - **Anti-Inflammatory**: Reduction of prostaglandins results in decreased vasodilation, permeability, and recruitment of inflammatory cells at the site of injury. - **Analgesic and Antipyretic**: NSAIDs also provide pain relief and reduce fever by blocking prostaglandins involved in pain perception and temperature regulation in the hypothalamus. - **Applications**: NSAIDs are commonly used for mild to moderate inflammation, pain, and fever, such as in arthritis, muscle strains, and headaches. Since NSAIDs primarily target COX-2 during inflammation, selective COX-2 inhibitors (e.g., celecoxib) were developed to provide anti-inflammatory effects with fewer gastrointestinal side effects associated with COX-1 inhibition. **Comparison of Corticosteroids and NSAIDs** **Feature** **Corticosteroids** **NSAIDs** ---------------------------- --------------------------------------------------------------------------------------------- ---------------------------------------------------------------- **Primary Target** Glucocorticoid receptors and gene expression COX-1 and COX-2 enzymes **Mechanism of Action** Inhibits cytokine production, immune cell activity, and prostaglandin/leukotriene synthesis Blocks prostaglandin synthesis **Effect on Immune Cells** Broad suppression Limited effect on immune cells **Common Uses** Autoimmune diseases, severe inflammation Mild to moderate inflammation, pain, fever **Side Effects** Broad immune suppression, adrenal suppression, osteoporosis with long-term use GI irritation, kidney issues, bleeding risk (COX-1 inhibition) In summary: - **Corticosteroids** provide broad and potent suppression of inflammation by targeting multiple inflammatory pathways, making them effective for severe or chronic inflammation but with higher risks of side effects. - **NSAIDs** specifically target COX enzymes to reduce prostaglandin production, effectively reducing inflammation, pain, and fever in a more targeted manner with fewer immune-suppressive effects than corticosteroids. Know the structure and functions of each of the 5 classes of immunoglobulins Immunoglobulins (Ig), also known as antibodies, are proteins produced by B cells in response to antigens. They have a fundamental role in the immune system, recognizing and neutralizing foreign substances. The five main classes of immunoglobulins---**IgG, IgM, IgA, IgE, and IgD**---each have unique structures and functions suited to different immune responses. **1. IgG (Immunoglobulin G)** - **Structure**: IgG is a **monomer** (a single antibody unit) with two antigen-binding sites. It is the most abundant antibody in serum, comprising about 75-80% of the immunoglobulins in the blood. - **Function**: - **Primary Role in Humoral Immunity**: IgG is the main antibody involved in secondary immune responses and provides long-term immunity. - **Neutralization of Toxins and Pathogens**: IgG binds directly to pathogens and toxins, preventing them from infecting cells. - **Opsonization**: IgG coats pathogens, enhancing phagocytosis by macrophages and neutrophils. - **Activation of the Complement System**: IgG can activate complement proteins, aiding in the destruction of pathogens. - **Crosses the Placenta**: IgG is the only antibody class that can cross the placenta, providing passive immunity to the fetus. **2. IgM (Immunoglobulin M)** - **Structure**: IgM is typically a **pentamer**, consisting of five antibody units linked together, with a total of ten antigen-binding sites. This large structure makes IgM highly effective at binding antigens. - **Function**: - **Primary Antibody in Initial Immune Response**: IgM is the first antibody produced in response to an infection and is crucial in the primary immune response. - **Effective in Agglutination and Neutralization**: Its pentameric structure allows IgM to cross-link multiple antigens, making it very effective in agglutinating (clumping) pathogens for easier elimination. - **Activates the Complement System**: IgM strongly activates the complement system, promoting pathogen lysis and phagocytosis. - **Present on B Cell Surfaces**: IgM serves as a receptor on the surface of naïve B cells, where it recognizes antigens and initiates B cell activation. **3. IgA (Immunoglobulin A)** - **Structure**: IgA is typically a **dimer** (two antibody units linked) in mucosal tissues, with four antigen-binding sites. It exists as a monomer in serum but is secreted as a dimer in mucosal areas. - **Function**: - **Mucosal Immunity**: IgA is the primary antibody found in mucosal secretions, such as saliva, tears, breast milk, and mucous. It provides immune defense on mucosal surfaces like the respiratory, gastrointestinal, and urogenital tracts. - **Neutralization of Pathogens**: IgA neutralizes pathogens by binding to them and preventing their adherence to mucosal surfaces. - **Passive Immunity in Infants**: IgA in breast milk provides passive immunity to infants by protecting their gastrointestinal tract from infections. **4. IgE (Immunoglobulin E)** - **Structure**: IgE is a **monomer** with two antigen-binding sites. It is present in very low concentrations in the blood compared to other immunoglobulins. - **Function**: - **Allergic Reactions**: IgE is involved in allergic responses and binds to allergens. Upon binding, it triggers degranulation of **mast cells** and **basophils**, which release histamines and other inflammatory mediators, causing allergic symptoms. - **Protection Against Parasites**: IgE plays a critical role in defending against parasitic infections, particularly helminths (worms), by triggering an inflammatory response that helps expel the parasites from the body. **5. IgD (Immunoglobulin D)** - **Structure**: IgD is a **monomer** with two antigen-binding sites and is found mainly on the surface of naïve B cells. - **Function**: - **B Cell Receptor**: IgD serves as a receptor on the surface of immature B cells and helps in the activation and differentiation of B cells upon encountering an antigen. - **Role in Immune Surveillance**: Although IgD's exact function is not fully understood, it is thought to play a role in respiratory immune defense and in initiating immune responses in some tissues. **Summary Table** **Immunoglobulin** **Structure** **Primary Location** **Key Functions** -------------------- ------------------------------------------- ---------------------------------------- --------------------------------------------------------------- **IgG** Monomer Blood, tissues, crosses placenta Long-term immunity, opsonization, complement activation **IgM** Pentamer Blood Primary immune response, agglutination, complement activation **IgA** Dimer (in secretions), Monomer (in serum) Mucosal areas, secretions, breast milk Mucosal immunity, neutralization, passive immunity to infants **IgE** Monomer Blood, bound to mast cells/basophils Allergic reactions, defense against parasites **IgD** Monomer B cell surfaces B cell receptor, role in B cell activation In summary, each immunoglobulin class has a unique structure and function that enables the immune system to address a wide range of pathogens and immune challenges, from initial infection to long-term immunity and mucosal defense to allergy and parasitic responses. Know the general maturation process of B cells, including the function of RAG, the process of somatic recombination, and selection events The **maturation of B cells** is a complex process that takes place primarily in the **bone marrow** and involves multiple steps to ensure the development of functional and self-tolerant B cells. The process includes the rearrangement of the immunoglobulin (Ig) genes to generate a diverse B cell receptor (BCR) repertoire, as well as selection events to ensure only appropriate B cells mature and exit into the peripheral blood. **Key Steps in B Cell Maturation** 1. **Gene Rearrangement and the Role of RAG** - **Recombination Activating Genes (RAG)**: The RAG-1 and RAG-2 proteins are essential for initiating **somatic recombination**, the process by which gene segments of the immunoglobulin genes rearrange to produce a unique B cell receptor for each B cell. - **Somatic Recombination**: - This process involves recombining the **variable (V), diversity (D), and joining (J)** segments of the heavy chain (and the V and J segments of the light chain) to create a unique variable region that defines the specificity of the BCR. - **Heavy Chain Rearrangement**: In the early stages of maturation, the D and J gene segments of the immunoglobulin heavy chain rearrange first, followed by the joining of a V segment to the DJ segment. If successful, this produces a functional **heavy chain**. - **Light Chain Rearrangement**: After a functional heavy chain is produced, the cell begins rearranging the V and J segments of the immunoglobulin light chain genes to produce a functional **light chain**. - **Role of RAG Proteins**: RAG-1 and RAG-2 recognize specific DNA sequences and cut the DNA, facilitating the rearrangement of these gene segments. This process generates a large diversity of BCRs, allowing the immune system to recognize many different antigens. 2. **Checkpoint 1: Pre-B Cell Receptor Formation** - After successful heavy chain rearrangement, the heavy chain pairs with a surrogate light chain to form a **pre-B cell receptor (pre-BCR)**. - **Function of Pre-BCR**: The pre-BCR signals the cell to stop further heavy chain rearrangement (allelic exclusion) and proceed to light chain rearrangement. This checkpoint ensures that each B cell expresses only one type of heavy chain. 3. **Light Chain Rearrangement and BCR Formation** - After passing the pre-BCR checkpoint, the B cell rearranges the light chain gene segments (V and J) to form a functional light chain. - Once a functional light chain is produced, it combines with the heavy chain to form the **B cell receptor (BCR)** on the surface of the immature B cell. - This completed BCR gives the B cell its specificity and allows it to recognize a unique antigen. 4. **Selection Events** - **Positive Selection (Functional Testing)**: Only B cells that successfully rearrange both heavy and light chains to produce a functional BCR continue to develop. Cells that fail to produce a functional BCR undergo apoptosis. - **Negative Selection (Self-Tolerance)**: Immature B cells are tested for **self-reactivity**. Those that strongly bind to self-antigens in the bone marrow are either eliminated through apoptosis (clonal deletion), undergo receptor editing (further rearrangement of the light chain genes to change their specificity), or become anergic (rendered nonresponsive). - This process is essential to prevent the development of self-reactive B cells that could cause autoimmune diseases. 5. **Final Maturation and Peripheral Circulation** - After passing both selection checkpoints, the surviving immature B cells exit the bone marrow and migrate to peripheral lymphoid tissues, such as the spleen and lymph nodes. - In these tissues, they complete their maturation and become **naïve mature B cells**, ready to respond to specific antigens they may encounter. **Summary of Key Components and Processes in B Cell Maturation** **Step** **Key Component/Process** **Function** ------------------------------- ------------------------------ ----------------------------------------------------------------- **Gene Rearrangement** **RAG-1 and RAG-2 Proteins** Initiate somatic recombination for BCR diversity **Heavy Chain Rearrangement** **Somatic Recombination** Creates unique heavy chain for BCR specificity **Pre-BCR Checkpoint** **Pre-B Cell Receptor** Ensures functional heavy chain before light chain rearrangement **Light Chain Rearrangement** **V and J Gene Segments** Finalizes BCR specificity **Positive Selection** Functional Testing Ensures functional BCR **Negative Selection** Self-Tolerance Testing Eliminates or edits self-reactive B cells **Peripheral Maturation** Final Maturation Prepares mature B cells for antigen encounter **Key Concepts** - **Somatic Recombination**: Creates a diverse B cell receptor repertoire by randomly combining gene segments. - **RAG Proteins**: Crucial for initiating BCR gene rearrangement. - **Selection Events**: Ensure only functional and non-self-reactive B cells mature and enter circulation. This maturation process generates a pool of diverse, functional, and self-tolerant B cells capable of responding to foreign antigens, a cornerstone of adaptive immunity. Identify B cell activation signals and be able to describe the major events occurring during B cell activation **B cell activation** is a multi-step process that enables B cells to respond to specific antigens effectively. Activation typically occurs in secondary lymphoid tissues (e.g., lymph nodes, spleen) and requires two main signals to ensure specificity and regulation. Here's an overview of the **activation signals** and **major events** that occur during B cell activation. **Key Activation Signals for B Cells** 1. **Signal 1: Antigen Recognition** - **B Cell Receptor (BCR) Binding to Antigen**: - The first signal in B cell activation occurs when the B cell receptor (BCR), which is specific for a particular antigen, binds to that antigen. This antigen can be a protein, carbohydrate, or other structure. - **Cross-Linking of BCRs**: To initiate signaling, multiple BCRs must be cross-linked (bound to multiple copies of an antigen) on the B cell surface. This clustering initiates downstream signaling events. - **Intracellular Signaling Cascade**: Once the BCR binds an antigen, intracellular signaling molecules (such as **Igα** and **Igβ**) activate downstream signaling pathways, including **Src-family kinases** and **Syk kinase**, leading to phosphorylation and activation of several proteins within the B cell. 2. **Signal 2: Costimulation** - **T-Dependent Antigen (Most Protein Antigens)**: - For many antigens, B cells require assistance from **helper T cells (Th cells)**, specifically CD4+ T cells, to receive a second activation signal. This type of activation is referred to as **T-dependent** activation. - **Interaction with Helper T Cells**: After binding to an antigen, the B cell internalizes it, processes it, and presents a peptide fragment on its surface in complex with **MHC class II** molecules. - **CD40-CD40L Interaction**: The activated helper T cell binds to the antigen-MHC complex on the B cell and provides the critical second signal through the **CD40 ligand (CD40L)** on the T cell binding to **CD40** on the B cell. This interaction is essential for full activation and proliferation of the B cell. - **Cytokine Release**: The helper T cell also releases cytokines (e.g., IL-4, IL-5, IL-6), which further stimulate B cell proliferation, differentiation, and antibody class switching. - **T-Independent Antigen (Polysaccharides and Repetitive Antigens)**: - Some antigens, particularly **polysaccharides** or **repetitive structures** (e.g., bacterial cell wall components), can activate B cells without T cell help. This is known as **T-independent** activation. - These antigens can cross-link multiple BCRs strongly enough to provide a second activation signal, leading to B cell activation, although this response is typically weaker and limited to the production of **IgM** antibodies without class switching or the formation of memory cells. **Major Events in B Cell Activation** 1. **Clonal Expansion** - Once fully activated, B cells begin to proliferate, creating many clones of the original B cell that recognized the antigen. This **clonal expansion** generates a large population of B cells with the same antigen specificity, increasing the immune system's ability to respond to the specific pathogen. 2. **Differentiation into Plasma Cells and Memory B Cells** - **Plasma Cells**: Most of the activated B cells differentiate into **plasma cells**, which are antibody-secreting cells. Plasma cells leave the lymphoid tissues and move to the bone marrow or other sites, where they produce large quantities of antibodies that circulate in the blood and lymph, targeting the pathogen for elimination. - **Memory B Cells**: A subset of activated B cells differentiates into **memory B cells**. These cells do not immediately produce antibodies but remain in the body long-term, providing a quicker and more robust response if the same antigen is encountered again in the future. 3. **Antibody Class Switching (Isotype Switching)** - **Function**: Upon activation, B cells can undergo **class switching** (or isotype switching), changing the class of antibody they produce from IgM to another class (e.g., IgG, IgA, or IgE) with help from cytokines produced by helper T cells. - **Mechanism**: Class switching is directed by cytokines (e.g., IL-4 promotes switching to IgE, TGF-β to IgA) and allows the immune system to tailor the antibody response to different types of pathogens or tissue environments. - **Result**: Class switching maintains the antibody's antigen specificity while allowing it to perform different functions (e.g., IgG for opsonization, IgA for mucosal immunity). 4. **Affinity Maturation** - **Process**: During the response, B cells undergo **somatic hypermutation** in their antibody variable regions, introducing small mutations that can improve antigen binding. - **Selection**: B cells with higher-affinity receptors for the antigen are preferentially selected to continue dividing and differentiating. This process, known as **affinity maturation**, enhances the effectiveness of the antibody response over time. **Summary of B Cell Activation** **Step** **Description** **Outcome** ------------------------------- ----------------------------------------------------------------------------------------------------- ---------------------------------------------------------------------------- **Signal 1: Antigen Binding** BCR binds to antigen, initiating signaling Activation begins **Signal 2: Costimulation** CD40-CD40L interaction with helper T cell (T-dependent) or strong BCR cross-linking (T-independent) Full activation, proliferation **Clonal Expansion** Proliferation of B cells with same specificity Increased number of antigen-specific B cells **Differentiation** B cells become plasma cells or memory B cells Plasma cells produce antibodies, memory B cells remain for future response **Class Switching** Switching from IgM to other antibody classes Tailored immune response based on pathogen and location **Affinity Maturation** Somatic hypermutation selects for high-affinity antibodies Improved antibody effectiveness In summary, B cell activation is a complex process that ensures a strong, specific, and adaptable antibody response to pathogens. T-dependent activation requires T cell help and leads to a more robust immune response, including antibody class switching, affinity maturation, and memory cell formation, while T-independent activation produces a rapid but less diverse antibody response. Be able to describe the processes of class-switching and affinity maturation in B cells and know the cytokines that stimulate class-switching to IgA and IgE Class-switching and affinity maturation are two critical processes in B cell development that enhance the immune system\'s adaptability and specificity in fighting infections. Here's a detailed explanation of each process, including the cytokines involved in class-switching to **IgA** and **IgE**. **1. Class-Switching (Isotype Switching)** - **Definition**: Class-switching (or isotype switching) is the process by which a B cell changes the class (isotype) of antibody it produces without altering the antigen specificity. This allows the immune system to produce antibodies with different functions tailored to specific types of pathogens and tissue environments. - **Mechanism**: - **Initiation**: Class-switching is initiated after B cell activation, particularly in response to T-dependent antigens, where B cells receive help from **CD4+ helper T cells**. - **Role of Cytokines**: Cytokines secreted by helper T cells play a critical role in directing class-switching to specific antibody classes by activating transcription factors that open up certain regions of the immunoglobulin heavy chain gene locus. - **Switch Regions**: B cells have **switch (S) regions** in their DNA located upstream of each heavy-chain constant region gene (except for IgD). During class-switching, an enzyme called **Activation-Induced Cytidine Deaminase (AID)** creates double-strand breaks in these S regions. The DNA between the selected S region and the previously expressed IgM S region is then looped out and deleted. - **Result**: The B cell produces antibodies of a different class (e.g., IgG, IgA, or IgE) with the same antigen specificity as the original IgM antibodies, allowing it to respond more effectively to specific types of infections or conditions. - **Cytokines that Stimulate Class-Switching**: - **IgA**: **Transforming Growth Factor-beta (TGF-β)** is the primary cytokine that stimulates class-switching to IgA, which is especially important for mucosal immunity in areas such as the respiratory and gastrointestinal tracts. - **IgE**: **Interleukin-4 (IL-4)** is the main cytokine that induces class-switching to IgE, which plays a key role in responses against parasitic infections and in allergic reactions. - **Functional Implications**: - **IgG** (stimulated by cytokines like IFN-γ): Effective in opsonization, neutralization, and complement activation. - **IgA** (stimulated by TGF-β): Provides protection on mucosal surfaces by neutralizing pathogens. - **IgE** (stimulated by IL-4): Important in defense against parasites and involved in allergic responses by binding to mast cells and basophils. **2. Affinity Maturation** - **Definition**: Affinity maturation is a process that increases the binding affinity of antibodies for their specific antigens over time, allowing the immune response to become more effective during an ongoing infection. - **Mechanism**: - **Somatic Hypermutation**: Affinity maturation occurs through **somatic hypermutation**, a process in which the **variable regions** of the immunoglobulin genes in activated B cells undergo a high rate of point mutations. This process is also mediated by **Activation-Induced Cytidine Deaminase (AID)**. - **Selection for High-Affinity B Cells**: These mutations lead to a diverse population of B cells with varying affinities for the antigen. B cells with higher-affinity receptors for the antigen are selectively favored and continue to proliferate, while those with lower affinities may undergo apoptosis. - **Role of Follicular Dendritic Cells and T Follicular Helper Cells (Tfh)**: In the germinal centers of lymphoid tissues, follicular dendritic cells present antigen to B cells. B cells with higher-affinity receptors can bind the antigen more effectively, receiving survival signals from T follicular helper cells (Tfh). This selection process results in a population of B cells producing antibodies with significantly increased affinity. - **Outcome**: - Affinity maturation ensures that as an immune response progresses, the antibodies produced by plasma cells become increasingly effective at neutralizing and eliminating the pathogen. - Memory B cells that arise from this process also carry high-affinity receptors, which allows for a quicker and more effective response if the body encounters the same antigen in the future. **Summary of Class-Switching and Affinity Maturation** **Process** **Description** **Key Cytokines** **Result** ------------------------- ------------------------------------------------------------- ------------------------------------------------------------------- -------------------------------------------------------------------------- **Class-Switching** Change of antibody isotype without altering specificity **IgA**: TGF-β, **IgE**: IL-4 Production of IgG, IgA, or IgE antibodies suited for different functions **Affinity Maturation** Increase in antibody affinity through somatic hypermutation Not cytokine-driven (dependent on antigen exposure and selection) Production of high-affinity antibodies, improving immune response In summary, **class-switching** allows B cells to produce antibody classes that are better suited for specific immune functions, directed by cytokines such as TGF-β for IgA and IL-4 for IgE. **Affinity maturation** enhances the quality of antibodies by selecting B cells with high-affinity receptors, ensuring an optimized immune response against the pathogen. Section 2 Be able to describe how each complement pathway is activated (i.e., initiated) The complement system consists of a group of proteins that play a key role in immune defense, particularly in enhancing (or \"complementing\") the ability of antibodies and phagocytic cells to clear pathogens. There are three main complement pathways, each with its unique activation mechanism: **1. Classical Pathway** - **Initiation**: The classical pathway is activated when the C1 complex binds to antibodies (typically IgG or IgM) that are attached to antigens on the surface of pathogens. - **Key Trigger**: This binding activates C1, a complex made up of C1q, C1r, and C1s proteins, which then cleaves C4 and C2 to form the C3 convertase, starting the cascade. - **Specificity**: This pathway is adaptive, as it relies on antibodies produced by the immune system in response to specific pathogens. **2. Lectin Pathway** - **Initiation**: The lectin pathway is activated when mannose-binding lectin (MBL) or ficolins bind to specific carbohydrate residues (mannose or other sugars) on the surface of pathogens. - **Key Trigger**: Once MBL binds to these sugars, it activates associated serine proteases (MASP-1 and MASP-2), which then cleave C4 and C2 to form the C3 convertase. - **Specificity**: This pathway is part of the innate immune system and does not rely on antibodies; instead, it recognizes common microbial surface sugars. **3. Alternative Pathway** - **Initiation**: The alternative pathway is continuously active at a low level through the spontaneous hydrolysis of C3 (referred to as \"tickover\") in plasma. - **Key Trigger**: When C3b, a product of C3 hydrolysis, binds to microbial surfaces, it is stabilized by factors B and D, forming the C3 convertase. This amplifies the production of C3b and leads to further complement activation. - **Specificity**: It is also part of the innate immune system and does not require antibodies or specific pathogen recognition. Each pathway ultimately leads to the formation of C3 convertase, a key enzyme that cleaves C3, generating C3a (which has inflammatory roles) and C3b (which promotes opsonization and formation of the membrane attack complex). This convergence of pathways amplifies the immune response against pathogens. Understand the three main functions of complement and which active complement proteins participate in each of these three functions The complement system has three main functions essential for immune defense: opsonization, inflammation, and cell lysis. Each function is facilitated by specific complement proteins, which work together to enhance the immune system's response to pathogens. **1. Opsonization** - **Function**: Opsonization tags pathogens for easier recognition and ingestion by phagocytes, like macrophages and neutrophils. This process enhances phagocytosis by making the pathogen more \"appealing\" to immune cells. - **Key Complement Protein**: **C3b** is the primary opsonin in the complement system. Once it binds to the surface of pathogens, it serves as a \"tag\" recognized by complement receptors on phagocytic cells, promoting phagocytosis. **2. Inflammation (Anaphylatoxin Production)** - **Function**: Complement proteins can stimulate inflammation by attracting immune cells to the site of infection and promoting the release of inflammatory molecules. - **Key Complement Proteins**: **C3a**, **C4a**, and **C5a** are known as anaphylatoxins, which promote inflammation. C5a, in particular, is a potent chemoattractant that recruits neutrophils and other immune cells to the site of infection, while C3a and C4a contribute to increased vascular permeability and the release of histamine from mast cells. **3. Cell Lysis (Membrane Attack Complex)** - **Function**: The complement system can directly lyse pathogen cells by forming a pore in their membrane, leading to cell death. - **Key Complement Proteins**: **C5b**, **C6**, **C7**, **C8**, and multiple **C9** molecules assemble to form the Membrane Attack Complex (MAC). The MAC creates a pore in the membrane of the pathogen, disrupting its integrity and ultimately causing lysis of the pathogen. These three functions---opsonization, inflammation, and cell lysis---work in concert to identify, recruit immune cells to, and eliminate pathogens, enhancing the body's ability to clear infections. Understand the function of MHC molecules and identify which cells express each MHC class MHC (Major Histocompatibility Complex) molecules play a critical role in the immune system by presenting antigenic peptides to T cells, allowing the immune system to recognize and respond to pathogens or infected cells. There are two main classes of MHC molecules, each with distinct roles and expression patterns. **1. MHC Class I** - **Function**: MHC class I molecules present endogenous antigens---peptides from proteins found inside the cell (e.g., viral proteins or abnormal peptides in cancer cells). They display these peptides on the cell surface for recognition by **CD8+ cytotoxic T cells**, which can then destroy infected or abnormal cells. - **Expressed By**: MHC class I molecules are expressed by **all nucleated cells** in the body. This broad expression allows nearly all cells to present intracellular antigens and be targeted for destruction if infected or abnormal. **2. MHC Class II** - **Function**: MHC class II molecules present exogenous antigens---peptides derived from extracellular sources, like pathogens that have been phagocytosed by immune cells. They display these peptides to **CD4+ helper T cells**, which then help orchestrate an immune response by activating other immune cells, including B cells and macrophages. - **Expressed By**: MHC class II molecules are expressed primarily by **professional antigen-presenting cells (APCs)**, which include **dendritic cells, macrophages, and B cells**. This restricted expression ensures that helper T cells are activated by specialized immune cells capable of triggering an adaptive immune response. **Key Differences** - **MHC Class I** is involved in targeting infected or abnormal cells for destruction and is widely expressed. - **MHC Class II** is involved in activating helper T cells to coordinate an immune response and is restricted to professional antigen-presenting cells. Together, MHC class I and II molecules ensure that the immune system can respond to both intracellular and extracellular pathogens effectively. Describe antigen processing and presentation in both Class I and Class II MHC, including knowing which MHC class presents to which T cell subset Antigen processing and presentation are essential processes by which cells display antigenic peptides on their surface via MHC molecules. This presentation allows T cells to recognize and respond to pathogens or abnormal cells. The processes differ between MHC class I and MHC class II, each presenting to a distinct subset of T cells. **1. MHC Class I Antigen Processing and Presentation** - **Process**: 1. **Intracellular Antigens**: MHC class I molecules present endogenous antigens, which are typically proteins synthesized within the cell, such as viral proteins during an infection or abnormal proteins in cancer cells. 2. **Proteasome Degradation**: These intracellular proteins are degraded into peptide fragments by the **proteasome**, a protein complex responsible for breaking down unwanted or damaged proteins. 3. **Transport to ER**: The peptide fragments are transported into the **endoplasmic reticulum (ER)** via the **TAP (Transporter Associated with Antigen Processing)** complex, which selectively transfers peptides into the ER. 4. **Loading onto MHC Class I**: Inside the ER, peptides are loaded onto MHC class I molecules. 5. **Transport to Cell Surface**: The MHC class I-peptide complex is then transported to the cell surface via the Golgi apparatus. - **Presentation**: MHC class I molecules present these peptides to **CD8+ cytotoxic T cells**, which can then recognize and kill infected or abnormal cells. **2. MHC Class II Antigen Processing and Presentation** - **Process**: 1. **Extracellular Antigens**: MHC class II molecules present exogenous antigens, which are antigens from pathogens or other sources outside the cell. 2. **Endocytosis/Phagocytosis**: Professional antigen-presenting cells (APCs) such as dendritic cells, macrophages, and B cells engulf extracellular pathogens or antigens through endocytosis or phagocytosis. 3. **Lysosomal Degradation**: The engulfed material is degraded into peptide fragments within **lysosomes**. 4. **Loading onto MHC Class II**: The MHC class II molecules, synthesized in the ER and transported to the endosomal/lysosomal compartment, are loaded with the antigenic peptides. The invariant chain, a protein that prevents premature peptide binding in the ER, is removed in the lysosomal environment to allow peptide loading. 5. **Transport to Cell Surface**: The MHC class II-peptide complex is transported to the cell surface for presentation. - **Presentation**: MHC class II molecules present these peptides to **CD4+ helper T cells**, which then help activate other immune cells, such as B cells and macrophages, to coordinate a broader immune response. **Summary of MHC Class Functions** - **MHC Class I**: - Presents **endogenous** (intracellular) antigens. - Interacts with **CD8+ cytotoxic T cells**. - Found on all nucleated cells. - **MHC Class II**: - Presents **exogenous** (extracellular) antigens. - Interacts with **CD4+ helper T cells**. - Expressed by professional antigen-presenting cells (APCs) only. This system allows MHC molecules to alert the immune system to different types of threats, with MHC class I focusing on intracellular pathogens and MHC class II on extracellular threats. Compare and contrast the two pathways of antigen processing and presentation, focusing on the functions of proteasomes, TAP, and lysosomes The pathways of antigen processing and presentation for MHC class I and MHC class II molecules have distinct mechanisms, each tailored to presenting either intracellular or extracellular antigens. Here's a breakdown of the differences and similarities, focusing on the roles of the **proteasome**, **TAP**, and **lysosomes**: **MHC Class I Pathway (Endogenous Pathway)** - **Function**: Presents intracellular antigens, typically derived from proteins synthesized within the cell, such as viral proteins or tumor antigens. - **Antigen Processing**: - **Proteasome**: The proteasome is a large protein complex that degrades endogenous proteins within the cell into small peptide fragments. These fragments are usually 8-10 amino acids long, suitable for loading onto MHC class I molecules. - **TAP (Transporter Associated with Antigen Processing)**: TAP is a transport protein located in the membrane of the endoplasmic reticulum (ER). It selectively transports peptide fragments from the cytosol into the ER. TAP's role is crucial for the MHC class I pathway, as it allows peptides to reach the ER, where they are loaded onto MHC class I molecules. - **Loading and Presentation**: Once inside the ER, peptides are loaded onto MHC class I molecules. The MHC-peptide complexes are then transported to the cell surface for presentation to **CD8+ cytotoxic T cells**. - **Summary**: In this pathway, the **proteasome** and **TAP** are essential components. The proteasome generates peptide fragments, while TAP transports these fragments to the ER for loading onto MHC class I molecules. **MHC Class II Pathway (Exogenous Pathway)** - **Function**: Presents extracellular antigens, typically from pathogens or other external sources that have been engulfed by the cell. - **Antigen Processing**: - **Lysosomes**: After phagocytosis or endocytosis, the ingested material is enclosed within a phagosome or endosome, which fuses with a lysosome. Lysosomes contain digestive enzymes and an acidic environment that degrades the engulfed material into peptide fragments, which are typically 10-20 amino acids long. - **MHC Class II Loading**: MHC class II molecules are synthesized in the ER and transported to a specialized compartment, where they encounter the lysosome-derived peptides. Here, the invariant chain, a chaperone that blocks the MHC class II binding site until it reaches the proper compartment, is removed, allowing peptide loading. - **Presentation**: The MHC class II-peptide complexes are then transported to the cell surface for presentation to **CD4+ helper T cells**. - **Summary**: In this pathway, **lysosomes** are critical for degrading exogenous antigens. The acidic environment and enzymes within lysosomes break down the proteins into peptides suitable for loading onto MHC class II molecules. **Comparison of MHC Class I and Class II Pathways** **Feature** **MHC Class I Pathway** **MHC Class II Pathway** ----------------------- ------------------------------------------ ------------------------------------------ **Type of Antigen** Endogenous (intracellular) Exogenous (extracellular) **Cellular Location** Cytoplasm and ER Endosomes and lysosomes **Proteasome** Essential; degrades cytoplasmic proteins Not involved **TAP** Essential; transports peptides to ER Not involved **Lysosome** Not involved Essential; degrades endocytosed proteins **Presented To** CD8+ cytotoxic T cells CD4+ helper T cells **MHC Expression** All nucleated cells Professional APCs only **Key Differences** - **Proteasome and TAP** are unique to the MHC class I pathway, enabling it to process and present peptides from within the cell to cytotoxic T cells. - **Lysosomes** play a central role in the MHC class II pathway, breaking down extracellular proteins into peptides for presentation to helper T cells. By using distinct processing mechanisms, these pathways allow the immune system to recognize and respond to a wide range of pathogens, both inside and outside cells. Identify T cell activation signals and be able to describe the major events occurring during T cell activation T cell activation is a critical process in the adaptive immune response, enabling T cells to proliferate and differentiate into effector cells capable of combating infections. T cell activation requires multiple signals, each playing an essential role in ensuring a controlled and specific immune response. **Three Key Signals in T Cell Activation** 1. **Signal 1: Antigen Recognition via T Cell Receptor (TCR)** - **Mechanism**: The T cell receptor (TCR) on a naïve T cell binds to a specific antigenic peptide presented by an MHC molecule on an antigen-presenting cell (APC). - **CD4+ T cells** recognize peptides presented on **MHC class II** molecules (e.g., on dendritic cells, macrophages, and B cells). - **CD8+ T cells** recognize peptides presented on **MHC class I** molecules (on almost all nucleated cells). - **Purpose**: This interaction provides the specificity of the immune response, allowing T cells to recognize a specific pathogen-derived peptide. 2. **Signal 2: Co-stimulatory Signal** - **Mechanism**: Co-stimulatory molecules on the T cell surface interact with ligands on the APC. The most important interaction is between **CD28** on the T cell and **B7** molecules (CD80 and CD86) on the APC. - **Purpose**: Signal 2 is essential for full T cell activation and prevents T cells from becoming anergic (non-responsive) when encountering self-antigens. This signal ensures that T cells only respond to infections or danger signals. 3. **Signal 3: Cytokine Signaling** - **Mechanism**: After receiving the first two signals, cytokines are produced by the APCs and other immune cells, which then bind to cytokine receptors on the T cell surface. - **Purpose**: These cytokines provide context for the type of immune response needed. They influence T cell differentiation into specific subsets (e.g., Th1, Th2, Th17, Treg for CD4+ T cells) or promote the cytotoxic functions of CD8+ T cells. This signal is critical for tailoring the immune response to the nature of the pathogen. **Major Events During T Cell Activation** 1. **TCR-MHC Binding and Signal Transduction**: - When the TCR binds to its specific peptide-MHC complex, it initiates a signaling cascade within the T cell. This cascade involves **phosphorylation** of key proteins (e.g., through the action of the protein tyrosine kinase Lck), leading to the activation of downstream signaling pathways. 2. **Activation of Transcription Factors**: - Signal transduction from TCR engagement leads to the activation of transcription factors such as **NF-κB**, **NFAT (Nuclear Factor of Activated T cells)**, and **AP-1**. These transcription factors move into the nucleus and initiate the transcription of genes necessary for T cell proliferation, differentiation, and effector function. 3. **Clonal Expansion and Proliferation**: - The activated T cell starts to proliferate, undergoing multiple rounds of cell division. This clonal expansion produces a large number of T cells that are specific for the antigen, increasing the body\'s ability to respond to the pathogen. 4. **Differentiation into Effector and Memory T Cells**: - **Effector T cells**: CD4+ T cells differentiate into specific helper T cell subsets (e.g., Th1, Th2, Th17, Treg) depending on the cytokines present, each with a specialized function. CD8+ T cells differentiate into cytotoxic T lymphocytes (CTLs) capable of killing infected cells. - **Memory T cells**: Some activated T cells become memory T cells, which persist in the body long-term. They respond more quickly and robustly if the antigen is encountered again in the future. 5. **Cytokine Production and Effector Functions**: - Activated T cells begin producing cytokines. CD4+ helper T cells secrete cytokines that activate B cells, macrophages, and other T cells, enhancing the immune response. CD8+ cytotoxic T cells release perforin and granzymes, directly killing infected or abnormal cells. **Summary of T Cell Activation Process** - **Signal 1 (Antigen Recognition)**: Provides specificity. - **Signal 2 (Co-stimulation)**: Confirms the presence of a pathogen, preventing autoimmunity. - **Signal 3 (Cytokine Signaling)**: Tailors the immune response and drives differentiation. This multistep activation ensures that T cells respond appropriately and effectively, adapting to different pathogens while maintaining control to prevent excessive or inappropriate immune responses. Identify how CD4+ helper T cells and CD8+ cytotoxic T cells bind to target cells CD4+ helper T cells and CD8+ cytotoxic T cells bind to target cells through interactions between their T cell receptors (TCRs) and major histocompatibility complex (MHC) molecules on the surface of the target or antigen-presenting cell (APC). Each T cell subtype has a specific mechanism and binding target, ensuring that each cell type performs its distinct role in the immune response. **1. CD4+ Helper T Cells** - **Binding Target**: CD4+ T cells bind to **MHC class II** molecules, which present exogenous antigenic peptides derived from extracellular pathogens. - **Binding Mechanism**: - The T cell receptor (TCR) on CD4+ T cells recognizes and binds to a specific peptide-MHC class II complex presented by **professional antigen-presenting cells (APCs)**, such as dendritic cells, macrophages, and B cells. - The CD4 molecule on the helper T cell acts as a co-receptor, specifically binding to a non-peptide portion of the MHC class II molecule. This binding stabilizes the TCR-MHC interaction and strengthens the signal to activate the helper T cell. - **Outcome of Binding**: Upon binding, CD4+ helper T cells receive activation signals, leading them to proliferate and differentiate into various subsets (e.g., Th1, Th2, Th17, Treg), which coordinate and enhance different aspects of the immune response by secreting cytokines and activating other immune cells like B cells and macrophages. **2. CD8+ Cytotoxic T Cells** - **Binding Target**: CD8+ T cells bind to **MHC class I** molecules, which present endogenous antigenic peptides derived from proteins synthesized within the cell, such as viral proteins or tumor antigens. - **Binding Mechanism**: - The TCR on CD8+ T cells recognizes and binds to a specific peptide-MHC class I complex presented by **all nucleated cells**, as nearly all cells express MHC class I molecules. - The CD8 molecule on the cytotoxic T cell serves as a co-receptor, binding to a specific region on the MHC class I molecule. This interaction stabilizes the TCR-MHC class I binding, facilitating the cytotoxic T cell's activation. - **Outcome of Binding**: Once bound to a target cell displaying a foreign peptide via MHC class I, the activated CD8+ cytotoxic T cell releases cytotoxic molecules, such as **perforin** and **granzymes**. Perforin forms pores in the target cell membrane, and granzymes enter through these pores to induce apoptosis, effectively killing infected or abnormal cells. **Summary of Binding Specificity** - **CD4+ helper T cells** bind to **MHC class II** molecules on APCs, using the CD4 molecule as a co-receptor, and act by coordinating and amplifying immune responses. - **CD8+ cytotoxic T cells** bind to **MHC class I** molecules on all nucleated cells, using the CD8 molecule as a co-receptor, and directly kill infected or abnormal cells. This specificity ensures that CD4+ T cells mainly assist other immune cells, while CD8+ T cells target and eliminate cells presenting intracellular pathogens or cancer-associated peptides, providing a robust, targeted immune response. Describe how NK cells function to recognize and kill cells Natural Killer (NK) cells are a type of lymphocyte in the innate immune system with the primary function of recognizing and killing infected, stressed, or abnormal cells, including tumor cells. NK cells have a unique mechanism of action that does not rely on specific antigen recognition, allowing them to respond rapidly to threats without prior sensitization. **Mechanisms of NK Cell Recognition and Activation** NK cells use a balance of **activating** and **inhibitory receptors** to decide whether to kill a target cell. This system enables NK cells to detect abnormalities that may not be recognized by T cells, particularly changes in MHC class I expression often seen in infected or transformed cells. 1. **Inhibitory Receptors and \"Missing Self\" Recognition**: - NK cells possess inhibitory receptors that recognize **MHC class I molecules** on the surface of normal, healthy cells. - Most healthy cells express MHC class I molecules, which bind to inhibitory receptors on NK cells and send a "do not kill" signal, preventing the NK cell from initiating a cytotoxic response. - However, many viruses and cancerous transformations downregulate MHC class I expression to evade detection by cytotoxic CD8+ T cells. NK cells can detect this "missing self" (lack of MHC class I) and, in the absence of inhibitory signaling, become activated to kill the target cell. 2. **Activating Receptors and \"Induced Self\" Recognition**: - NK cells also have activating receptors that recognize stress-induced ligands on infected, stressed, or transformed cells. These ligands are often upregulated in response to infection, DNA damage, or cellular stress. - Some well-known activating receptors include **NKG2D** and **NKp30/NKp46**. When these receptors bind to their respective ligands, they send "kill" signals that promote NK cell activation. - If activating signals outweigh inhibitory signals, the NK cell initiates a cytotoxic response. **Mechanism of NK Cell-Mediated Killing** Once activated, NK cells can kill target cells using two main cytotoxic mechanisms: 1. **Release of Cytotoxic Granules**: - NK cells release cytotoxic granules containing **perforin** and **granzymes**. - **Perforin** forms pores in the target cell membrane, creating entry points for granzymes. - **Granzymes** are proteases that enter the target cell through the perforin-created pores and trigger apoptosis, leading to cell death. 2. **Death Receptor Pathway (Fas-FasL Interaction)**: - NK cells can also express **Fas ligand (FasL)**, which binds to **Fas receptors** on the target cell surface. - The Fas-FasL interaction activates a signaling cascade within the target cell that induces apoptosis through the caspase pathway. - This pathway is particularly important in killing cells that may resist cytotoxic granules. **Role of Cytokines in NK Cell Function** NK cells are also influenced by cytokines in the environment, which can enhance their activation and cytotoxic function. For instance: - **Interleukin-12 (IL-12)**, produced by macrophages, promotes NK cell activation and proliferation. - **Interferon-alpha (IFN-α)** and **Interferon-beta (IFN-β)**, produced in response to viral infections, also enhance NK cell cytotoxicity. **Summary of NK Cell Function** - **Recognition**: NK cells assess a balance between inhibitory signals (presence of MHC class I) and activating signals (stress-induced ligands). - **Activation**: Lack of MHC class I or the presence of activating signals on a target cell triggers NK cell activation. - **Killing**: Activated NK cells kill target cells by releasing cytotoxic granules (perforin and granzymes) or through the Fas-FasL pathway, both of which induce apoptosis in the target cell. NK cells, therefore, provide an essential defense against infected, stressed, or transformed cells, especially when these cells attempt to evade the adaptive immune response. Describe the general steps in immediate innate, induced innate, and adaptive immunity to pathogens The immune response to pathogens occurs in a coordinated sequence of events that can be divided into three phases: immediate innate immunity, induced innate immunity, and adaptive immunity. Each phase has distinct mechanisms, timelines, and cell types involved, allowing for a rapid and escalated response to control and eliminate infections. **1. Immediate Innate Immunity** - **Timeline**: 0--4 hours after infection. - **Primary Components**: Pre-existing immune defenses, including physical barriers, antimicrobial peptides, and resident immune cells like macrophages and natural killer (NK) cells. - **Steps**: 1. **Barrier Defenses**: Physical barriers like skin and mucous membranes prevent pathogen entry. Chemical defenses, such as enzymes (e.g., lysozyme in saliva and tears) and acidic environments (e.g., stomach acid), create inhospitable conditions for pathogens. 2. **Pre-formed Effector Molecules**: If pathogens breach these barriers, they encounter pre-existing immune molecules in tissues and blood, including complement proteins and antimicrobial peptides, which can kill or neutralize pathogens directly. 3. **Resident Immune Cells**: Resident macrophages and dendritic cells in tissues can immediately recognize and phagocytose pathogens. They use pattern recognition receptors (PRRs), like Toll-like receptors (TLRs), to recognize pathogen-associated molecular patterns (PAMPs) on pathogens. - **Goal**: To quickly contain and neutralize pathogens, preventing their spread and reducing the likelihood of infection. **2. Induced Innate Immunity** - **Timeline**: 4 hours to 4 days after infection. - **Primary Components**: Recruitment of additional innate immune cells, activation of cytokines and chemokines, inflammation, and enhanced phagocytosis. - **Steps**: 1. **Recognition and Signaling**: When initial containment fails, PRRs on resident macrophages and dendritic cells recognize PAMPs and activate signaling pathways. This activation leads to the production and release of cytokines (e.g., TNF-α, IL-1, IL-6) and chemokines. 2. **Inflammation and Recruitment**: Cytokines and chemokines promote inflammation, increasing blood flow and permeability in infected tissues. This attracts neutrophils, monocytes (which differentiate into macrophages), and other immune cells to the infection site. 3. **Phagocytosis and Killing**: Recruited phagocytes (like neutrophils and macrophages) engulf and destroy pathogens. NK cells are also activated to kill infected or stressed cells lacking MHC class I, which often indicates viral infection. 4. **Complement Activation**: The complement system is further activated, enhancing opsonization (marking pathogens for phagocytosis), promoting inflammation, and directly lysing pathogens. - **Goal**: To limit pathogen spread and create an environment to support the development of the adaptive immune response. **3. Adaptive Immunity** - **Timeline**: Begins around 4 days after infection and can last for weeks. - **Primary Components**: Antigen-specific T and B lymphocytes, antibodies, memory cell formation. - **Steps**: 1. **Antigen Presentation and T Cell Activation**: Dendritic cells, which have ingested pathogens or pathogen fragments, migrate to lymph nodes, where they present antigens on MHC molecules to naïve T cells. CD4+ helper T cells recognize antigens presented on MHC class II, while CD8+ cytotoxic T cells recognize antigens on MHC class I. 2. **Clonal Expansion and Differentiation**: Activated T cells undergo clonal expansion and differentiate into effector cells. CD4+ helper T cells further differentiate into subsets (e.g., Th1, Th2, Th17) that support various immune functions. CD8+ T cells become cytotoxic T lymphocytes (CTLs), capable of killing infected cells. 3. **B Cell Activation and Antibody Production**: Activated CD4+ helper T cells stimulate B cells, which, upon recognizing specific antigens, differentiate into plasma cells that produce antibodies. These antibodies neutralize pathogens, promote opsonization, and enhance phagocytosis. 4. **Memory Cell Formation**: Some T and B cells become memory cells, which persist long-term and respond more quickly and robustly if the same pathogen is encountered again. - **Goal**: To provide a highly specific, targeted response that eliminates the pathogen and establishes immunological memory, protecting the body against future infections by the same pathogen. **Summary of Immune Response Phases** **Phase** **Timeline** **Primary Components** **Goals** ------------------- ----------------- ------------------------------------------------------ ----------------------------------------- Immediate Innate 0--4 hours Barriers, resident macrophages, NK cells, complement Rapid containment of infection Induced Innate 4 hours--4 days Recruited phagocytes, cytokines, inflammation Limit spread, support adaptive response Adaptive Immunity 4 days--weeks T and B lymphocytes, antibodies, memory cells Specific pathogen elimination, memory This sequence ensures that pathogens are met with immediate containment, robust innate responses, and finally, a precise and lasting adaptive immune response, allowing the body to efficiently combat and remember pathogens. Know the functions of these cytokines: IFN-α, TNF, IL-6, IL-1β, and CXCL8 (IL-8) Cytokines are signaling molecules that play crucial roles in coordinating immune responses, especially during infection and inflammation. Here are the functions of **IFN-α**, **TNF**, **IL-6**, **IL-1β**, and **CXCL8 (IL-8)**: **1. IFN-α (Interferon-alpha)** - **Function**: IFN-α is part of the type I interferon family and plays a vital role in antiviral defense. - **Effects**: - Induces an antiviral state in neighboring cells by activating genes that inhibit viral replication. - Enhances the activity of NK cells, increasing their ability to kill virus-infected cells. - Increases the expression of MHC class I molecules, improving the presentation of viral antigens to CD8+ cytotoxic T cells. - **Role in Immune Response**: IFN-α is rapidly produced by virus-infected cells and plasmacytoid dendritic cells, helping to control viral infections early on. **2. TNF (Tumor Necrosis Factor)** - **Function**: TNF is a pro-inflammatory cytokine involved in inflammation and immune regulation. - **Effects**: - Promotes inflammation by increasing vascular permeability, allowing immune cells and proteins to enter infected or injured tissues. - Activates endothelial cells to express adhesion molecules, which helps recruit immune cells (e.g., neutrophils and monocytes) to the site of infection or injury. - Can induce fever and apoptosis in certain cells, contributing to the destruction of infected cells and limiting pathogen spread. - **Role in Immune Response**: TNF is critical for acute inflammatory responses and is released by macrophages, T cells, and other immune cells in response to infection or injury. **3. IL-6 (Interleukin-6)** - **Function**: IL-6 is a multifunctional cytokine that plays roles in inflammation, immune regulation, and hematopoiesis. - **Effects**: - Promotes the production of acute-phase proteins by the liver, which help fight infections (e.g., C-reactive protein). - Stimulates the differentiation of B cells into antibody-producing plasma cells. -