Coulson & Richardson Vol. 2 Particle Technology and Separation Processes PDF
Document Details
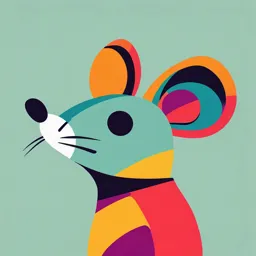
Uploaded by CoherentNeumann
Ladoke Akintola University of Technology Ogbomoso
J. F. Richardson, J. H. Harker, and J. R. Backhurst
Tags
Summary
This book, "Coulson & Richardson Vol. 2", provides a detailed exploration of particle technology and separation processes within chemical engineering. It covers various topics, including particulate solids, particle size reduction and enlargement, fluid flow through granular beds, sedimentation, and more. The book is intended as a textbook for students in chemical engineering.
Full Transcript
Coulson and Richardson’s CHEMICAL ENGINEERING VOLUME 2 FIFTH EDITION Particle Technology and Separation Processes Related Butterworth-Heinemann Titles in the Chemical Engineering Series by J. M. COULSON & J. F. RICHARDSON Chemical Engineering, Volume 1, Sixth e...
Coulson and Richardson’s CHEMICAL ENGINEERING VOLUME 2 FIFTH EDITION Particle Technology and Separation Processes Related Butterworth-Heinemann Titles in the Chemical Engineering Series by J. M. COULSON & J. F. RICHARDSON Chemical Engineering, Volume 1, Sixth edition Fluid Flow, Heat Transfer and Mass Transfer (with J. R. Backhurst and J. H. Harker) Chemical Engineering, Volume 3, Third edition Chemical and Biochemical Reaction Engineering, and Control (edited by J. F. Richardson and D. G. Peacock) Chemical Engineering, Volume 6, Third edition Chemical Engineering Design (R. K. Sinnott) Chemical Engineering, Solutions to Problems in Volume 1 (J. R. Backhurst, J. H. Harker and J. F. Richardson) Chemical Engineering, Solutions to Problems in Volume 2 (J. R. Backhurst, J. H. Harker and J. F. Richardson) Coulson and Richardson’s CHEMICAL ENGINEERING VOLUME 2 FIFTH EDITION Particle Technology and Separation Processes J. F. RICHARDSON University of Wales Swansea and J. H. HARKER University of Newcastle upon Tyne with J. R. BACKHURST University of Newcastle upon Tyne OXFORD AMSTERDAM BOSTON LONDON NEW YORK PARIS SAN DIEGO SAN FRANCISCO SINGAPORE SYDNEY TOKYO Butterworth-Heinemann An imprint of Elsevier Science Linacre House, Jordan Hill, Oxford OX2 8DP 225 Wildwood Avenue, Woburn, MA 01801-2041 First published 1955 Reprinted (with revisions) 1956, 1959, 1960 Reprinted 1962 Second edition 1968 Reprinted 1976 Third edition (SI units) 1978 Reprinted (with revisions) 1980, 1983, 1985, 1987, 1989 Fourth edition 1991 Reprinted (with revisions) 1993, 1996, 1997, 1998, 1999, 2001 Fifth edition 2002 Copyright 1991, 2002, J. F. Richardson and J. H. Harker. All rights reserved The right of J. F. Richardson and J. H. Harker to be identified as the authors of this work has been asserted in accordance with the Copyright, Designs and Patents Act 1988 All rights reserved. No part of this publication may be reproduced in any material form (including photocopying or storing in any medium by electronic means and whether or not transiently or incidentally to some other use of this publication) without the written permission of the copyright holder except in accordance with the provisions of the Copyright, Designs and Patents Act 1988 or under the terms of a licence issued by the Copyright Licensing Agency Ltd, 90 Tottenham Court Road, London, England W1P 0LP. Applications for the copyright holder’s written permission to reproduce any part of this publication should be addressed to the publishers British Library Cataloguing in Publication Data A catalogue record for this book is available from the British Library Library of Congress Cataloguing in Publication Data A catalogue record for this book is available from the Library of Congress ISBN 0 7506 4445 1 Typeset by Laserwords Private Limited, Chennai, India Printed and bounded in Great Britain by the Bath Press, Bath Preface to the Fifth Edition....................................... xvii Preface to the Fourth Edition.................................... xix Preface to the 1983 Reprint of the Third Edition..... xxi Preface to Third Edition............................................. xxiii Preface to Second Edition......................................... xxv Preface to First Edition.............................................. xxvii Acknowledgements................................................... xxix INTRODUCTION.......................................................... xxxi 1 Particulate Solids.................................................... 1 1.1. INTRODUCTION..................................................... 1 1.2. PARTICLE CHARACTERISATION......................... 2 1.3. PARTICULATE SOLIDS IN BULK.......................... 22 1.4. BLENDING OF SOLID PARTICLES....................... 30 1.5. CLASSIFICATION OF SOLID PARTICLES............ 37 1.6. SEPARATION OF SUSPENDED SOLID PARTICLES FROM FLUIDS.......................................... 67 1.7. FURTHER READING.............................................. 91 1.8. REFERENCES........................................................ 92 1.9. NOMENCLATURE.................................................. 93 2 Particle Size Reduction and Enlargement............ 95 2.1. INTRODUCTION..................................................... 95 2.2. SIZE REDUCTION OF SOLIDS.............................. 95 2.3. TYPES OF CRUSHING EQUIPMENT.................... 106 2.4. SIZE ENLARGEMENT OF PARTICLES................. 137 2.5. FURTHER READING.............................................. 143 2.6. REFERENCES........................................................ 143 2.7. NOMENCLATURE.................................................. 144 3 Motion of Particles in a Fluid................................. 146 3.1. INTRODUCTION..................................................... 146 3.2. FLOW PAST A CYLINDER AND A SPHERE......... 146 3.3. THE DRAG FORCE ON A SPHERICAL PARTICLE...................................................................... 149 3.4. NON-SPHERICAL PARTICLES.............................. 164 3.5. MOTION OF BUBBLES AND DROPS.................... 168 3.6. DRAG FORCES AND SETTLING VELOCITIES FOR PARTICLES IN NON- NEWTONIAN FLUIDS....... 169 3.7. ACCELERATING MOTION OF A PARTICLE IN THE GRAVITATIONAL FIELD....................................... 173 3.8. MOTION OF PARTICLES IN A CENTRIFUGAL FIELD............................................................................. 185 3.9. FURTHER READING.............................................. 187 3.10. REFERENCES...................................................... 188 3.11. NOMENCLATURE................................................ 189 4 Flow of Fluids through Granular Beds and Packed Columns........................................................ 191 4.1. INTRODUCTION..................................................... 191 4.2. FLOW OF A SINGLE FLUID THROUGH A GRANULAR BED........................................................... 191 4.3. DISPERSION.......................................................... 205 4.4. HEAT TRANSFER IN PACKED BEDS................... 211 4.5. PACKED COLUMNS.............................................. 212 4.6. FURTHER READING.............................................. 232 4.7. REFERENCES........................................................ 232 4.8. NOMENCLATURE.................................................. 234 5 Sedimentation......................................................... 237 5.1. INTRODUCTION..................................................... 237 5.2. SEDIMENTATION OF FINE PARTICLES.............. 237 5.3. SEDIMENTATION OF COARSE PARTICLES....... 267 5.4. FURTHER READING.............................................. 286 5.5. REFERENCES........................................................ 286 5.6. NOMENCLATURE.................................................. 288 6 Fluidisation.............................................................. 291 6.1. CHARACTERISTICS OF FLUIDISED SYSTEMS...................................................................... 291 6.2. LIQUID-SOLIDS SYSTEMS.................................... 302 6.3. GAS-SOLIDS SYSTEMS........................................ 315 6.4. GAS-LIQUID SOLIDS FLUIDISED BEDS............... 333 6.5. HEAT TRANSFER TO A BOUNDARY SURFACE...................................................................... 334 6.6. MASS AND HEAT TRANSFER BETWEEN FLUID AND PARTICLES............................................... 343 6.7. SUMMARY OF THE PROPERTIES OF FLUIDISED BEDS.......................................................... 357 6.8. APPLICATIONS OF THE FLUIDISED SOLIDS TECHNIQUE.................................................................. 358 6.9. FURTHER READING.............................................. 364 6.10. REFERENCES...................................................... 364 6.11. NOMENCLATURE................................................ 369 7 Liquid Filtration....................................................... 372 7.1. INTRODUCTION..................................................... 372 7.2. FILTRATION THEORY........................................... 374 7.3. FILTRATION PRACTICE........................................ 382 7.4. FILTRATION EQUIPMENT..................................... 387 7.5. FURTHER READING.............................................. 434 7.6. REFERENCES........................................................ 435 7.7. NOMENCLATURE.................................................. 435 8 Membrane Separation Processes.......................... 437 8.1. INTRODUCTION..................................................... 437 8.2. CLASSIFICATION OF MEMBRANE PROCESSES................................................................. 437 8.3. THE NATURE OF SYNTHETIC MEMBRANES...... 438 8.4. GENERAL MEMBRANE EQUATION..................... 442 8.5. CROSS-FLOW MICROFILTRATION...................... 442 8.6. ULTRAFILTRATION............................................... 446 8.7. REVERSE OSMOSIS............................................. 452 8.8. MEMBRANE MODULES AND PLANT CONFIGURATION......................................................... 455 8.9. MEMBRANE FOULING.......................................... 464 8.10. ELECTRODIALYSIS............................................. 465 8.11. REVERSE OSMOSIS WATER TREATMENT PLANT............................................................................ 467 8.12. PERVAPORATION............................................... 469 8.13. LIQUID MEMBRANES.......................................... 471 8.14. GAS SEPARATIONS............................................ 472 8.15. FURTHER READING............................................ 472 8.16. REFERENCES...................................................... 473 8.17. NOMENCLATURE................................................ 474 9 Centrifugal Separations.......................................... 475 9.1. INTRODUCTION..................................................... 475 9.2. SHAPE OF THE FREE SURFACE OF THE LIQUID........................................................................... 476 9.3. CENTRIFUGAL PRESSURE.................................. 477 9.4. SEPARATION OF IMMISCIBLE LIQUIDS OF DIFFERENT DENSITIES............................................... 478 9.5. SEDIMENTATION IN A CENTRIFUGAL FIELD..... 480 9.6. FILTRATION IN A CENTRIFUGE........................... 485 9.7. MECHANICAL DESIGN.......................................... 489 9.8. CENTRIFUGAL EQUIPMENT................................ 489 9.9. FURTHER READING.............................................. 500 9.10. REFERENCES...................................................... 500 9.11. NOMENCLATURE................................................ 501 10 Leaching................................................................ 502 10.1. INTRODUCTION................................................... 502 10.2. MASS TRANSFER IN LEACHING OPERATIONS................................................................ 503 10.3. EQUIPMENT FOR LEACHING............................. 506 10.4. COUNTERCURRENT WASHING OF SOLIDS.... 515 10.5. CALCULATION OF THE NUMBER OF STAGES......................................................................... 519 10.6. NUMBER OF STAGES FOR COUNTERCURRENT WASHING BY GRAPHICAL METHODS..................................................................... 526 10.7. FURTHER READING............................................ 540 10.8. REFERENCES...................................................... 540 10.9. NOMENCLATURE................................................ 540 11 Distillation.............................................................. 542 11.1. INTRODUCTION................................................... 542 11.2. VAPOUR LIQUID EQUILIBRIUM......................... 542 11.3. METHODS OF DISTILLATION TWO COMPONENT MIXTURES............................................ 555 11.4. THE FRACTIONATING COLUMN........................ 559 11.5. CONDITIONS FOR VARYING OVERFLOW IN NON-IDEAL BINARY SYSTEMS................................... 581 11.6. BATCH DISTILLATION......................................... 592 11.7. MULTICOMPONENT MIXTURES........................ 599 11.8. AZEOTROPIC AND EXTRACTIVE DISTILLATION............................................................... 616 11.9. STEAM DISTILLATION......................................... 621 11.10. PLATE COLUMNS.............................................. 625 11.11. PACKED COLUMNS FOR DISTILLATION......... 638 11.12. FURTHER READING.......................................... 649 11.13. REFERENCES.................................................... 649 11.14. NOMENCLATURE.............................................. 652 12 Absorption of Gases............................................. 656 12.1. INTRODUCTION................................................... 656 12.2. CONDITIONS OF EQUILIBRIUM BETWEEN LIQUID AND GAS.......................................................... 657 12.3. THE MECHANISM OF ABSORPTION................. 658 12.4. DETERMINATION OF TRANSFER COEFFICIENTS............................................................. 666 12.5. ABSORPTION ASSOCIATED WITH CHEMICAL REACTION................................................. 675 12.6. ABSORPTION ACCOMPANIED BY THE LIBERATION OF HEAT................................................. 681 12.7. PACKED TOWERS FOR GAS ABSORPTION..... 682 12.8. PLATE TOWERS FOR GAS ABSORPTION........ 702 12.9. OTHER EQUIPMENT FOR GAS ABSORPTION................................................................ 709 12.10. FURTHER READING.......................................... 714 12.11. REFERENCES.................................................... 715 12.12. NOMENCLATURE.............................................. 717 13 Liquid Liquid Extraction...................................... 721 13.1. INTRODUCTION................................................... 721 13.2. EXTRACTION PROCESSES................................ 722 13.3. EQUILIBRIUM DATA............................................ 725 13.4. CALCULATION OF THE NUMBER OF THEORETICAL STAGES.............................................. 728 13.5. CLASSIFICATION OF EXTRACTION EQUIPMENT.................................................................. 742 13.6. STAGE-WISE EQUIPMENT FOR EXTRACTION................................................................ 744 13.7. DIFFERENTIAL CONTACT EQUIPMENT FOR EXTRACTION................................................................ 750 13.8. USE OF SPECIALISED FLUIDS.......................... 763 13.9. FURTHER READING............................................ 766 13.10. REFERENCES.................................................... 767 13.11. NOMENCLATURE.............................................. 769 14 Evaporation........................................................... 771 14.1. INTRODUCTION................................................... 771 14.2. HEAT TRANSFER IN EVAPORATORS............... 771 14.3. SINGLE-EFFECT EVAPORATORS..................... 778 14.4. MULTIPLE-EFFECT EVAPORATORS................. 780 14.5. IMPROVED EFFICIENCY IN EVAPORATION..... 791 14.6. EVAPORATOR OPERATION............................... 802 14.7. EQUIPMENT FOR EVAPORATION..................... 805 14.8. FURTHER READING............................................ 823 14.9. REFERENCES...................................................... 823 14.10. NOMENCLATURE.............................................. 825 15 Crystallisation....................................................... 827 15.1. INTRODUCTION................................................... 827 15.2. CRYSTALLISATION FUNDAMENTALS............... 828 15.3. CRYSTALLISATION FROM SOLUTIONS............ 853 15.4. CRYSTALLISATION FROM MELTS..................... 868 15.5. CRYSTALLISATION FROM VAPOURS............... 875 15.6. FRACTIONAL CRYSTALLISATION..................... 885 15.7. FREEZE CRYSTALLISATION.............................. 888 15.8. HIGH PRESSURE CRYSTALLISATION.............. 890 15.9. FURTHER READING............................................ 893 15.10. REFERENCES.................................................... 894 15.11. NOMENCLATURE.............................................. 897 16 Drying..................................................................... 901 16.1. INTRODUCTION................................................... 901 16.2. GENERAL PRINCIPLES....................................... 901 16.3. RATE OF DRYING................................................ 904 16.4. THE MECHANISM OF MOISTURE MOVEMENT DURING DRYING.................................... 912 16.5. DRYING EQUIPMENT.......................................... 918 16.6. SPECIALISED DRYING METHODS..................... 957 16.7. THE DRYING OF GASES..................................... 963 16.8. FURTHER READING............................................ 964 16.9. REFERENCES...................................................... 965 16.10. NOMENCLATURE.............................................. 967 17 Adsorption............................................................. 970 17.1. INTRODUCTION................................................... 970 17.2. THE NATURE OF ADSORBENTS....................... 974 17.3. ADSORPTION EQUILIBRIA................................. 979 17.4. MULTICOMPONENT ADSORPTION................... 993 17.5. ADSORPTION FROM LIQUIDS........................... 994 17.6. STRUCTURE OF ADSORBENTS........................ 994 17.7. KINETIC EFFECTS............................................... 1002 17.8. ADSORPTION EQUIPMENT................................ 1008 17.9. REGENERATION OF SPENT ADSORBENT....... 1026 17.10. FURTHER READING.......................................... 1047 17.11. REFERENCES.................................................... 1047 17.12. NOMENCLATURE.............................................. 1049 18 Ion Exchange......................................................... 1053 18.1. INTRODUCTION................................................... 1053 18.2. ION EXCHANGE RESINS.................................... 1054 18.3. RESIN CAPACITY................................................ 1054 18.4. EQUILIBRIUM....................................................... 1056 18.5. EXCHANGE KINETICS........................................ 1060 18.6. ION EXCHANGE EQUIPMENT............................ 1066 18.7. FURTHER READING............................................ 1073 18.8. REFERENCES...................................................... 1073 18.9. NOMENCLATURE................................................ 1074 19 Chromatographic Separations............................. 1076 19.1. INTRODUCTION................................................... 1076 19.2. ELUTION CHROMATOGRAPHY......................... 1077 19.3. BAND BROADENING AND SEPARATION EFFICIENCY.................................................................. 1080 19.4. TYPES OF CHROMATOGRAPHY....................... 1083 19.5. LARGE SCALE ELUTION (CYCLIC BATCH) CHROMATOGRAPHY................................................... 1088 19.6. SELECTIVE ADSORPTION OF PROTEINS........ 1093 19.7. SIMULATED COUNTERCURRENT TECHNIQUES................................................................ 1096 19.8. COMBINED REACTION AND SEPARATION...... 1098 19.9. COMPARISON WITH OTHER SEPARATION METHODS..................................................................... 1099 19.10. FURTHER READING.......................................... 1100 19.11. REFERENCES.................................................... 1100 19.12. NOMENCLATURE.............................................. 1103 20 Product Design and Process Intensification...... 1104 20.1. PRODUCT DESIGN.............................................. 1104 20.2. PROCESS INTENSIFICATION............................. 1110 20.3. FURTHER READING............................................ 1134 20.4. REFERENCES...................................................... 1134 Appendix..................................................................... 1137 A1. STEAM TABLES...................................................... 1138 A2. CONVERSION FACTORS FOR SOME COMMON SI UNITS...................................................... 1147 Problems..................................................................... 1149 Preface to the Fifth Edition It is now 47 years since Volume 2 was first published in 1955, and during the inter- vening time the profession of chemical engineering has grown to maturity in the UK, and worldwide; the Institution of Chemical Engineers, for instance, has moved on from its 33rd to its 80th year of existence. No longer are the heavy chemical and petroleum-based indus- tries the main fields of industrial applications of the discipline, but chemical engineering has now penetrated into areas, such as pharmaceuticals, health care, foodstuffs, and biotechnology, where the general level of sophistication of the products is much greater, and the scale of production often much smaller, though the unit value of the products is generally much higher. This change has led to a move away from large-scale continuous plants to smaller-scale batch processing, often in multipurpose plants. Furthermore, there is an increased emphasis on product purity, and the need for more refined separation technology, especially in the pharmaceutical industry where it is often necessary to carry out the difficult separation of stereo-isomers, one of which may have the desired thera- peutic properties while the other is extremely malignant. Many of these large molecules are fragile and are liable to be broken down by the harsh solvents commonly used in the manufacture of bulk chemicals. The general principles involved in processing these more specialised materials are essentially the same as in bulk chemical manufacture, but special care must often be taken to ensure that processing conditions are mild. One big change over the years in the chemical and processing industries is the emphasis on designing products with properties that are specified, often in precise detail, by the customer. Chemical composition is often of relatively little importance provided that the product has the desired attributes. Hence product design, a multidisciplinary activity, has become a necessary precursor to process design. Although undergraduate courses now generally take into account these new require- ments, the basic principles of chemical engineering remain largely unchanged and this is particularly the case with the two main topics of Volume 2, Particle Mechanics and Separation Processes. In preparing this new edition, the authors have faced a typical engineering situation where a compromise has to be reached on size. The knowledge- base has increased to such an extent that many of the individual chapters appear to merit expansion into separate books. At the same time, as far as students and those from other disciplines are concerned, there is still a need for a an integrated concise treatment in which there is a consistency of approach across the board and, most importantly, a degree of uniformity in the use of symbols. It has to be remembered that the learning capacity of students is certainly no greater than it was in the past, and a book of manageable proportions is still needed. The advice that academic staffs worldwide have given in relation to revising the book has been that the layout should be retained substantially unchanged — better the devil we know, with all his faults! With this in mind the basic structure has been maintained. However, the old Chapter 8 on Gas Cleaning, which probably did not merit a chapter xvii xviii PREFACE TO THE FIFTH EDITION on its own, has been incorporated into Chapter 1, where it sits comfortably beside other topics involving the separation of solid particles from fluids. This has left Chapter 8 free to accommodate Membrane Separations (formerly Chapter 20) which then follows on logically from Filtration in Chapter 7. The new Chapter 20 then provides an opportunity to look to the future, and to introduce the topics of Product Design and the Use of Intensified Fields (particularly centrifugal in place of gravitational) and miniaturisation, with all the advantages of reduced hold-up, leading to a reduction in the amount of out-of-specification material produced during the changeover between products in the case multipurpose plants, and in improved safety where the materials have potentially hazardous properties. Other significant changes are the replacement of the existing chapter on Crystallisation by an entirely new chapter written with expert guidance from Professor J. W. Mullin, the author of the standard textbook on that topic. The other chapters have all been updated and additional Examples and Solutions incorporated in the text. Several additional Problems have been added at the end, and solutions are available in the Solutions Manual, and now on the Butterworth-Heinemann website. We are, as usual, indebted to both reviewers and readers for their suggestions and for pointing out errors in earlier editions. These have all been taken into account. Please keep it up in future! We aim to be error-free but are not always as successful as we would like to be! Unfortunately, the new edition is somewhat longer than the previous one, almost inevitably so with the great expansion in the amount of information available. Whenever in the past we have cut out material which we have regarded as being out-of-date, there is inevitably somebody who writes to say that he now has to keep both the old and the new editions because he finds that something which he had always found particularly useful in the past no longer appears in the revised edition. It seems that you cannot win, but we keep trying! J. F. RICHARDSON J. H. HARKER Preface to the Fourth Edition Details of the current restructuring of this Chemical Engineering Series, coinciding with the publication of the Fourth Edition of Volumes 1 and 2 and to be followed by new editions of the other volumes, have been set out in the Preface to the Fourth Edition of Volume 1. The revision involves the inclusion in Volume 1 of material on non-Newtonian flow (previously in Volume 3) and the transference from Volume 2 to Volume 1 of Pneumatic and Hydraulic Conveying and Liquid Mixing. In addition, Volume 6, written by Mr. R. K. Sinnott, which first appeared in 1983, nearly thirty years after the first volumes, acquires some of the design-orientated material from Volume 2, particularly that related to the hydraulics of packed and plate columns. The new sub-title of Volume 2, Particle Technology and Separation Processes, reflects both the emphasis of the new edition and the current importance of these two topics in Chemical Engineering. Particle Technology covers the basic properties of systems of particles and their preparation by comminution (Chapters 1 and 2). Subsequent chapters deal with the interaction between fluids and particles, under conditions ranging from those applicable to single isolated particles, to systems of particles freely suspended in fluids, as in sedimentation and fluidisation; and to packed beds and columns where particles are held in a fixed configuration relative to one another. The behaviour of particles in both gravitational and centrifugal fields is also covered. It will be noted that Centrifugal Separations are now brought together again in a single chapter, as in the original scheme of the first two editions, because the dispersal of the material between other chapters in the Third Edition was considered to be not entirely satisfactory. Fluid–solids Separation Processes are discussed in the earlier chapters under the headings of Sedimentation, Filtration, Gas Cleaning and Centrifugal Separations. The remaining separations involve applications of mass-transfer processes, in the presence of solid particles in Leaching (solid–liquid extraction), Drying and Crystallisation. In Distillation, Gas Absorption and Liquid–Liquid Extraction, interactions occur between two fluid streams with mass transfer taking place across a phase boundary. Usually these operations are carried out as continuous countercurrent flow processes, either stagewise (as in a plate-column) or with differential contacting (as in a packed-column). There is a case therefore for a generalised treatment of countercurrent contacting processes with each of the individual operations, such as Distillation, treated as particular cases. Although this approach has considerable merit, both conceptually and in terms of economy of space, it has not been adopted here, because the authors’ experience of teaching suggests that the student more readily grasps the principles involved, by considering each topic in turn, provided of course that the teacher makes a serious attempt to emphasise the common features. The new edition concludes with four chapters which are newcomers to Volume 2, each written by a specialist author from the Chemical Engineering Department at Swansea — xix xx PREFACE TO THE FOURTH EDITION Adsorption and Ion Exchange (Chapters 17 and 18) (topics previously covered in Volume 3) by J. H. Bowen Chromatographic Separations (Chapter 19) by J. R. Conder and Membrane Separations (Chapter 20) by W. R. Bowen. These techniques are of particular interest in that they provide a means of separating molecular species which are difficult to separate by other techniques and which may be present in very low concentrations. Such species include large molecules, sub-micrometre size particles, stereo-isomers and the products from bioreactors (Volume 3). The separa- tions can be highly specific and may depend on molecular size and shape, and the configuration of the constituent chemical groups of the molecules. Again I would express our deep sense of loss on the death of our colleague, Professor John Coulson, in January 1990. His two former colleagues at Newcastle, Dr. John Back- hurst and the Reverend Dr. John Harker, have played a substantial part in the preparation of this new edition both by updating the sections originally attributable to him, and by obtaining new illustrations and descriptions of industrial equipment. Finally, may I again thank our readers who, in the past, have made such helpful sugges- tions and have drawn to our attention errors, many of which would never have been spotted by the authors. Would they please continue their good work! Swansea J. F. RICHARDSON July 1990 Note to Fourth Edition — Revised Impression 1993 In this reprint corrections and minor revisions have been incorporated. The principal changes are as follows: (1) Addition of an account of the construction and operation of the Szego Grinding Mill (Chapter 2). (2) Inclusion of the Yoshioka method for the design of thickeners (Chapter 5). (3) Incorporation of Geldart’s classification of powders in relation to fluidisation charac- teristics (Chapter 6). (4) The substitution of a more logical approach to filtration of slurries yielding compressible cakes and redefinition of the specific resistance (Chapter 7). (5) Revision of the nomenclature for the underflow streams of washing thickeners to bring it into line with that used for other stagewise processes, including distillation and absorption (Chapter 10). (6) A small addition to the selection of dryers and the inclusion of Examples (Chapter 16). JFR Preface to the 1983 Reprint of the Third Edition In this volume, there is an account of the basic theory underlying the various Unit Opera- tions, and typical items of equipment are described. The equipment items are the essential components of a complete chemical plant, and the way in which such a plant is designed is the subject of Volume 6 of the series which has just appeared. The new volume includes material on flowsheeting, heat and material balances, piping, mechanical construction and costing. It completes the Series and forms an introduction to the very broad subject of Chemical Engineering Design. xxi Preface to Third Edition In producing a third edition, we have taken the opportunity, not only of updating the material but also of expressing the values of all the physical properties and characteristics of the systems in the SI System of units, as has already been done in Volumes 1 and 3. The SI system, which is described in detail in Volume 1, is widely adopted in Europe and is now gaining support elsewhere in the world. However, because some readers will still be more familiar with the British system, based on the foot, pound and second, the old units have been retained as alternatives wherever this can be done without causing confusion. The material has, to some extent, been re-arranged and the first chapter now relates to the characteristics of particles and their behaviour in bulk, the blending of solids, and classification according to size or composition of material. The following chapters describe the behaviour of particles moving in a fluid and the effects of both gravitational and centrifugal forces and of the interactions between neighbouring particles. The old chapter on centrifuges has now been eliminated and the material dispersed into the appro- priate parts of other chapters. Important applications which are considered include flow in granular beds and packed columns, fluidisation, transport of suspended particles, filtration and gas cleaning. An example of the updating which has been carried out is the addition of a short section on fluidised bed combustion, potentially the most important commercial application of the technique of fluidisation. In addition, we have included an entirely new section on flocculation, which has been prepared for us by Dr. D. J. A. Williams of University College, Swansea, to whom we are much indebted. Mass transfer operations play a dominant role in chemical processing and this is reflected in the continued attention given to the operations of solid–liquid extraction, distillation, gas absorption and liquid–liquid extraction. The last of these subjects, together with material on liquid–liquid mixing, is now dealt within a single chapter on liquid–liquid systems, the remainder of the material which appeared in the former chapter on mixing having been included earlier under the heading of solids blending. The volume concludes with chapters on evaporation, crystallisation and drying. Volumes 1, 2 and 3 form an integrated series with the fundamentals of fluid flow, heat transfer and mass transfer in the first volume, the physical operations of chemical engineering in this, the second volume, and in the third volume, the basis of chemical and biochemical reactor design, some of the physical operations which are now gaining in importance and the underlying theory of both process control and computation. The solutions to the problems listed in Volumes 1 and 2 are now available as Volumes 4 and 5 respectively. Furthermore, an additional volume in the series is in course of preparation and will provide an introduction to chemical engineering design and indicate how the principles enunciated in the earlier volumes can be translated into chemical plant. We welcome the collaboration of J. R. Backhurst and J. H. Harker as co-authors in the preparation of this edition, following their assistance in the editing of the latest edition of Volume 1 and their authorship of Volumes 4 and 5. We also look forward to the appearance of R. K. Sinnott’s volume on chemical engineering design. xxiii Preface to Second Edition This text deals with the physical operations used in the chemical and allied industries. These operations are conveniently designated “unit operations” to indicate that each single operation, such as filtration, is used in a wide range of industries, and frequently under varying conditions of temperature and pressure. Since the publication of the first edition in 1955 there has been a substantial increase in the relevant technical literature but the majority of developments have originated in research work in government and university laboratories rather than in industrial compa- nies. As a result, correlations based on laboratory data have not always been adequately confirmed on the industrial scale. However, the section on absorption towers contains data obtained on industrial equipment and most of the expressions used in the chapters on distillation and evaporation are based on results from industrial practice. In carrying out this revision we have made substantial alteration to Chapters 1, 5, 6, 7, 12, 13 and 15∗ and have taken the opportunity of presenting the volume paged separately from Volume 1. The revision has been possible only as the result of the kind co- operation and help of Professor J. D. Thornton (Chapter 12), Mr. J. Porter (Chapter 13), Mr. K. E. Peet (Chapter 10) and Dr. B. Waldie (Chapter 1), all of the University at Newcastle, and Dr. N. Dombrowski of the University of Leeds (Chapter 15). We want in particular to express our appreciation of the considerable amount of work carried out by Mr. D. G. Peacock of the School of Pharmacy, University of London. He has not only checked through the entire revision but has made numerous additions to many chapters and has overhauled the index. We should like to thank the companies who have kindly provided illustrations of their equipment and also the many readers of the previous edition who have made useful comments and helpful suggestions. Chemical engineering is no longer confined to purely physical processes and the unit operations, and a number of important new topics, including reactor design, automatic control of plants, biochemical engineering, and the use of computers for both process design and control of chemical plant will be covered in a forthcoming Volume 3 which is in course of preparation. Chemical engineering has grown in complexity and stature since the first edition of the text, and we hope that the new edition will prove of value to the new generation of university students as well as forming a helpful reference book for those working in industry. In presenting this new edition we wish to express our gratitude to Pergamon Press who have taken considerable trouble in coping with the technical details. J. M. COULSON J. F. RICHARDSON ∗ N.B. Chapter numbers are altered in the current (third) edition. xxv Preface to First Edition In presenting Volume 2 of Chemical Engineering, it has been our intention to cover what we believe to be the more important unit operations used in the chemical and process industries. These unit operations, which are mainly physical in nature, have been classified, as far as possible, according to the underlying mechanism of the transfer operation. In only a few cases is it possible to give design procedures when a chemical reaction takes place in addition to a physical process. This difficulty arises from the fact that, when we try to design such units as absorption towers in which there is a chemical reaction, we are not yet in a position to offer a thoroughly rigorous method of solution. We have not given an account of the transportation of materials in such equipment as belt conveyors or bucket elevators, which we feel lie more distinctly in the field of mechanical engineering. In presenting a good deal of information in this book, we have been much indebted to facilities made available to us by Professor Newitt, in whose department we have been working for many years. The reader will find a number of gaps, and a number of principles which are as yet not thoroughly developed. Chemical engineering is a field in which there is still much research to be done, and, if this work will in any way stimulate activities in this direction, we shall feel very much rewarded. It is hoped that the form of presentation will be found useful in indicating the kind of information which has been made available by research workers up to the present day. Chemical engineering is in its infancy, and we must not suppose that the approach presented here must necessarily be looked upon as correct in the years to come. One of the advantages of this subject is that its boundaries are not sharply defined. Finally, we should like to thank the following friends for valuable comments and suggestions: Mr. G. H. Anderson, Mr. R. W. Corben, Mr. W. J. De Coursey, Dr. M. Guter, Dr. L. L. Katan, Dr. R. Lessing, Dr. D. J. Rasbash, Dr. H. Sawistow- ski, Dr. W. Smith, Mr. D. Train, Mr. M. E. O’K. Trowbridge, Mr. F. E. Warner and Dr. W. N. Zaki. xxvii Acknowledgements The authors and publishers acknowledge with thanks the assistance given by the following companies and individuals in providing illustrations and data for this volume and giving their permission for reproduction. Everyone was most helpful and some firms went to considerable trouble to provide exactly what was required. We are extremely grateful to them all. Robinson Milling Systems Ltd for Fig. 1.16. Baker Perkins Ltd for Figs. 1.23, 2.8, 2.34, 13.39, 13.40. Buss (UK) Ltd, Cheadle Hulme, Cheshire for Figs. 1.24, 14.24. Dorr-Oliver Co Ltd, Croydon, Surrey for Figs. 1.26, 1.29, 7.15, 7.22, 10.8, 10.9. Denver Process Equipment Ltd, Leatherhead, Surrey for Figs. 1.27, 1.30, 1.32, 1.47, 1.48. Wilfley Mining Machinery Co Ltd for Fig. 1.33. NEI International Combustion Ltd, Derby for Figs. 1.34, 1.35, 1.36, 1.37, 2.10, 2.20–2.24, 2.27, 2.30, 14.18, 16.23. Lockers Engineers Ltd, Warrington for Figs. 1.40, 1.41, 1.42. Master Magnets Ltd, Birmingham for Figs. 1.43, 1.44, 1.45. AAF Ltd, Cramlington, Northumberland for Figs. 1.54, 1.68, 1.70, 1.72. Vaba Process Plant Ltd, Rotherham, Yorks, successors to Edgar Allen Co Ltd for Figs. 2.4, 2.7, 2.13, 2.14, 2.29. Hadfields Ltd for Fig. 2.5. Hosokawa Micron Ltd, Runcorn, Cheshire for Fig. 2.12. Babcock & Wilcox Ltd for Fig. 2.19. Premier Colloid Mills for Figs. 2.33. Amandus–Kahl, Hamburg, Germany for Fig. 2.35. McGraw-Hill Book Co for Fig. 3.3. Norton Chemical Process Products (Europe) Ltd, Stoke-on-Trent, Staffs for Table 4.3. Glitsch UK Ltd, Kirkby Stephen, Cheshire for Figs. 11.51, 11.52, 11.53. Sulzer (UK) Ltd, Farnborough, Hants for Figs. 1.67, 4.13, 4.14, 4.15. Johnson-Progress Ltd, Stoke-on-Trent, Staffs for Figs. 7.6, 7.7, 7.9, 7.10. Filtration Systems Ltd, Mirfield, W. Yorks, for Fig. 7.11. Stockdale Filtration Systems Ltd, Macclesfield, Cheshire for Fig. 7.12, and Tables 7.1, 7.2, 7.3. Stella-Meta Filters Ltd, Whitchurch, Hants for Figs. 7.13, 7.14. Delfilt Ltd, Bath, Avon for Fig. 7.16. Charlestown Engineering Ltd, St Austell, Cornwall for Figs. 7.24, 7.25. Institution of Mechanical Engineers for Fig. 1.59. Sturtevant Engineering Co Ltd for Fig. 1.60. Thomas Broadbent & Sons Ltd, Huddersfield, West Yorkshire for Figs. 9.13, 9.14. xxix xxx ACKNOWLEDGEMENTS Amicon Ltd for Figs. 8.12. P. C. I. for Fig. 8.9. A/S De Danske Sukkerfabrikker, Denmark for Fig. 8.10. Dr Huabin Yin for Fig. 8.1. Dr Nrdal Hilal for Fig. 8.2. Alfa-Laval Sharples Ltd, Camberley, Surrey, for Figs. 9.8, 9.10, 9.11, 9.12, 9.16, 9.17, 13.37, 14.20. Sulzer Escher Wyss Ltd, Zurich, Switzerland for Fig. 9.15. APV Mitchell Ltd for Fig. 12.17. Davy Powergas Ltd for Fig. 13.26. Swenson Evaporator Co for Figs. 14.17, 14.18, 14.19, 14.20. APV Baker Ltd, Crawley, Sussex for Figs. 14.21, 14.22, 14.23, 14.24, 14.25, 14.26. The Editor and Publishers of Chemical and Process Engineering for Figs. 14.28, 14.30. APV Pasilac Ltd, Carlisle, Cumbria for Figs. 16.10, 16.11. Buflovak Equipment Division of Blaw-Knox Co Ltd for Figs. 16.17, 16.18, and Table 16.3. Dr N. Dombrowski for Figs. 16.19, 16.22. Ventilex for Fig. 16.29. INTRODUCTION The understanding of the design and construction of chemical plant is frequently regarded as the essence of chemical engineering and it is this area which is covered in Volume 6 of this series. Starting from the original conception of the process by the chemist, it is necessary to appreciate the chemical, physical and many of the engineering features in order to develop the laboratory process to an industrial scale. This volume is concerned mainly with the physical nature of the processes that take place in industrial units, and, in particular, with determining the factors that influence the rate of transfer of material. The basic principles underlying these operations, namely fluid dynamics, and heat and mass transfer, are discussed in Volume 1, and it is the application of these principles that forms the main part of Volume 2. Throughout what are conveniently regarded as the process industries, there are many physical operations that are common to a number of the individual industries, and may be regarded as unit operations. Some of these operations involve particulate solids and many of them are aimed at achieving a separation of the components of a mixture. Thus, the separation of solids from a suspension by filtration, the separation of liquids by distillation, and the removal of water by evaporation and drying are typical of such operations. The problem of designing a distillation unit for the fermentation industry, the petroleum industry or the organic chemical industry is, in principle, the same, and it is mainly in the details of construction that the differences will occur. The concentration of solutions by evaporation is again a typical operation that is basically similar in the handling of sugar, or salt, or fruit juices, though there will be differences in the most suitable arrangement. This form of classification has been used here, but the operations involved have been grouped according to the mechanism of the transfer operation, so that the operations involving solids in fluids are considered together and then the diffusion processes of distillation, absorption and liquid-liquid extraction are taken in successive chapters. In examining many of these unit operations, it is found that the rate of heat transfer or the nature of the fluid flow is the governing feature. The transportation of a solid or a fluid stream between processing units is another instance of the importance of understanding fluid dynamics. One of the difficult problems of design is that of maintaining conditions of similarity between laboratory units and the larger-scale industrial plants. Thus, if a mixture is to be maintained at a certain temperature during the course of an exothermic reaction, then on the laboratory scale there is rarely any real difficulty in maintaining isothermal conditions. On the other hand, in a large reactor the ratio of the external surface to the volume — which is inversely proportional to the linear dimension of the unit — is in most cases of a different order, and the problem of removing the heat of reaction becomes a major item in design. Some of the general problems associated with scaling-up are considered as they arise in many of the chapters. Again, the introduction and removal of the reactants may present difficult problems on the large scale, especially if they contain corrosive liquids or abrasive xxxi xxxii INTRODUCTION solids. The general tendency with many industrial units is to provide a continuous process, frequently involving a series of stages. Thus, exothermic reactions may be carried out in a series of reactors with interstage cooling. The planning of a process plant will involve determining the most economic method, and later the most economic arrangement of the individual operations used in the process. This amounts to designing a process so as to provide the best combination of capital and operating costs. In this volume the question of costs has not been considered in any detail, but the aim has been to indicate the conditions under which various types of units will operate in the most economical manner. Without a thorough knowledge of the physical principles involved in the various operations, it is not possible to select the most suitable one for a given process. This aspect of the design can be considered by taking one or two simple illustrations of separation processes. The particles in a solid-solid system may be separated, first according to size, and secondly according to the material. Generally, sieving is the most satisfactory method of classifying relatively coarse materials according to size, but the method is impracticable for very fine particles and a form of settling process is generally used. In the first of these processes, the size of the particle is used directly as the basis for the separation, and the second depends on the variation with size of the behaviour of particles in a fluid. A mixed material can also be separated into its components by means of settling methods, because the shape and density of particles also affect their behaviour in a fluid. Other methods of separation depend on differences in surface properties (froth flotation), magnetic properties (magnetic separation), and on differences in solubility in a solvent (leaching). For the separation of miscible liquids, three commonly used methods are: 1. Distillation — depending on difference in volatility. 2. Liquid–liquid extraction — depending on difference in solubility in a liquid solvent. 3. Freezing — depending on difference in melting point. The problem of selecting the most appropriate operation will be further complicated by such factors as the concentration of liquid solution at which crystals start to form. Thus, in the separation of a mixture of ortho-, meta-, and para-mononitrotoluenes, the decision must be made as to whether it is better to carry out the separation by distillation followed by crystallisation, or in the reverse order. The same kind of consideration will arise when concentrating a solution of a solid; then it must be decided whether to stop the evaporation process when a certain concentration of solid has been reached and then to proceed with filtration followed by drying, or whether to continue to concentration by evaporation to such an extent that the filtration stage can be omitted before moving on to drying. In many operations, for instance in a distillation column, it is necessary to understand the fluid dynamics of the unit, as well as the heat and mass transfer relationships. These factors are frequently interdependent in a complex manner, and it is essential to consider the individual contributions of each of the mechanisms. Again, in a chemical reaction the final rate of the process may be governed either by a heat transfer process or by the chemical kinetics, and it is essential to decide which is the controlling factor; this problem is discussed in Volume 3, which deals with both chemical and biochemical reactions and their control. INTRODUCTION xxxiii Two factors of overriding importance have not so far been mentioned. Firstly, the plant must be operated in such a way that it does not present an unacceptable hazard to the workforce or to the surrounding population. Safety considerations must be in the forefront in the selection of the most appropriate process route and design, and must also be reflected in all the aspects of plant operation and maintenance. An inherently safe plant is to be preferred to one with inherent hazards, but designed to minimise the risk of the hazard being released. Safety considerations must be taken into account at an early stage of design; they are not an add-on at the end. Similarly control systems, the integrity of which play a major part in safe operation of plant, must be designed into the plant, not built on after the design is complete. The second consideration relates to the environment. The engineer has the responsi- bility for conserving natural resources, including raw materials and energy sources, and at the same time ensuring that effluents (solids, liquids and gases) do not give rise to unacceptable environmental effects. As with safety, effluent control must feature as a major factor in the design of every plant. The topics discussed in this volume form an important part of any chemical engineering project. They must not, however, be considered in isolation because, for example, a difficult separation problem may often be better solved by adjustment of conditions in the preceding reactor, rather than by the use of highly sophisticated separation techniques. CHAPTER 1 Particulate Solids 1.1. INTRODUCTION In Volume 1, the behaviour of fluids, both liquids and gases is considered, with particular reference to their flow properties and their heat and mass transfer characteristics. Once the composition, temperature and pressure of a fluid have been specified, then its relevant physical properties, such as density, viscosity, thermal conductivity and molecular diffu- sivity, are defined. In the early chapters of this volume consideration is given to the properties and behaviour of systems containing solid particles. Such systems are generally more complicated, not only because of the complex geometrical arrangements which are possible, but also because of the basic problem of defining completely the physical state of the material. The three most important characteristics of an individual particle are its composition, its size and its shape. Composition determines such properties as density and conductivity, provided that the particle is completely uniform. In many cases, however, the particle is porous or it may consist of a continuous matrix in which small particles of a second material are distributed. Particle size is important in that this affects properties such as the surface per unit volume and the rate at which a particle will settle in a fluid. A particle shape may be regular, such as spherical or cubic, or it may be irregular as, for example, with a piece of broken glass. Regular shapes are capable of precise definition by mathematical equations. Irregular shapes are not and the properties of irregular particles are usually expressed in terms of some particular characteristics of a regular shaped particle. Large quantities of particles are handled on the industrial scale, and it is frequently necessary to define the system as a whole. Thus, in place of particle size, it is necessary to know the distribution of particle sizes in the mixture and to be able to define a mean size which in some way represents the behaviour of the particulate mass as a whole. Important operations relating to systems of particles include storage in hoppers, flow through orifices and pipes, and metering of flows. It is frequently necessary to reduce the size of particles, or alternatively to form them into aggregates or sinters. Sometimes it may be necessary to mix two or more solids, and there may be a requirement to separate a mixture into its components or according to the sizes of the particles. In some cases the interaction between the particles and the surrounding fluid is of little significance, although at other times this can have a dominating effect on the behaviour of the system. Thus, in filtration or the flow of fluids through beds of granular particles, the characterisation of the porous mass as a whole is the principal feature, and the resistance to flow is dominated by the size and shape of the free space between the particles. In such situations, the particles are in physical contact with adjoining particles and there is 1 2 CHEMICAL ENGINEERING little relative movement between the particles. In processes such as the sedimentation of particles in a liquid, however, each particle is completely surrounded by fluid and is free to move relative to other particles. Only very simple cases are capable of a precise theoretical analysis and Stokes’ law, which gives the drag on an isolated spherical particle due to its motion relative to the surrounding fluid at very low velocities, is the most important theoretical relation in this area of study. Indeed very many empirical laws are based on the concept of defining correction factors to be applied to Stokes’ law. 1.2. PARTICLE CHARACTERISATION 1.2.1. Single particles The simplest shape of a particle is the sphere in that, because of its symmetry, any question of orientation does not have to be considered, since the particle looks exactly the same from whatever direction it is viewed and behaves in the same manner in a fluid, irrespective of its orientation. No other particle has this characteristic. Frequently, the size of a particle of irregular shape is defined in terms of the size of an equivalent sphere although the particle is represented by a sphere of different size according to the property selected. Some of the important sizes of equivalent spheres are: (a) The sphere of the same volume as the particle. (b) The sphere of the same surface area as the particle. (c) The sphere of the same surface area per unit volume as the particle. (d) The sphere of the same area as the particle when projected on to a plane perpen- dicular to its direction of motion. (e) The sphere of the same projected area as the particle, as viewed from above, when lying in its position of maximum stability such as on a microscope slide for example. (f) The sphere which will just pass through the same size of square aperture as the particle, such as on a screen for example. (g) The sphere with the same settling velocity as the particle in a specified fluid. Several definitions depend on the measurement of a particle in a particular orientation. Thus Feret’s statistical diameter is the mean distance apart of two parallel lines which are tangential to the particle in an arbitrarily fixed direction, irrespective of the orientation of each particle coming up for inspection. This is shown in Figure 1.1. A measure of particle shape which is frequently used is the sphericity, ψ, defined as: surface area of sphere of same volume as particle ψ= (1.1) surface area of particle Another method of indicating shape is to use the factor by which the cube of the size of the particle must be multiplied to give the volume. In this case the particle size is usually defined by method (e). Other properties of the particle which may be of importance are whether it is crystalline or amorphous, whether it is porous, and the properties of its surface, including roughness and presence of adsorbed films. PARTICULATE SOLIDS 3 Figure 1.1. Feret’s diameter Hardness may also be important if the particle is subjected to heavy loading. 1.2.2. Measurement of particle size Measurement of particle size and of particle size distribution is a highly specialised topic, and considerable skill is needed in the making of accurate measurements and in their interpretation. For details of the experimental techniques, reference should be made to a specialised text, and that of ALLEN(1) is highly recommended. No attempt is made to give a detailed account or critical assessment of the various methods of measuring particle size, which may be seen from Figure 1.2 to cover a range of 107 in linear dimension, or 1021 in volume! Only a brief account is given of some of the principal methods of measurement and, for further details, it is necessary to refer to one of the specialist texts on particle size measurement, the outstanding example of which is the two-volume monograph by ALLEN(1) , with HERDAN(2) providing additional information. It may be noted that both the size range in the sample and the particle shape may be as important, or even more so, than a single characteristic linear dimension which at best can represent only one single property of an individual particle or of an assembly of particles. The ability to make accurate and reliable measurements of particle size is acquired only after many years of practical experimental experience. For a comprehensive review of methods and experimental details it is recommended that the work of Allen be consulted and also Wardle’s work on Instrumentation and Control discussed in Volume 3. Before a size analysis can be carried out, it is necessary to collect a representative sample of the solids, and then to reduce this to the quantity which is required for the chosen method of analysis. Again, the work of Allen gives information on how this is best carried out. Samples will generally need to be taken from the bulk of the powder, whether this is in a static heap, in the form of an airborne dust, in a flowing or falling stream, or on a conveyor belt, and in each case the precautions which need to be taken to obtain a representative sample are different. A wide range of measuring techniques is available both for single particles and for systems of particles. In practice, each method is applicable to a finite range of sizes and gives a particular equivalent size, dependent on the nature of the method. The principles 4 CHEMICAL ENGINEERING 105 Pelleted products Particle size Crystalline industrial chemicals (µm) 104 Granular fertilisers, herbicides, fungicides Detergents 103 Granulated sugars Spray dried products 10 2 Powdered chemicals Powdered sugar Flour 1 10 Toners Powder metals Ceramics 100 Electronic materials Photographic emulsions Magnetic and other pigments 10−1 Organic pigments Fumed silica Metal catalysts 10−2 Carbon blacks Figure 1.2. Sizes of typical powder products(1) of some of the chief methods are now considered together with an indication of the size range to which they are applicable. Sieving (>50 µm) Sieve analysis may be carried out using a nest of sieves, each lower sieve being of smaller aperture size. Generally, sieve series are arranged so that the ratio of aperture sizes on consecutive sieves is 2, 21/2 or 21/4 according to the closeness of sizing that is required. The sieves may either be mounted on a vibrator, which should be designed to give a degree of vertical movement in addition to the horizontal vibration, or may be hand shaken. Whether or not a particle passes through an aperture depends not only upon its size, but also on the probability that it will be presented at the required orientation at the surface of the screen. The sizing is based purely on the linear dimensions of the particle and the lower limit of size which can be used is determined by two principal factors. The first is that the proportion of free space on the screen surface becomes very small as the size of the aperture is reduced. The second is that attractive forces between particles become larger at small particle sizes, and consequently particles tend to stick together and block the screen. Sieves are available in a number of standard series. There are several standard series of screen and the sizes of the openings are determined by the thickness of wire used. In the U.K., British Standard (B.S.)(3) screens are made in sizes PARTICULATE SOLIDS 5 from 300-mesh upwards, although these are too fragile for some work. The Institute of Mining and Metallurgy (I.M.M.)(4) screens are more robust, with the thickness of the wire approximately equal to the size of the apertures. The Tyler series, which is standard in the United States, is intermediate between the two British series. Details of the three series of screens(3) are given in Table 1.1, together with the American Society for Testing Materials (ASTM) series(5). Table 1.1. Standard sieve sizes British fine mesh (B.S.S. 410)(3) I.M.M.(4) U.S. Tyler(5) U.S. A.S.T.M.(5) Nominal Nominal Nominal Nominal Sieve aperture Sieve aperture Sieve aperture Sieve aperture no. no. no. no. in. µm in. µm in. µm in. µm 325 0.0017 43 325 0.0017 44 270 0.0021 53 270 0.0021 53 300 0.0021 53 250 0.0024 61 230 0.0024 61 240 0.0026 66 200 0.0025 63 200 0.0029 74 200 0.0029 74 200 0.0030 76 170 0.0034 88 170 0.0035 89 150 0.0033 84 170 0.0035 89 150 0.0041 104 150 0.0041 104 140 0.0041 104 120 0.0049 124 120 0.0042 107 115 0.0049 125 120 0.0049 125 100 0.0060 152 100 0.0050 127 100 0.0058 147 100 0.0059 150 90 0.0055 139 80 0.0069 175 80 0.0070 177 85 0.0070 178 80 0.0062 157 65 0.0082 208 70 0.0083 210 70 0.0071 180 60 0.0098 250 72 0.0083 211 60 0.0083 211 60 0.0097 246 50 0.0117 297 60 0.0099 251 45 0.0138 350 52 0.0116 295 50 0.0100 254 48 0.0116 295 40 0.0165 420 40 0.0125 347 42 0.0133 351 35 0.0197 500 44 0.0139 353 35 0.0164 417 30 0.0232 590 36 0.0166 422 30 0.0166 422 32 0.0195 495 30 0.0197 500 28 0.0232 589 25 0.0236 600 22 0.0275 699 20 0.0250 635 24 0.0276 701 25 0.0280 710 18 0.0336 853 16 0.0312 792 20 0.0328 833 20 0.0331 840 16 0.0395 1003 16 0.0390 991 18 0.0394 1000 14 0.0474 1204 12 0.0416 1056 14 0.0460 1168 16 0.0469 1190 12 0.0553 1405 10 0.0500 1270 12 0.0550 1397 10 0.0660 1676 8 0.0620 1574 10 0.0650 1651 14 0.0555 1410 8 0.0810 2057 9 0.0780 1981 12 0.0661 1680 7 0.0949 2411 8 0.0930 2362 10 0.0787 2000 6 0.1107 2812 5 0.1000 2540 7 0.1100 2794 8 0.0937 2380 5 0.1320 3353 6 0.1310 3327 5 0.1560 3962 7 0.1110 2839 4 0.1850 4699 6 0.1320 3360 5 0.1570 4000 4 0.1870 4760 The efficiency of screening is defined as the ratio of the mass of material which passes the screen to that which is capable of passing. This will differ according to the size of the material. It may be assumed that the rate of passage of particles of a given size through the screen is proportional to the number or mass of particles of that size on the screen at any 6 CHEMICAL ENGINEERING instant. Thus, if w is the mass of particles of a particular size on the screen at a time t, then: dw = −kw (1.2) dt where k is a constant for a given size and shape of particle and for a given screen. Thus, the mass of particles (w1 − w2 ) passing the screen in time t is given by: w2 ln = −kt w1 or: w2 = w1 e−kt (1.3) If the screen contains a large proportion of material just a little larger than the maximum size of particle which will pass, its capacity is considerably reduced. Screening is generally continued either for a predetermined time or until the rate of screening falls off to a certain fixed value. Screening may be carried out with either wet or dry material. In wet screening, material is washed evenly over the screen and clogging is prevented. In addition, small particles are washed off the surface of large ones. This has the obvious disadvantage, however, that it may be necessary to dry the material afterwards. With dry screening, the material is sometimes brushed lightly over the screen so as to form a thin even sheet. It is important that any agitation is not so vigorous that size reduction occurs, because screens are usually quite fragile and easily damaged by rough treatment. In general, the larger and the more abrasive the solids the more robust is the screen required. Microscopic analysis (1–100 µm) Microscopic examination permits measurement of the projected area of the particle and also enables an assessment to be made of its two-dimensional shape. In general, the third dimension cannot be determined except when using special stereomicroscopes. The apparent size of particle is compared with that of circles engraved on a graticule in the eyepiece as shown in Figure 1.3. Automatic methods of scanning have been developed. By using the electron microscope(7) , the lower limit of size can be reduced to about 0.001 µm. Figure 1.3. Particle profiles and comparison circles PARTICULATE SOLIDS 7 Sedimentation and elutriation methods (>1 µm) These methods depend on the fact that the terminal falling velocity of a particle in a fluid increases with size. Sedimentation methods are of two main types. In the first, the pipette method, samples are abstracted from the settling suspension at a fixed horizontal level at intervals of time. Each sample contains a representative sample of the suspension, with the exception of particles larger than a critical size, all of which will have settled below the level of the sampling point. The most commonly used equipment, the Andreason pipette, is described by ALLEN(1). In the second method, which involves the use of the sedimentation balance, particles settle on an immersed balance pan which is continuously weighed. The largest particles are deposited preferentially and consequently the rate of increase of weight falls off progressively as particles settle out. Sedimentation analyses must be carried out at concentrations which are sufficiently low for interactive effects between particles to be negligible so that their terminal falling velocities can be taken as equal to those of isolated particles. Careful temperature control (preferably to ±0.1 deg K) is necessary to suppress convection currents. The lower limit of particle size is set by the increasing importance of Brownian motion for progressively smaller particles. It is possible however, to replace gravitational forces by centrifugal forces and this reduces the lower size limit to about 0.05 µm. The elutriation method is really a reverse sedimentation process in which the particles are dispersed in an upward flowing stream of fluid. All particles with terminal falling velocities less than the upward velocity of the fluid will be carried away. A complete size analysis can be obtained by using successively higher fluid velocities. Figure 1.4 shows the standard elutriator (BS 893)(6) for particles with settling velocities between 7 and 70 mm/s. Permeability methods (>1 µm) These methods depend on the fact that at low flowrates the flow through a packed bed is directly proportional to the pressure difference, the proportionality constant being propor- tional to the square of the specific surface (surface : volume ratio) of the powder. From this method it is possible to obtain the diameter of the sphere with the same specific surface as the powder. The reliability of the method is dependent upon the care with which the sample of powder is packed. Further details are given in Chapter 4. Electronic particle counters A suspension of particles in an electrolyte is drawn through a small orifice on either side of which is positioned an electrode. A constant electrical current supply is connected to the electrodes and the electrolyte within the orifice constitutes the main resistive component of the circuit. As particles enter the orifice they displace an equivalent volume of electrolyte, thereby producing a change in the electrical resistance of the circuit, the magnitude of which is related to the displaced volume. The consequent voltage pulse across the electrodes is fed to a multi-channel analyser. The distribution of pulses arising from the passage of many thousands of particles is then processed to provide a particle (volume) size distribution. The main disadvantage of the method is that the suspension medium must be so highly conducting that its ionic strength may be such that surface active additives may be 8 CHEMICAL ENGINEERING Figure 1.4. Standard elutriator with 70-mm tube (all dimensions in mm)(6) required in order to maintain colloidal stability of fine particle suspensions as discussed in Section 5.2.2. The technique is suitable for the analysis of non-conducting particles and for conducting particles when electrical double layers confer a suitable degree of electrical insulation. This is also discussed in Section 5.2.2. By using orifices of various diameters, different particle size ranges may be examined and the resulting data may then be combined to provide size distributions extending over a large proportion of the sub-millimetre size range. The prior removal from the suspension of particles of sizes upwards of about 60 per cent of the orifice diameter helps to prevent problems associated with blocking of the orifice. The Coulter Counter and the Elzone Analyser work on this principle. PARTICULATE SOLIDS 9 Laser diffraction analysers These instruments(8) exploit the radial light scattering distribution functions of particles. A suspension of particles is held in, or more usually passed across, the path of a colli- mated beam of laser light, and the radially scattered light is collected by an array of photodetectors positioned perpendicular to the optical axis. The scattered light distri- bution is sampled and processed using appropriate scattering models to provide a particle size distribution. The method is applicable to the analysis of a range of different particles in a variety of media. Consequently, it is possible to examine aggregation phenomenas as discussed in Section 5.4, and to monitor particle size for on-line control of process streams. Instruments are available which provide particle size information over the range 0.1–600 µm. Light scattered from particles smaller than 1 µm is strongly influenced by their optical properties and care is required in data processing and interpretation. The scattering models employed in data processing invariably involve the assumption of particle sphericity. Size data obtained from the analysis of suspensions of asymmetrical particles using laser diffraction tend to be somewhat more ambiguous than those obtained by electronic particle counting, where the solid volumes of the particles are detected. X-ray or photo-sedimentometers Information on particle size may be obtained from the sedimentation of particles in dilute suspensions. The use of pipette techniques can be rather tedious and care is required to ensure that measurements are sufficiently precise. Instruments such as X-ray or photo- sedimentometers serve to automate this method in a non-intrusive manner. The attenuation of a narrow collimated beam of radiation passing horizontally through a sample of suspension is related to the mass of solid material in the path of the beam. This attenuation can be monitored at a fixed height in the suspension, or can be monitored as the beam is raised at a known rate. This latter procedure serves to reduce the time required to obtain sufficient data from which the particle size distribution may be calculated. This technique is limited to the analysis of particles whose settling behaviour follows Stokes’ law, as discussed in Section 3.3.4, and to conditions where any diffusive motion of particles is negligible. Sub-micron particle sizing Particles of a size of less than 2 µm are of particular interest in Process Engineering because of their large specific surface and colloidal properties, as discussed in Section 5.2. The diffusive velocities of such particles are significant in comparison with their settling velocities. Provided that the particles scatter light, dynamic light scattering techniques, such as photon correlation spectroscopy (PCS), may be used to provide information about particle diffusion. In the PCS technique, a quiescent particle suspension behaves as an array of mobile scattering centres over which the coherence of an incident laser light beam is preserved. The frequency of the light intensity fluctuations at a point outside the incident light path is related to the time taken for a particle to diffuse a distance equivalent to the wavelength of the incident light. The dynamic light signal at such a point is sampled and 10 CHEMICAL ENGINEERING correlated with itself at different time intervals using a digital correlator and associated computer software. The relationship of the (so-called) auto-correlation function to the time intervals is processed to provide estimates of an average particle size and variance (polydispersity index). Analysis of the signals at different scattering angles enables more detailed information to be obtained about the size distribution of this fine, and usually problematical, end of the size spectrum. The technique allows fine particles to be examined in a liquid environment so that estimates can be made of their effective hydrodynamic sizes. This is not possible using other techniques. Provided that fluid motion is uniform in the illuminated region of the suspension, then similar information may also be extracted by analysis of laser light scattering from particles undergoing electrophoretic motion, that is migratory motion in an electric field, superimposed on that motion. Instrumentation and data processing techniques for systems employing dynamic light scattering for the examination of fine particle motion are currently under development. 1.2.3. Particle size distribution Most particulate systems of practical interest consist of particles of a wide range of sizes and it is necessary to be able to give a quantitative indication of the mean size and of the spread of sizes. The results of a size analysis can most conveniently be represented by means of a cumulative mass fraction curve, in which the proportion of particles (x) smaller than a certain size (d) is plotted against that size (d). In most practical determinations of particle size, the size analysis will be obtained as a series of steps, each step representing the proportion of particles lying within a certain small range of size. From these results a cumulative size distribution can be built up and this can then be approximated by a smooth curve provided that the size intervals are sufficiently small. A typical curve for size distribution on a cumulative basis is shown in Figure 1.5. This curve rises from zero to unity over the range from the smallest to the largest particle size present. The distribution of particle sizes can be seen more readily by plotting a size frequency curve, such as that shown in Figure 1.6, in which the slope (dx/dd) of the cumulative 1 dx x dd 0 d Figure 1.5. Size distribution curve — cumulative basis PARTICULATE SOLIDS 11 dx dd d Figure 1.6. Size distribution curve — frequency basis curve (Figure 1.5) is plotted against particle size (d). The most frequently occurring size is then shown by the maximum of the curve. For naturally occurring materials the curve will generally have a single peak. For mixtures of particles, there may be as many peaks as components in the mixture. Again, if the particles are formed by crushing larger particles, the curve may have two peaks, one characteristic of the material and the other characteristic of the equipment. 1.2.4. Mean particle size The expression of the particle size of a powder in terms of a single linear dimension is often required. For coarse particles, BOND(9,10) has somewhat arbitrarily chosen the size of the opening through which 80 per cent of the material will pass. This size d80 is a useful rough comparative measure for the size of material which has been through a crusher. A mean size will describe only one particular characteristic of the powder and it is important to decide what that characteristic is before the mean is calculated. Thus, it may be desirable to define the size of particle such that its mass or its surface or its length is the mean value for all the particles in the system. In the following discussion it is assumed that each of the particles has the same shape. Considering unit mass of particles consisting of n1 particles of characteristic dimension d1 , constituting a mass fraction x1 , n2 particles of size d2 , and so on, then: x1 = n1 k1 d13 ρs (1.4) and: x1 = 1 = ρs k1 (n1 d13 ) (1.5) 1 x1 Thus: n1 = (1.6) ρs k1 d13 If the size distribution can be represented by a continuous function, then: dx = ρs k1 d 3 dn dx or: = ρ s k1 d 3 (1.7) dn 12 CHEMICAL ENGINEERING 1 and: dx = 1 = ρs k1 d 3 dn (1.8) 0 where ρs is the density of the particles, and k1 is a constant whose value depends on the shape of the particle. Mean sizes based on volume The mean abscissa in Figure 1.5 is defined as the volume mean diameter dv , or as the mass mean diameter, where: 1 d dx 1 dv = 0 1 = d dx. (1.9) 0 dx 0 Expressing this relation in finite difference form, then: (d1 x1 ) dv = = (x1 d1 ) (1.10) x1 which, in terms of particle numbers, rather than mass fractions gives: ρs k1 (n1 d14 ) (n1 d14 ) dv = 3 = (1.11) ρs k1 (n1 d1 ) (n1 d13 ) Another mean size based on volume is the mean volume diameter d v. If all the particles are of diameter d v , then the total volume of particles is the same as in the mixture. k1 d v n1 = (k1 n1 d13 ) 3 Thus: − 3 (n1 d13 ) or: dv= (1.12) n1 Substituting from equation 1.6 gives: − − 3 x 3 1 d v = 1 = (1.13) (x1 /d13 ) (x1 /d13 ) Mean sizes based on surface In Figure 1.5, if, instead of fraction of total mass, the surface in each fraction is plotted against size, then a similar curve is obtained although the mean abscissa ds is then the surface mean diameter. [(n1 d1 )S1 ] (n1 k2 d13 ) (n1 d13 ) Thus: ds = = = (1.14) (n1 S1 ) (n1 k2 d12 ) (n1 d12 ) PARTICULATE SOLIDS 13 where S1 = k2 d12 , and k2 is a constant whose value depends on particle shape. ds is also known as the Sauter mean diameter and is the diameter of the particle with the same specific surface as the powder. Substituting for n1 from equation 1.6 gives: x1 1 ds = = (1.15) x1 x1 d1 d1 The mean surface diameter is defined as the size of particle d s which is such that if all the particles are of this size, the total surface will be the same as in the mixture. k2 d s n1 = (k2 n1 d12 ) 2 Thus: − (n1 d12 ) or: ds= (1.16) n1 Substituting for n1 gives: − (x1 /d1 ) ds= (1.17) (x1 /d13 ) Mean dimensions based on length A length mean diameter may be defined as: x1 [(n1 d1 )d1 ] (n1 d12 ) d dl = = = 1 (1.18) (n1 d1 ) (n1 d1 ) x1 d12 A mean length diameter or arithmetic mean diameter may also be defined by: d l n1 = (n1 d1 ) x1 (n1 d1 ) d12 d l = = (1.19) n1 x1 d13 Example 1.1 The size analysis of a powdered material on a mass basis is represented by a straight line from 0 per cent mass at 1 µm particle size to 100 per cent mass at 101 µm particle size as shown in Figure 1.7. Calculate the surface mean diameter of the particles constituting the system. 14 CHEMICAL ENGINEERING Figure 1.7. Size analysis of powder Solution From equation 1.15, the surface mean diameter is given by: 1 ds = (x1 /d1 ) Since the size analysis is represented by the continuous curve: d = 100x + 1 1 then: ds = 1 dx 0 d 1 = 1 dx 0 100x + 1 = (100/ ln 101) = 21.7 µm Example 1.2 The equations giving the number distribution curve for a powdered material are dn/dd = d for the size range 0–10 µm and dn/dd = 100,000/d 4 for the size range 10–100 µm where d is in µm. Sketch the number, surface and mass distribution curves and calculate the surface mean diameter for the powder. Explain briefly how the data required for the construction of these curves may be obtained experimentally. Solution Note: The equations for the number distributions are valid only for d expressed in µm. For the range, d = 0 − 10 µm, dn/dd = d On integration: n = 0.5d 2 + c1 (i) PARTICULATE SOLIDS 15 where c1 is the constant of integration. For the range, d = 10 − 100 µm, dn/dd = 105 d −4 On integration: n = c2 − (0.33 × 1