Inorganic Chemistry Chapter 4 Lecture Notes PDF
Document Details
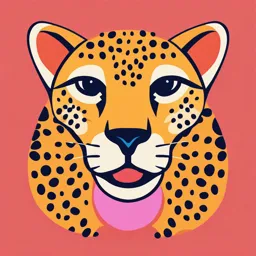
Uploaded by LoyalSteelDrums
Kent State University
Shriver/ Atkins
Tags
Related
- FALLSEM2024-25 BCHY101L TH VL2024250106792 Reference Material I PDF
- FALLSEM2024-25 BCHY101L TH VL2024250106792 Reference Material I PDF
- Module-2 Inorganic and Organometallic Chemistry PDF
- Metal-Metal Bonding in Organometallic Chemistry PDF
- Inorganic Chemistry II Practice Questions for Chapter 4 PDF
- Advanced Inorganic Chemistry Past Paper 2024 PDF
Summary
This document is a lecture about d-metal organometallic chemistry from a textbook. It covers topics on organometallic compounds, including their bonding, structures, and nomenclature. The document focuses on concepts central to inorganic textbooks used at the undergraduate level.
Full Transcript
Shriver/ Atkins: Inorganic Chemistry CHAPTER 4: d-Metal Organometallic Chemistry 1. Introduction ❖ Organometallic chemistry: the chemistry of compounds containing metal-carbon bonds. ❖ The organometallic chemistry of the s- and p-block metals was developed in the early...
Shriver/ Atkins: Inorganic Chemistry CHAPTER 4: d-Metal Organometallic Chemistry 1. Introduction ❖ Organometallic chemistry: the chemistry of compounds containing metal-carbon bonds. ❖ The organometallic chemistry of the s- and p-block metals was developed in the early part of the 20th centaury. ❖ The organometallic chemistry of the d- and f- blocks has been developed since the mid-1950s. ❖ The latter is now a thriving area that spans new types of reactions, unusual structures, and practical applications in organic synthesis and industrial catalysis. Inorganic Chemistry Chapter 1: Figure 20.1 Three early examples of d-metal organometallic compounds: Tetracarbonylnickel, One of the many anionic An ethene complex of synthesized by L. Mond, platinum(II), prepared metal carbonyls C. Langer, and F. Quinke by W.C. Zeise in 1827 synthesized by W. Hieber in 1890 in the 1930s © 2009 W.H. Freeman Shriver/ Atkins: Inorganic Chemistry 1. Introduction (cont’d) ❖ Based on the convention, compounds 1 – 3 clearly qualify as organometallic, but a complex such as [Co(en)3]3+, does not. ❖ However, metal cyanide complexes, e.g., hexacyanidoferrate(II) ions, do have M-C bonds, but are generally not considered as organometallic because their properties are more akin to coordination complexes. ❖ The justification for this somewhat arbitrary distinction is that many metal carbonyls are significantly different from coordination com[pounds. Shriver/ Atkins: Inorganic Chemistry 1. Introduction (cont’d) ❖ The distinctions between the two classes of compounds are clear: ❖ Coordination complexes normally are charged, with variable d-electron count, and are soluble in water; ❖ Organometallic compounds are often neutral, with fixed d-electron count, and are soluble in organic solvents such as tetrahydrofuran. Most organometallic compounds are more similar to organic compounds than inorganic salts, e.g., they have low melting points (some are liquid at room temperature). Shriver/ Atkins: Inorganic Chemistry 2. Bonding ❖ d-metal organometallic compounds have relatively few stable electron configurations. ❖ Two most common electron configurations have a total of 16 or 18 valence electrons around the metal atom. ❖ This restriction is due to the strength of the π (and d where appropriate) bonding interactions between the metal atom and the carbon-containing ligands. Shriver/ Atkins: Inorganic Chemistry 2.1 Stable electron configurations ❖ In the 1920s, N.V. Sidgwick recognized that Ni(CO)4 has the same valence electron count (18) as the noble gas that terminates the long period to which the metal belongs. ❖ He coined the term ‘inert gas rule’ for this indication of stability, but it is now usually referred to as the 18- electron rule. ❖ Compounds that have this configuration are remarkably stable, and the MO theory can satisfactorily explain the origin of such stability. Inorganic Chemistry Chapter 1: Figure 22.1 Hence, octahedral organometallic compounds are most stable when they have a total of 18 valence electrons around their central metal ion! © 2009 W.H. Freeman Inorganic Chemistry Chapter 1: Figure 20.1 © 2009 W.H. Freeman Inorganic Chemistry Chapter 1: Figure 20.2 © 2009 W.H. Freeman Shriver/ Atkins: Inorganic Chemistry 2.1 Stable electron configurations ❖ With strong-field ligands, a square-planar complex has only eight bonding MOs, thus a 16-electron configuration is the most energetically favorable configuration. ❖ Organometallic ligands often produce a strong field, many square-planar organometallic compounds exist. ❖ Stable square-planar complexes are normally found with a total of 16 valence electrons, resulting in filling all the bonding and none of the antibonding MOs. Inorganic Chemistry Chapter 1: Figure 22.2 © 2009 W.H. Freeman Inorganic Chemistry Chapter 1: Table 22.1 © 2009 W.H. Freeman Shriver/ Atkins: Inorganic Chemistry 2.2 Electron counting and oxidation states ❖ Two models are routinely used to count electrons, the so-called neutral-ligand method (sometimes called the covalent method) and the donor-pair method (sometimes known as the ionic method). ❖ In the neutral-ligand method, all ligands are treated as neutral and are categorized according to how many electrons they can donate. ❖ Ligands are defined as L type (two-electron donors) and X type (one-electron radical donors). Inorganic Chemistry Chapter 1: Table 22.2 © 2009 W.H. Freeman Shriver/ Atkins: Inorganic Chemistry 2.2 Electron counting and oxidation states ❖ The donor-pair method requires a calculation of the oxidation number. ❖ Neutral ligands, such as CO and phosphine, are considered to be two-electron donors and are formally assigned an oxidation number of 0. ❖ Ligands such as halides, H, and CH3 are treated as Cl- , H-, and CH3- with an oxidation number -1. ❖ The cyclopentadienyl ligand, C5H5 (Cp), is treated as C5H5- with an oxidation number of -1), and is considered to be a six-electron donor. Inorganic Chemistry Chapter 1: Table 22.2 © 2009 W.H. Freeman Shriver/ Atkins: Inorganic Chemistry 2.3 Nomenclature ❖ Ligands are listed in alphabetical order followed by the name of the metal, all written as one word. ❖ The name of the metal should be followed by its oxidation number in parentheses. ❖ In research journals, this has yet to become a common practice. ❖ For example, (11) is sometimes referred to as benzenemolybdenum-tricarbonyl, rather than the preferred name benzene(tricarbonyl)molybdenum(0). Inorganic Chemistry Chapter 1: Figure 22.3 © 2009 W.H. Freeman Shriver/ Atkins: Inorganic Chemistry 2.3 Nomenclature (cont’d) ❖ For the formula of an organometallic compound, the symbol for the metal is written first, followed by the ligands listed in alphabetical order based on their chemical symbol. ❖ Often a ligand with carbon donor atoms can exhibit multiple bonding modes, e.g. the cyclopentadienyl group can commonly bond to a d-metal atom in three different way ❖ This gives rise to the notion of hapticity, the number of ligand atoms bonded to the metal atom, ηn, where n is the number of atoms. Inorganic Chemistry Chapter 1: Figure 22.3 Three different bonding modes of Cp © 2009 W.H. Freeman Shriver/ Atkins: Inorganic Chemistry 2.4 Carbon monoxide as a ligand ❖ CO is a very common ligand in organometallic chemistry, where it is known as the carbonyl group. ❖ CO is particularly good at stabilizing very low oxidation states (+1, 0, -1). ❖ The lone pair on the carbon atom as a Lewis σ base (an electron-pair donor) and the empty CO antibonding orbital as a Lewis π acid (an electron-pair acceptor). Inorganic Chemistry Chapter 1: Figure 22.3 The bonding is made up of two parts: a σ bond from the ligand to the metal atom (15) and a π bond from the metal atom to the ligand (16). This type of π bonding is sometimes referred to as π backbonding. © 2009 W.H. Freeman Inorganic Chemistry Chapter 1: Figure 22.3 The MO diagram of CO © 2009 W.H. Freeman Shriver/ Atkins: Inorganic Chemistry 2.4 Carbon monoxide as a ligand (cont’d) ❖ The overall effect: the stronger the metal-carbon bond becomes, the weaker the CO bond becomes. ❖ In the extreme case, when two electrons are fully donated by the metal atom, a formal metal-carbon double bond is formed; because the two electrons occupy a CO antibonding orbital, this donation results in a decrease in the bond order of the CO to 2. ❖ In practice, the bonding is somewhere between M- CΞO, with no backbonding, and M=C=O, with complete backbonding. Inorganic Chemistry Chapter 1: Table 22.3 © 2009 W.H. Freeman Inorganic Chemistry Chapter 1: Figure 22.4 © 2009 W.H. Freeman Shriver/ Atkins: Inorganic Chemistry 2.5 Phosphines as ligands ❖ Phosphines bond to metals by a combination of σ donation from the P atom and π back-bonding from the metal atom. ❖ Like ammonia, phosphines can behave as a Lewis base and use its lone pair to donate electron density to a Lewis acid, and so act as a ligand. ❖ Phosphines also have empty orbitals on the P atom that can overlap with filled d orbitals on 3d-metal ions and behave as π acceptors Shriver/ Atkins: Inorganic Chemistry 2.5 Phosphines as ligands ❖ The σ-donating ability and π-acceptor ability of phosphines are inversely correlated. ❖ For example, electron-rich phosphines, such as PMe3, are good σ donors and poor π acceptors, whereas electron-poor phosphines, such as PF3, are poor σ donors and good π acceptors. ❖ Lewis basicity can normally be used as a single scale to indicate their donor/acceptor ability. The generally accepted order of basicity of phosphines is: Inorganic Chemistry Chapter 1: Table 22.4 © 2009 W.H. Freeman Shriver/ Atkins: Inorganic Chemistry 2.6 Hydride and dihydrogen as ligands ❖ A hydrogen atom directly bonded to a metal is commonly found in organometallic complexes and is referred to as a hydride ligand. ❖ The name ‘hydride’ can be misleading as it implies a H ligand. ❖ The formulation H- might be appropriate for most hydrides, such as [CoH(PMe3)4], but some hydrides are appreciably acidic and behave as though they contain H+, for example [CoH(CO)4] is an acid with pKa = 8.3 (in acetonitrile). Shriver/ Atkins: Inorganic Chemistry 2.6 Hydride and dihydrogen as ligands (cont’d) ❖ The M-H bond can be considered as a σ interaction between the two atoms. ❖ Hydrides are readily identified by NMR spectroscopy as their chemical shift is rather unusual, typically occurring in the range ❖ -50 < d < 0. ❖ Infrared spectroscopy can also be useful in identifying metal hydrides as they normally have a stretching band in the range 2850-2250 cm-1. ❖ X-ray diffraction is of little use in identifying hydrides because the low electron density of the H atom. Shriver/ Atkins: Inorganic Chemistry 2.6 Hydride and dihydrogen as ligands (cont’d) ❖ The bonding of dihydrogen to the metal atom is considered to be made up of two components: a σ donation of the two electrons in the H2 bond to the metal atom and a π backdonation from the metal to the σ* antibonding orbital of H2. ❖ This picture of the bonding raises a number of interesting issues. In particular, as the π backbonding from the metal atom increases, the strength of the H-H bond decreases and the structure tends to that of a dihydride: Inorganic Chemistry Chapter 1: Table 22.2 The π backdonation from the The σ donation of the two metal to the σ* antibonding electrons in the H2 bond orbital of H2 to the metal atom © 2009 W.H. Freeman Shriver/ Atkins: Inorganic Chemistry 2.6 Hydride and dihydrogen as ligands (cont’d) ❖ A dihydrogen molecule is treated as a neutral two- electron donor. ❖ Thus the transformation of the dihydrogen into two hydrides requires the formal charge on the metal atom to increase by two, i.e. the metal is oxidized by two units and the dihydrogen is reduced.. ❖ This transformation of the dihydrogen molecule to a dihydride is an example of oxidative addition. Shriver/ Atkins: Inorganic Chemistry 2.7 η1-Alkyl, -alkenyl, -alkynyl, and -aryl ligands ❖ They are best considered a simple covalent ’ interaction between the metal atom and the carbon atom of the organic fragment. ❖ Alkyl groups with a hydrogen atom on the adjacent C atom are prone to decompose by a process known as b-hydrogen elimination. ❖ They are commonly introduced into metal centers by the displacement of a halide with a lithium or Grignard reagent: Shriver/ Atkins: Inorganic Chemistry 2.7 η2-Alkene and -alkyne ligands ❖ They normally bond side-on to a metal atom with both carbon atoms of the double bond equidistant from the metal atom. ❖ Thus, the electron density of the C=C π bond can be donated to an empty orbital on the metal atom to form a σ bond, and thus considered to be two-electron neutral ligands. ❖ In parallel with this interaction, a filled metal d orbital can donate electron density back to the empty π* orbit- als of the alkene to form a π bond. Inorganic Chemistry Chapter 1: Figure 22.5 © 2009 W.H. Freeman Shriver/ Atkins: Inorganic Chemistry 2.8 Butadiene, cyclobutadiene, and cyclooctatetraene ❖ The two occupied lower energy MOs can behave as donors to the metal, the lowest a σ donor and the next a π donor. ❖ The next higher unoccupied MO, the LUMO, can act as a π acceptor from the metal atom. ❖ The resulting modification of electron density results in a shortening of the central C-C bond and a lengthening of the double C=C bonds. Inorganic Chemistry Chapter 1: Figure 22.6 © 2009 W.H. Freeman Shriver/ Atkins: Inorganic Chemistry 2.8 Butadiene, cyclobutadiene, and cyclooctatetraene ❖ Cyclobutadiene is rectangular (D2h) and unstable as a free molecule, as a result of bond angle constraints and its four-electron anti-aromatic configuration. ❖ However, stable complexes are known, including [Ru(η4-C4H4)(CO)3], in which coordination to a metal atom stabilizes an otherwise unstable molecule. ❖ If the ligand formally accepts two electrons from the metal atom, it will have six π electrons with three MOs occupied and no incentive to distort from square; two of the MOs are degenerate in this configuration. Inorganic Chemistry Chapter 1: Figure 22.7 © 2009 W.H. Freeman Inorganic Chemistry Chapter 1: Figure 22.7 © 2009 W.H. Freeman Shriver/ Atkins: Inorganic Chemistry 2.9 Benzene and other arenes ❖ In the molecular orbital picture of the π bonding in benzene there are three bonding and three antibonding orbitals. ❖ The strongest interaction is a σ interaction between the most strongly bonding a1 benzene MO and the dz2 orbital of the metal atom. ❖ π bonds are possible between the two other bonding benzene MOs and the dzx and dyz orbitals. ❖ Backbonding is possible as a δ interaction. Inorganic Chemistry Chapter 1: Figure 22.8 © 2009 W.H. Freeman Inorganic Chemistry Chapter 1: Figure 22.9 © 2009 W.H. Freeman Inorganic Chemistry Chapter 1: Figure 22.10 © 2009 W.H. Freeman Inorganic Chemistry Chapter 1: Table 22.5 © 2009 W.H. Freeman Inorganic Chemistry Chapter 1: Figure 22.11 © 2009 W.H. Freeman Inorganic Chemistry Chapter 1: Table 22.6 © 2009 W.H. Freeman Inorganic Chemistry Chapter 1: Figure 22.12 © 2009 W.H. Freeman Inorganic Chemistry Chapter 1: Table 22.7 © 2009 W.H. Freeman Inorganic Chemistry Chapter 1: Figure 22.13 © 2009 W.H. Freeman Inorganic Chemistry Chapter 1: Table 22.8 © 2009 W.H. Freeman Inorganic Chemistry Chapter 1: Figure 22.14 © 2009 W.H. Freeman Inorganic Chemistry Chapter 1: Figure 22.15 © 2009 W.H. Freeman Inorganic Chemistry Chapter 1: Table 22.9 © 2009 W.H. Freeman Inorganic Chemistry Chapter 1: Table 22.10 © 2009 W.H. Freeman Inorganic Chemistry Chapter 1: Figure 22.16 © 2009 W.H. Freeman Inorganic Chemistry Chapter 1: Table 22.11 © 2009 W.H. Freeman Inorganic Chemistry Chapter 1: Figure 22.17 © 2009 W.H. Freeman