Brock Biology of Microorganisms PDF
Document Details
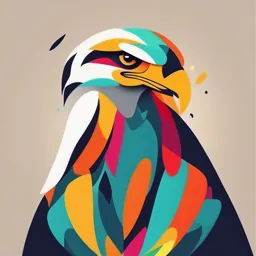
Uploaded by NimblePedalSteelGuitar1104
2021
Tags
Related
- Microbiology - Week 4 - Lecture 1 - Introduction to Microbial Diversity 2023 PDF
- Microbiology Lecture 2 - Microbial Growth and Nutrition 2023 PDF
- BIOL220 General Microbiology Course Overview PDF
- Microbiology - Week 4 - Lecture 1 - Introduction to Microbial Diversity 2023 PDF
- Microbiology Lecture 2 - Microbial Growth and Nutrition 2023 PDF
- Lecture 1 Microbiology & Microbial Growth PDF
Summary
This document is chapter 7 of the sixteenth edition of Brock Biology of Microorganisms, discussing microbial regulatory systems. It covers topics such as DNA-binding proteins, transcription factors, and the roles of activators and repressors.
Full Transcript
Brock Biology of Microorganisms Sixteenth Edition Chapter 7 Microbial Regulatory Systems Copyright © 2021, 2...
Brock Biology of Microorganisms Sixteenth Edition Chapter 7 Microbial Regulatory Systems Copyright © 2021, 2018, 2015 Pearson Education, Inc. All Rights Reserved I. DNA-Binding Proteins and One. Transcriptional Regulation 7.2 Transcription Factors and Effectors 7.3 Repression and Activation 7.4 Transcription Controls in Archaea Copyright © 2021, 2018, 2015 Pearson Education, Inc. All Rights Reserved 7.2 Transcription Factors and Effectors (1 of 2) More transcription leads to more mRNA for translation and more protein product Mechanisms of Transcription Factors – Transcription factors: Proteins that control the rate of transcription by binding to specific DNA ▪ Activator protein (Figure 7.4a) turns on transcription – Binds DNA and recruits RNA polymerase or sigma factor to promoter region ▪ Repressor protein turns off expression – Binds operator region of DNA downstream of promoter Copyright © 2021, 2018, 2015 Pearson Education, Inc. All Rights Reserved Figure 7.4 Transcription Factors, Effectors, and Allosterism Copyright © 2021, 2018, 2015 Pearson Education, Inc. All Rights Reserved 7.2 Transcription Factors and Effectors (2 of 2) Effectors: Small molecules that control binding of activators and repressors – Typically cell metabolites (e.g., substrates, products) or structural analogs Allosteric proteins: Conformation altered when effector molecule binds – Since transcription factors are allosteric, conformational change determines whether transcription factor can bind DNA – Inducers turn on transcription, corepressors turn off transcription Copyright © 2021, 2018, 2015 Pearson Education, Inc. All Rights Reserved 7.3 Repression and Activation (1 of 8) Enzyme Repression and Induction – Enzyme repression: preventing the synthesis of an enzyme unless product is absent from culture medium; excess of product decreases enzyme synthesis (Figure 7.5a) ▪ Specific effect (synthesis of all other enzymes continues normally) ▪ Widespread as control for production of amino acid and nucleotide precursors ▪ Usually, final product of a biosynthetic pathway is the corepressor effector molecule ▪ Typically affects biosynthetic/anabolic enzymes Copyright © 2021, 2018, 2015 Pearson Education, Inc. All Rights Reserved Figure 7.5 Enzyme Repression and Expression of the Arginine Operon Copyright © 2021, 2018, 2015 Pearson Education, Inc. All Rights Reserved Operons: Repression Copyright © 2021, 2018, 2015 Pearson Education, Inc. All Rights Reserved 7.3 Repression and Activation (2 of 8) Enzyme Repression and Induction – Enzyme induction: ▪ opposite of repression ▪ production of an enzyme in response to presence of substrate ▪ typically affects degradative/catabolic enzymes (e.g., lac operon, Figure 7.6) ▪ ensures enzymes are synthesized only when needed Copyright © 2021, 2018, 2015 Pearson Education, Inc. All Rights Reserved Figure 7.6 Enzyme Induction and Expression of the Lactose Operon Copyright © 2021, 2018, 2015 Pearson Education, Inc. All Rights Reserved 7.3 Repression and Activation (3 of 8) Mechanisms of Repression and Derepression – Repressors turn off transcription – Corepressors only bind DNA in presence of its effector. – Example: Arginine becomes corepressor when plentiful ▪ Binds arginine repressor (ArgR) ▪ Results in allosteric change and operator binding ▪ Since arg mRNA is polycistronic, all peptides encoded are repressed Copyright © 2021, 2018, 2015 Pearson Education, Inc. All Rights Reserved 7.3 Repression and Activation (4 of 8) Mechanisms of Repression and Derepression – Some repressors bind in absence of effector, e.g., lac operon (derepression) ▪ LacI (lactose repressor) binds to operator, blocks transcription ▪ If LacI effector present, combines with repressor, causing allosteric change that prevents LacI from binding DNA ▪ Transcription can proceed ▪ Corepressor = inducer (inactivates repressor) ▪ Allolactose and isopropylthiogalactosie (IPTG) are lac inducers Copyright © 2021, 2018, 2015 Pearson Education, Inc. All Rights Reserved 7.3 Repression and Activation (5 of 8) Mechanisms of Repression and Derepression – Repressor’s role is inhibitory (preventing mRNA synthesis), so it is called negative control – Genes are not turned on and off completely; often very low level of basal transcription when “fully repressed” Copyright © 2021, 2018, 2015 Pearson Education, Inc. All Rights Reserved 7.3 Repression and Activation (6 of 8) Mechanisms of Activation – Some operons transcribed only if activator protein first bound to DNA (Figure 7.7) – Promoter sequences are poor matches to consensus promoter sequences, thus only weakly bind RNA polymerase – Positive control: regulator protein facilitates transcription Copyright © 2021, 2018, 2015 Pearson Education, Inc. All Rights Reserved Figure 7.7 Activator Protein Interactions With RNA Polymerase Copyright © 2021, 2018, 2015 Pearson Education, Inc. All Rights Reserved 7.3 Repression and Activation (7 of 8) Mechanisms of Activation – Activator proteins help RNA polymerase recognize promoter. ▪ May bend DNA structure ▪ May interact directly with RNA polymerase – Example: maltose catabolism in E. coli (Figure 7.9) ▪ Maltose activator protein (MalT) cannot bind to DNA unless it first binds maltose (effector/inducer) ▪ Binding of MalT allows RNA polymerase to transcribe – Activator proteins bind specifically to activator-binding site (specific DNA sequence, not called an operator) Copyright © 2021, 2018, 2015 Pearson Education, Inc. All Rights Reserved Figure 7.9 Positive Control of Enzyme Induction in the Maltose Operon Copyright © 2021, 2018, 2015 Pearson Education, Inc. All Rights Reserved 7.3 Repression and Activation (8 of 8) Operons versus Regulons – Genes for maltose are spread out over the chromosome in several operons. (Figure 7.10) ▪ Each operon has an activator-binding site ▪ Maltose activator protein controls transcription of more than one operon – Multiple operons controlled by the same regulatory protein are called a regulon (e.g., maltose regulon) – Regulons also exist for negatively controlled systems (e.g., arginine regulon) – Multiple control features common in many operons and regulons Copyright © 2021, 2018, 2015 Pearson Education, Inc. All Rights Reserved Figure 7.10 Maltose Regulon of Escherichia coli Copyright © 2021, 2018, 2015 Pearson Education, Inc. All Rights Reserved 7.4 Transcription Controls in Archaea (1 of 3) Archaeal transcription regulation more closely resembles control by Bacteria than Eukarya (transcription factors) Archaea use both positive and negative control Repressor proteins in Archaea either block RNA polymerase binding or block binding of TATA-binding protein (TBP) and transcription factor B (TFB), which are required for archaeal RNA polymerase to bind to its promoter Some archaeal activators recruit TBP to promoter, facilitating transcription Copyright © 2021, 2018, 2015 Pearson Education, Inc. All Rights Reserved 7.4 Transcription Controls in Archaea (2 of 3) Control of Nitrogen Assimilation in Archaea – Archaeal repressor example: NrpR from Methanococcus maripaludis (Figure 7.11) ▪ Represses genes involved in nitrogen assimilation when organic nitrogen is plentiful ▪ When alpha-ketoglutarate levels rise, ammonia is limiting, so additional pathways need to be activated (e.g., nitrogen fixation or glutamine synthetase) – Alpha-ketoglutarate functions as inducer, binding to NrpR, which no longer binds and no longer blocks transcription Copyright © 2021, 2018, 2015 Pearson Education, Inc. All Rights Reserved II. Sensing and Signal Transduction Two 7.5 Two-Component Regulatory Systems 7.6 Regulation of Chemotaxis 7.7 Cell-to-Cell Signaling Copyright © 2021, 2018, 2015 Pearson Education, Inc. All Rights Reserved 7.5 Two-Component Regulatory Systems (1 of 3) Prokaryotes regulate cellular metabolism in response to environmental fluctuations – External signal may be transmitted directly to the target – External signal may be detected by sensor and transmitted to regulatory machinery (signal transduction) Copyright © 2021, 2018, 2015 Pearson Education, Inc. All Rights Reserved 7.5 Two-Component Regulatory Systems (2 of 3) Two-Component Regulatory Systems – Most signal transduction systems are two-component regulatory systems containing two parts ▪ sensor kinase (in cytoplasmic membrane): detects environmental signal and autophosphorylates at specific histidine residue (histidine kinase) ▪ response regulator (in cytoplasm): DNA-binding protein that regulates transcription, receives phosphate from sensor kinase – Also has feedback loop ▪ terminates response ▪ uses phosphatase that removes phosphate from response regulator Copyright © 2021, 2018, 2015 Pearson Education, Inc. All Rights Reserved 7.5 Two-Component Regulatory Systems (3 of 3) Examples of Two-Component Regulatory Systems – Almost 50 different two-component systems in E. coli (Table 7.1) ▪ examples include phosphate assimilation, nitrogen metabolism, and osmotic pressure response ▪ e.g., OmpC and OmpF controlled by environment osmolarity; EnvZ sensor histidine kinase detects osmotic pressure changes (Figure 7.14a) – Some signal transduction systems have multiple regulatory elements ▪ e.g., Ntr regulation of nitrogen assimilation Copyright © 2021, 2018, 2015 Pearson Education, Inc. All Rights Reserved Table 7.1 Examples of Two-Component Systems That Regulate Transcription in Escherichia coli System Environmental Sensor kinase Response Primary activity of signal regulator response regulator a regulator Super a Arc system Oxygen ArcB ArcA Repressor/activator Nitrate and nitrite Nitrate and nitrite NarX NarL Activator/repressor respiration (Nar) NarQ NarP Activator/repressor Nitrogen utilization Shortage of NRII (=GlnL) NRI (=GlnG) Activator of promoters (Ntr) organic nitrogen requiring RpoN/ 54 Start fraction R p o N over sigma to the power 54 end fraction. Pho regulon Inorganic PhoR PhoB Activator/repressor phosphate Porin regulation Osmotic pressure EnvZ OmpR Activator/repressor a Note that many response regulator proteins act as both activators and repressors depending on the genes being regulated. Although ArcA can function as either an activator or a repressor, it functions as a repressor on most operons that it regulates. Copyright © 2021, 2018, 2015 Pearson Education, Inc. All Rights Reserved Figure 7.14 Two Examples of Well-Studied Two-Component Systems in Escherichia coli Copyright © 2021, 2018, 2015 Pearson Education, Inc. All Rights Reserved 7.6 Regulation of Chemotaxis (1 of 4) Chemotaxis: Moving toward attractants, away from repellents – respond to temporal gradients (change in concentration over time) – e.g., Pseudomonas aeruginosa (Figure 7.15) Bacteria use modified two-component system to sense temporal changes in attractants or repellents and regulate flagellar rotation – thus regulate activity of preexisting proteins instead of modifying transcription of genes Copyright © 2021, 2018, 2015 Pearson Education, Inc. All Rights Reserved Figure 7.15 Chemotaxis of Pseudomonas aeruginosa Cells Toward Nutrients From Damaged Epithelial Cells Copyright © 2021, 2018, 2015 Pearson Education, Inc. All Rights Reserved 7.6 Regulation of Chemotaxis (2 of 4) Response to Signal – Depends upon signal cascade of multiple proteins – Methyl-accepting chemotaxis proteins (MCPs): Sensory proteins that sense attractants and repellents and interact with cytoplasmic sensor kinases (Figure 7.16) ▪ Chemoreceptors: clusters of thousands of MCPs – E. coli has four transmembrance chemoreceptors, each containing five different MCPs – MCP binding of attractant or repellent triggers interactions with CheA (sensor kinase) and CheW (Figure 7.17) ▪ Increase in repellent increases CheA autophosphorylation ▪ Phosphate transferred to CheY (response regulator) that controls flagellar rotation Copyright © 2021, 2018, 2015 Pearson Education, Inc. All Rights Reserved Figure 7.16 Vibrio Chemoreceptor Arrays Copyright © 2021, 2018, 2015 Pearson Education, Inc. All Rights Reserved Figure 7.17 Mechanism of Chemotaxis in Escherichia coli Copyright © 2021, 2018, 2015 Pearson Education, Inc. All Rights Reserved 7.6 Regulation of Chemotaxis (3 of 4) Controlling Flagellar Rotation – CheY governs direction of rotation – Counterclockwise: run (swim smoothly) – Clockwise: tumble (move randomly) – When MCPs bind repellent or release attractant, CheY-P (phosphorylated) interacts with flagellar motor to induce clockwise rotation and tumbling – When MCPs bind attractant or release repellent, unphosphorylated CheY does not bind to flagellar motor, resulting in counterclockwise rotation and running Copyright © 2021, 2018, 2015 Pearson Education, Inc. All Rights Reserved 7.6 Regulation of Chemotaxis (4 of 4) Adaptation: Stop responding and reset sensory system – feedback loop resets system ▪ relies on response regulator CheB ▪ involves methylation of MCPs – Methylation stops response to attractants and increases response to repellents – When unmethylated, respond strongly to attractants, insensitive to repellents ▪ CheR methytlates, phosphorylated CheB (CheB-P) demethylates Copyright © 2021, 2018, 2015 Pearson Education, Inc. All Rights Reserved 7.7 Regulation of Chemotaxis Other Taxes – Che proteins also play a role in other taxes – phototaxis: movement toward light ▪ Light sensor replaces MCPs – aerotaxis: movement toward oxygen ▪ Redox protein monitors oxygen level – Sensors interact with cytoplasmic Che proteins, leading to runs/tumbles Copyright © 2021, 2018, 2015 Pearson Education, Inc. All Rights Reserved 7.7 Cell-to-Cell Signaling (1 of 5) Prokaryotes can communicate through production of small extracellular molecules – Small peptides or nonpeptide organics – Accumulation leads to coordinated group behaviors, e.g., biofilm formation Quorum sensing: regulatory mechanism by which Bacteria and some Archaea assess their population density Copyright © 2021, 2018, 2015 Pearson Education, Inc. All Rights Reserved 7.7 Cell-to-Cell Signaling (2 of 5) Mechanism of Quorum Sensing – Ensures sufficient number of cells are present before initiating activities that requires a certain cell density to be effective (e.g., toxin production by pathogenic bacterium) – Each species produces a specific autoinducer signaling molecule ▪ diffuses freely across the cell envelope ▪ reaches high concentrations inside cell only if many cells are nearby and making the same autoinducer ▪ binds to specific activator protein or sensor kinase, triggering transcription of specific genes Copyright © 2021, 2018, 2015 Pearson Education, Inc. All Rights Reserved 7.7 Cell-to-Cell Signaling (3 of 5) Mechanism of Quorum Sensing – Several different classes of autoinducers ▪ acyl homoserine lactone (AHL): first to be identified, several types found in different gram-negatives ▪ autoinducer 2 (AI-2): a common autoinducer among many gram-negative species, allowing interspecies communication ▪ short peptides used as autoinducers by gram-positives and Archaea – Quorum sensing first discovered as mechanism regulating light production in bacteria including Aliivibrio fischeri (Figure 7.19) ▪ Lux operon encodes bioluminescence/luciferase – also occurs in microbial eukaryotes (e.g., Saccharomyces cerevisiae and Candida) Copyright © 2021, 2018, 2015 Pearson Education, Inc. All Rights Reserved Figure 7.19 Bioluminescent Bacteria Producing the Enzyme Luciferase Copyright © 2021, 2018, 2015 Pearson Education, Inc. All Rights Reserved 7.7 Cell-to-Cell Signaling (4 of 5) Virulence factors – example: Escherichia coli O157:H7 (Figure 7.20a) ▪ Shiga toxin–producing strain ▪ Produces AHL AI-3 that induces virulence genes ▪ Epinephrine plus norepinephrine (both produced by host) + AI-3 bind to sensor kinases in cytoplasmic membrane. – activates two transcriptional activators, thus activating motility, toxin secretion, and production of lesion-forming proteins Copyright © 2021, 2018, 2015 Pearson Education, Inc. All Rights Reserved Figure 7.20 Quorum Sensing Regulation of Virulence Factors in Two Common Bacterial Pathogens (a) Virulence factor production in Shiga (b) Virulence factor production in toxin-producing Escherichia coli Staphylococcus aureus Copyright © 2021, 2018, 2015 Pearson Education, Inc. All Rights Reserved 7.7 Cell-to-Cell Signaling (5 of 5) Virulence factors – example: Staphylococcus aureus ▪ secretes small peptides that damage host cells or interfere with host’s immune system ▪ under control of autoinducing peptide (AIP) – activates several proteins that lead to production of virulence proteins (Figure 7.20b) – Quorum-sensing disruptors could be potential drugs for dispersing biofilms and preventing virulence gene expression Copyright © 2021, 2018, 2015 Pearson Education, Inc. All Rights Reserved III. Three Global Control 7.8 The lac Operon 7.11 The Heat Shock Response Copyright © 2021, 2018, 2015 Pearson Education, Inc. All Rights Reserved 7.8 The lac Operon (1 of 5) Global control systems: regulate transcription of many different genes in more than one regulon (Table 7.2) – May include activators, repressors, signal molecules, two-component regulatory systems, regulatory RNA, alternative sigma factors as components Copyright © 2021, 2018, 2015 Pearson Education, Inc. All Rights Reserved Table 7.2 Examples of Global Control a Systems Known in Escherichia coli coli Super a System Signal Primary activity of regulatory Number of genes protein regulated Aerobic respiration Presence of O2 O sub 2 Repressor (ArcA) > 50 Anaerobic respiration Lack of O2 O sub 2 Activator (FNR) > 70 Catabolite repression Cyclic AMP level Activator (CRP) > 300 Heat shock Temperature Alternative sigma factors (RpoH > 36 and RpoE) Nitrogen utilization NH3 limitation Activator (NRI)/alternative sigma > 12 factor (RpoN) Oxidative stress Oxidizing agents Activator (OxyR) > 30 SOS response Damaged DNA Repressor (LexA) > 20 General stress response Stress conditions Alternative sigma factor (RpoS) > 400 a For many of the global control systems, regulation is complex. A single regulatory protein can play more than one role. For instance, the regulatory protein for aerobic respiration is a repressor for many promoters but an activator for others, whereas the regulatory protein for anaerobic respiration is an activator protein for many promoters but a repressor for others. Regulation can also be indirect or require more than one regulatory protein. Many genes are regulated by more than one global system. Copyright © 2021, 2018, 2015 Pearson Education, Inc. All Rights Reserved 7.8 The lac Operon (2 of 5) Lactose operon and maltose regulon respond to global controls Catabolite Repression – controls use of carbon sources if more than one present ▪ glucose always used first – Synthesis of unrelated catabolic enzymes (e.g., lactose operon and maltose regulon) is repressed if glucose is present in growth medium – also called “glucose effect” – ensures that the “best” carbon and energy source is used first Copyright © 2021, 2018, 2015 Pearson Education, Inc. All Rights Reserved 7.8 The lac Operon (3 of 5) Catabolite Repression – Can lead to diauxic growth: two exponential growth phases if two energy sources available (Figure 7.21) ▪ Better energy source consumed first, growth stops ▪ After lag, growth resumes with second energy source Copyright © 2021, 2018, 2015 Pearson Education, Inc. All Rights Reserved Figure 7.21 Diauxic Growth of Escherichia coli on a Mixture of Glucose and Lactose Copyright © 2021, 2018, 2015 Pearson Education, Inc. All Rights Reserved 7.8 The lac Operon (4 of 5) Cyclic AMP and Cyclic AMP Receptor Protein – In catabolite repression, transcription is controlled by the cyclic AMP receptor protein (CRP), an activator protein, and is a form of activation – CRP is allosteric and binds to DNA only if it has first bound cyclic adenosine monophosphate (cyclic AMP or cAMP) ▪ regulatory nucleotide derived from adenosine ▪ synthesized by adenylate cyclase Copyright © 2021, 2018, 2015 Pearson Education, Inc. All Rights Reserved 7.8 The lac Operon (5 of 5) Cyclic AMP and Cyclic AMP Receptor Protein – For lac genes to be transcribed (Figure 7.23): ▪ Cyclic AMP level must be high enough for CRP protein to bind to CRP-binding site (positive control) ▪ Lactose or another inducer must be present to prevent lactose repressor (LacI) binding (negative control) Copyright © 2021, 2018, 2015 Pearson Education, Inc. All Rights Reserved Figure 7.23 Overall Regulation of the Lac System Copyright © 2021, 2018, 2015 Pearson Education, Inc. All Rights Reserved 7.11 The Heat Shock Response (1 of 2) Heat shock response: global control mechanism to protect cells from protein denaturation resulting from heat, high solvent levels, osmotic stress, ultraviolet (UV) light Heat Shock Proteins – Temperature and stress can generate large amounts of inactive proteins that need to be refolded or degraded – Heat shock proteins: counteract damage of denatured proteins and help cell recover from stress ▪ five major classes: Hsp100 (proteases that degrade denatured/aggregated proteins), Hsp90, Hsp70 (Dna K), Hsp60 (GroEL), Hsp10 (GroES) Copyright © 2021, 2018, 2015 Pearson Education, Inc. All Rights Reserved