Chapter 5 Carbon Nanomaterials PDF
Document Details
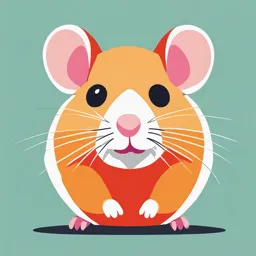
Uploaded by OptimalTrumpet
Nanyang Technological University
Leow Wan Ru
Tags
Summary
This presentation explores carbon nanomaterials, including fullerene, carbon nanotubes, and graphene. It details their structures, properties, and applications. The presentation is part of a course on nanoscience and nanotechnology at Nanyang Technological University.
Full Transcript
Chapter 5: Nanomaterials – Carbon CM4063: Nanoscience and Nanotechnology School of Chemistry, Chemical Engineering and Biotechnology Asst. Prof. Leow Wan Ru Email: [email protected] Learning Objectives By the end of this chapter, you should be able to: Describe carbon nanomaterials. Ident...
Chapter 5: Nanomaterials – Carbon CM4063: Nanoscience and Nanotechnology School of Chemistry, Chemical Engineering and Biotechnology Asst. Prof. Leow Wan Ru Email: [email protected] Learning Objectives By the end of this chapter, you should be able to: Describe carbon nanomaterials. Identify the differences between diamond and graphite. Describe the structure and properties of fullerene. State the applications of fullerene. Describe the structure and properties of carbon nanotubes. State the applications of carbon nanotubes. Explain how graphene is made. Describe the structure and properties of graphene. State the applications of graphene. 2 Topics 1 Introduction 2 Fullerene 3 Carbon nanotube 4 Graphene 5 Summary Reference: Chapter 3 of Chris Binns, Introduction to Nanoscience and Nanotechnology, Wiley- Interscience, 2010 3 5.1 Introduction CM4063: Nanoscience and Nanotechnology School of Chemistry, Chemical Engineering and Biotechnology 5.1 Introduction – Why Carbon? Carbon is a light and simple group IV element. A carbon atom contains just six electrons. Two of them are core (1s) electrons and the remaining four (2sp) are available for bonding with other atoms. Why Carbon? The details of the environment in which the atoms come together (pressure, temperature, etc.) determine the type of bonds. Depending on the bonding, carbon atoms form novel materials with unique properties (electronic, optical, mechanical, thermal, etc.) 5 5.1 Introduction – Diamond and Graphite Diamond Graphite The four valence electrons of each carbon The bonding electrons form a charge atom produce bonding with the nearest distribution of three equally spaced lobes (sp2 neighbours and these electrons form orbitals) in a plane, while the charge tetrahedral structure around each atom distribution of the fourth electron is out of the (sp3 orbitals). plane (sp hybrid orbital). 6 5.1 Introduction – Diamond and Graphite Diamond Graphite It is the hardest natural substance known. It is soft and greasy to touch. It has a high relative density (about 3.5). Its relative density is 2.3. It is transparent and has a high refractive index (2.45). It is black in colour and opaque. It is a non-conductor of heat and electricity. It is a good conductor of heat and electricity. It burns in air at 900°C to form CO2. It burns in air at 700-800°C to form CO2. It occurs as octahedral crystals. It occurs as hexagonal crystals. It is insoluble in all solvents. It is insoluble in all ordinary solvents. 7 5.1 Introduction – The Family of Carbon Nanomaterials 8 5.2 Fullerene CM4063: Nanoscience and Nanotechnology School of Chemistry, Chemical Engineering and Biotechnology 5.2 Fullerene – The Discovery of C60 In 1985 The first fullerene molecule, C60, was discovered in 1985 at Rice University. The discovery occurred almost by accident using the laser vapourisation-mass spectroscopy to vapourise graphite to simulate the carbon chemistry that occurs in the stars. C60, Buckminsterfullerene The team included Robert Curl, Richard Smalley and Harold Kroto, who all shared the 1996 Nobel Prize for Chemistry for their discovery. 10 5.2 Fullerene – The Discovery of C60 Discovery of fullerene: https://www.youtube.com/watch?v=-Aa66KHEuz0&t=19s 11 5.2 Fullerene – The Discovery of C60 Distribution of carbon clusters produced under various experimental conditions: a) Low helium density over graphite target at the time of laser vapourisation. b) High helium density over graphite target at the time of laser vapourisation. c) Same as b), but with addition of ‘integration cup’ to increase time between vapourisation and cluster analysis. 12 5.2 Fullerene – What is C60? C60 has 60 carbon atoms that are arranged as A fullerene is any molecule composed 12 pentagons and 20 hexagons, resembling a entirely of carbon, in the form of a hollow soccer ball. It is the smallest cage that can be sphere. It is also called bucky ball. built out of pentagons and hexagons without having adjacent pentagons. Architecture by Buckminster Fuller © Eberhard von Nellenburg / Wikimedia Commons / CC-BY-SA-3.0 © Souroush83 / Wikimedia Commons / CC-BY-SA-3.0 The bonding is peculiar. One fundamental question is, are these molecules stable? – Yes! 13 5.2 Fullerene – Other Fullerene Structures Types of isomers Smallest: C20, larger one up to C300, Most common: C60 C20 C24 C28 C32 C36 C50 14 5.2 Fullerene – More Information C60 structure Truncated icosahedron (~ football) 20 hexagons and 12 pentagons sp2 bonds in the sphere surface and weaker bonds of the orbitals perpendicular to the surface Diameter of C60 = 0.71 nm Most noted properties Stable, but not totally unreactive Soluble in aromatic solvents "Switching Molecular Orientation of Individual Fullerene at Room Temperature" / Lacheng Liu, Shuyi Liu, Xiu Chen, Chao Li, Jie Ling, Xiaoqing Liu, Yingxiang Cai and Li Wang / Scientific Reports 3, Article Superconductivity number: 3062 (2013) / doi:10.1038/srep03062 15 5.2 Fullerene – Electronic Properties HOMO−LUMO Molecule Gap (eV) The band gap of fullerene is typical Free C60a 1.75 of the electronic bandgap in bulk C60 on Si(100)b isolated molecules 1.8 semiconductors and is controllable C60 on Ag(001) isolated molecules 1.9 – 2.1 by changing the fullerene size and C60 on Au(111) isolated molecules 2.7 the substrate. C60 on Au(887)c 0.5 – 1 monolayer 2.7 Individual fullerene may be useful C60 on polycrystalline Au several layers 2.6 for molecular electronics. C84 on polycrystalline Au several layers 1.13 Ce@C82 on polycrystalline Au several layers 0.88 Dy@C82 on polycrystalline Au several layers 0.86 Binns, C. (2010), Introduction to Nanoscience and Nanotechnology / Wiley-Interscience 16 5.2 Fullerene – Application Polymer-fullerene solar cell Fullerene is an excellent acceptor of electrons. It is added in a solar cell to promote fast electron transfer. 17 5.3 Carbon Nanotubes CM4063: Nanoscience and Nanotechnology School of Chemistry, Chemical Engineering and Biotechnology 5.3 Carbon Nanotubes – Introduction Carbon nanotubes (CNTs) were first identified in the electron microscope images by Sumio Iijima in 1991 while working for NEC in Tsukuba. © 齋藤千絵 / Wikimedia Commons / CC-BY-SA-3.0 Kavli Prize in Nanoscience medal. Retrieved September 29, 2017 from http://www.kavlifoundation. (a) Five-sheet, (b) Two-sheet org/nanoscience Transmission Electron Microscopy and (c) Seven-sheet walled CNT (TEM) images of carbon nanotubes Iijima, S., “Helical Microtubules of Graphitic Carbon”, Nature 354, 56 – 58 (1991) / Radushkevich, L.V., and Lukyanovich, V.M., “The Structure of Carbon Forming in Thermal Decomposition of Carbon Monoxide on an Iron Catalyst”, Soviet J Phys Chem, 26, 88 (1952) 19 5.3 Carbon Nanotubes – Synthesis I. Laser ablation II. Arc discharge In this process, a pulsed laser vapourises a graphite target in a high-temperature CNTs can be found in the carbon soot of reactor while an inert gas is inserted into graphite electrodes during an arc the reactor. discharge involving high current. Nanotubes develop on the cooler surfaces This process yields CNTs with lengths up of the reactor as the vapourised carbon to 50 microns. condenses. 20 5.3 Carbon Nanotubes – Synthesis III. Chemical Vapour Deposition (CVD): Growth of CNTs The first step is to deposit catalyst (Ni, Co). In the second step, anneal the substrate to induce a de-wetting process in vacuum or reducing gas, which will help to reduce the size of the resulting catalyst droplet. The third step, catalytic growth, involves two steps. i. The hydrocarbon gas (ethylene, ethanol, methane) molecule dissociates on the catalyst surface and then the carbon atom diffuses from the free surface to the growth point, either through the droplet or over the catalyst surface. ii. The carbon then extrudes off the catalyst as the growing nanotube. 21 5.3 Carbon Nanotubes – Synthesis Link to video: https://www.youtube.com/watch?v=hkYb35e5JGo 22 5.3 Carbon Nanotubes – Synthesis III. Chemical Vapour Deposition (CVD): Growth of CNTs Good yield CVD is the most widely used method for the production of carbon nanotubes A mat of vertically aligned Single-Walled Nanotubes (SWNTs) grown at 450°C by thermal CVD. IV. Other methods They are natural, incidental and controlled flame environments. 23 5.3 Carbon Nanotubes – Conceptual Structure CNT can be formed by wrapping a graphene sheet into a cylinder. Therefore, there is a need to first understand the geometry of graphene. 24 5.3 Carbon Nanotubes – Conceptual Structure The way the graphene sheet is wrapped strongly affects their properties. Nanotechnology For Dummies (2nd edition), Wiley Publishing 25 5.3 Carbon Nanotubes – Electrical Properties All armchair carbon nanotubes are conducting. In theory, metallic nanotubes can carry an electrical current density of 4 109 A/cm2 which is more than 1,000 times greater than metals such as copper. Therefore, it is a promising candidate for electrical interconnect. Zig-zag carbon nanotubes have a very small bandgap generated by the curvature of the tube. Chiral carbon nanotubes are semiconducting with a bandgap that is inversely proportional to the tube diameter. 26 5.3 Carbon Nanotubes – Applications Transparent electrical conductor Zhuangchun Wu, et al., “Transparent, Conductive Carbon Nanotube Films”, Science, 305, 1273 (2004) CNT transistor CNT computer Room-temperature Max M. Shulaker, et al., transistor based on a “Carbon Nanotube Computer”, single carbon nanotube Nature, 501, 526 (2013) 27 5.3 Carbon Nanotubes – Thermal Properties All nanotubes are expected to be good thermal conductors along the tube, but good insulators lateral to the tube axis. It is predicted that carbon nanotubes will be able to transmit up to 6000 watts per meter per Kelvin (K) at room temperature; compare this to copper, a metal well-known for its good thermal conductivity, which transmits 385 watts per meter per K. The temperature stability of carbon nanotubes is estimated to be up to 2800°C in vacuum and about 750°C in air. 28 5.3 Carbon Nanotubes – Mechanical Properties Carbon nanotubes are one of the strongest known materials. It also has high modulus of elasticity. Young's Tensile Strength Elongation at Material Modulus (TPa) (GPa) Break (%) SWNT ~1 13-53 16 Armchair SWNT 0.94 126 23 Zigzag SWNT 0.94 94 ~16 Chiral SWNT 0.92 MWNT 0.8-0.9 150 Stainless Steel ~0.2 ~0.65 – 1 15-50 Kevlar ~0.15 ~3.5 ~2 29 5.3 Carbon Nanotubes – Mechanical Properties Tensile elongation (190%) of a Double-Walled Carbon Nanotube (DWCNT) at a constant bias voltage of 2.2 V. Link to journal http://journals.aps.org.ezlibproxy1.ntu.edu.sg /prl/abstract/10.1103/PhysRevLett.98.185501 30 5.3 Carbon Nanotubes – Applications CNT Bundles/Yarn Zhang, M., et al., “Multifunctional carbon nanotube yarns by downsizing an ancient technology.”, Science, 306, 1358-1361 (2004) https://www.youtube.com/watch?v=Lt2vGlC4uRc 31 5.3 Carbon Nanotubes – Applications CNT Bundles/Yarn Zhang, M., et al., “Multifunctional carbon nanotube yarns by downsizing an ancient technology.”, Science, 306, 1358-1361 (2004) Bicycle with CNT Composite Atomic Force Microscopy (AFM) Probe Stanislaus S. Wong, et al., Creative Commons 2006 “Covalently functionalised Tour De France by Michael nanotubes as nanometre- David Murphy is licensed sized probes in chemistry under CC-BY-SA-2.0 and biology”, Nature, 394, 52 (1998) 32 5.4 Graphene CM4014: Nanoscience and Nanotechnology School of Physical and Mathematical Sciences 5.4 Graphene – Introduction In 2004, Andre Geim and Konstantin Novoselov at Manchester Nobel Prize by Scotch tape! University managed to peel off single-atom-thick crystallites (graphene) from bulk graphite by using Scotch tape. They shared Nobel prize of physics in 2010 for their 'groundbreaking experiments regarding the two-dimensional material graphene'. Surprisingly and remarkably, graphene is stable. This is contradictory to Mermin-Wagner theorem, which states that a free-standing, two-dimensional crystal would be disrupted by Konstantin Andre Geim thermodynamic forces. Novoselov Nobel Prize of Physics 2010 © Jonathunder / Creative Commons / CC-BY-SA-3.0 Graphite Graphene 34 Trivia Prof Sir Andre Geim is the only person who won both a Nobel prize and Ig Nobel prize The Ig Nobel Prize is a satiric prize awarded annually since 1991 to celebrate ten unusual or trivial achievements in scientific research. Its aim is to "honor achievements that first make people laugh, and then make them think." 35 5.4 Graphene – Introduction Graphene is a one-atom-thick planar sheet of sp2 bonded carbon atoms that are densely packed in a honeycomb crystal lattice. It is the basic building block of graphitic materials, i.e. 0 D: Fullerene, 1 D: CNT and 3D: Graphite. The C-C bond length is 0.142 nm. High resolution TEM images of graphene © AlexanderAIUS / Wikimedia Commons / CC-BY-SA-3.0 Sur, U. K., “Graphene: A Rising Star on the Horizon of Materials Science”, International Journal of Electrochemistry, 2012, (2012-9-20), 2012, 2012 36 5.4 Graphene – Imaging Scanning Tunneling Microscopy Aberration corrected TEM image of (STM) of a single layer graphene a hole in a single layer of graphene Adapted with permission from E. Stolyarova et al., Proc. Nat. Acad. Sci. 104, 9209 (2007). Copyright (2007) National Academy of Sciences, U.S.A. Çağlar Ö. Girit, et al., “Graphene at the Edge: Stability and Dynamics”, Science 323, 1705 (2009) / doi: 10.1126/science.1166999 37 5.4 Graphene – Production Techniques Link to video: https://www.youtube.com/watch?v=rphiCdR68TE 38 5.4 Graphene – Production Techniques Mechanical method Chemical Vapour Deposition Exfoliate graphite using Epitaxial growth on SiC/ metal (30 inches) scotch tapes Using methane as carbon source under H2 condition 39 5.4 Graphene – Large Area S. Bae, et al., “Roll-to-roll production of 30-inch graphene films for transparent electrodes”, Nature Nanotechnology 5, 574-578 (2010) / doi: 10.1038/nnano.2010.132 40 5.4 Graphene – Production Techniques Please visit the following links for some examples. http://www.youtube.com/watch?v=PifL8bAybyc http://www.youtube.com/watch?v=rphiCdR68TE 41 5.4 Graphene – Bandgap LUMO: Conduction band HOMO: Valance band Pristine graphene is a semi-metal with zero bandgap. 42 5.4 Graphene – Highly Tunable Properties The Fermi level EF (highest energy level) of graphene can be easily tuned by electrical or chemical doping, offering highly tunable properties of graphene. Chemical method Electrical method Tuning the EF and open the bandgap Tuning the EF only 43 5.4 Graphene – Electrical Properties High electron mobility at room temperature, which is desired in electronic devices. (mobility) = vavg / E (velocity / electric field) 44 5.4 Graphene – Optical Properties Optical transmittance control: Transparent electrode Optical absorption of single layer: 2.3% F. Bonaccorso, et al., “Graphene photonics and optoelectronics”, Nature Photonics 4, 611 - 622 (2010) / doi: 10.1038/nphoton.2010.186 45 5.4 Graphene – Mechanical Properties Mechanical strength for flexible and stretchable devices. Young’s modulus = tensile stress/tensile strain = 1 TPa (1012) Diamond 1.2 TPa Force-displacement measurement C. Lee, et al., “Measurement of the elastic properties and intrinsic strength of monolayer graphene.”, Science 321, 385 – 388 (2008) / doi: 10.1126/science.1157996 46 5.4 Graphene – Properties Physical properties Thermal properties Low density 2 g/cm3 Excellent conductor > 4800 High specific surface area W/m/K (due to phonon (limit: 2630 m2/g) Chemical properties effect) Very resistant to fracture (tensile strength 130 GPa) Can be modified by strong acid, followed by reduction Mechanical properties by hydrazine/H2 Electrical properties Very resistant to fracture High electron mobility – up (tensile strength 130 GPa) to 15000 cm/V.s Young modulus E 0.5 TPa Quantum Hall effect 47 5.4 Graphene – Applications Graphene-based touch screen panel Schematic of the roll-based production of graphene films grown on a copper foil. A transparent ultra large-area graphene film transferred on a 35-inch PET sheet. A graphene-based touch-screen panel connected to a computer with control software. S. Bae, et al., “Roll-to-roll production of 30-inch graphene films for transparent electrodes”, Nature Nanotechnology 5, 574-578 (2010) / doi: 10.1038/nnano.2010.132 48 5.4 Graphene – Applications Graphene-based optoelectronics F. Bonaccorso, et al., “Graphene photonics and optoelectronics”, Nature Photonics 4, 611 - 622 (2010) / doi: 10.1038/nphoton.2010.186 49 5.4 Graphene – Applications 1 Flexible electronics (plastic electronics) 2 Nanoelectronic devices (logic, memory) 3 Lightweight electrical conductor (electric grid, high tension wire) Structural (remarkably high specific strength; wind turbine blades, flywheel, 4 aerospace, transportation, high power transmission lines, etc.) 5 Electrical energy storage (electrode material in supercapacitor, batteries, etc.) Thermal management (nanoscale, microscale, macroscale for HVAC, giant 6 computer servers, etc.) 50 5.4 Graphene – Applications 7 Large area, transparent heating element 8 Transparent conductive films (image display, solar PV, etc.) 9 Impermeable films; barrier resistance (passivate Cu; food packaging, OPV, etc.) Polymer, ceramic matrix composites; as paper-like materials but with unusual 10 mechanical properties 11 Sensors: Chem- and bio-sensors; pressure sensors, strain gauge, etc. Electric power generation: Fuel cells, possibly solar thermal; as transparent 12 conductive electrode; electron or hole “sponge” in OPV’s 51 5.5 Summary CM4014: Nanoscience and Nanotechnology School of Physical and Mathematical Sciences Carbon Nanomaterials – Summary of Properties Structure sp2 bond sp2 bond sp2 bond Armchair – metal Superconductivity High electron mobility Electrical Zigzag and chiral - (@ T < 40K) ~ 200000 cm2/V.s semiconductor Heat resistance, Thermal Good thermal - 3500 W/m.K > 4800 W/m/K 0.4 W/mK tensile strength, σ ~ 63 σ ~ 130 GPa Mechanical GPa E ~ 1 TPa E ~ 1 TPa Integrated circuit, Organic Electrical circuit Potential Transparent conducting Photovoltaics, Paper batteries applications electrodes, transistor; Catalyst, Antioxidants Reinforced composite Gas sensor 53 Carbon Nanomaterials – Key Takeaways Key points discussed in this chapter: Carbon-based nanomaterials manifest a number of extraordinary properties and applications. It is the bonding and structures that determine the properties of these materials. The practical applications are very promising. 54