Chapter 19 Benzene and Aromatic Compounds PDF
Document Details
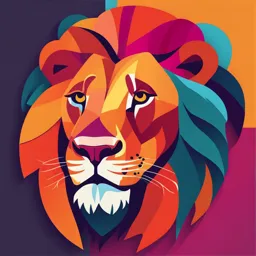
Uploaded by OpulentNirvana
University of Hawai'i
Janice Gorzynski Smith
Tags
Summary
This document is a chapter on benzene and aromatic compounds from an organic chemistry textbook. The chapter includes definitions, explanations, and illustrations of key concepts regarding benzene and aromatic compounds. It describes properties, reactions, and nomenclature.
Full Transcript
Organic Chemistry with Biological Topics, Sixth Edition Janice Gorzynski Smith University of Hawai’i Chapter 19 Lecture Outline Prepared by Andrea Leonard University of Louisiana at Lafayette ©2021 McGraw Hill. All rights reserved. Authorized only for instructor use in the classroom. No reproductio...
Organic Chemistry with Biological Topics, Sixth Edition Janice Gorzynski Smith University of Hawai’i Chapter 19 Lecture Outline Prepared by Andrea Leonard University of Louisiana at Lafayette ©2021 McGraw Hill. All rights reserved. Authorized only for instructor use in the classroom. No reproduction or further distribution permitted without the prior written consent of McGraw Hill. 1 Benzene Benzene is the simplest aromatic hydrocarbon (or arene). Benzene has four degrees of unsaturation, making it a highly unsaturated hydrocarbon. Whereas unsaturated hydrocarbons such as alkenes, alkynes, and dienes readily undergo addition reactions, benzene does not. ©McGraw Hill 2 Reactivity of Benzene Benzene does react with bromine, but only in the presence of (a Lewis acid), and results in substitution, not addition. Proposed structures of benzene must account for its high degree of unsaturation and its lack of reactivity towards electrophilic addition. ©McGraw Hill 3 Benzene Background August Kekulé proposed that benzene was a rapidly equilibrating mixture of two compounds, each containing a six-membered ring with three alternating bonds. In the Kekulé description, the bond between any two carbon atoms is sometimes a single bond and sometimes a double bond. These structures are known as Kekulé structures. Although benzene is still drawn as a six-membered ring with alternating bonds, in reality there is no equilibrium between the two different kinds of benzene molecules. Current descriptions of benzene are based on resonance and electron delocalization due to orbital overlap. ©McGraw Hill 4 Structure of Benzene Any structure for benzene must account for the following facts: 1) It contains a six-membered ring and three additional degrees of unsaturation. 2) It is planar. 3) All bond lengths are equal. The Kekulé structures satisfy the first two criteria but not the third, because having three alternating bonds means that benzene should have three short double bonds alternating with three longer single bonds. ©McGraw Hill 5 Resonance Hybrid of Benzene The resonance description of benzene consists of two equivalent Lewis structures, each with three double bonds that alternate with three single bonds. The true structure of benzene is a resonance hybrid of the two Lewis structures, with the dashed lines of the hybrid indicating the position of the bonds. We will use one of the two Lewis structures and not the hybrid in drawing benzene. This will make it easier to keep track of the electron pairs in the bonds (the electrons). ©McGraw Hill 6 Representations of Benzene Because each π bond has two electrons, benzene has six π electrons. In benzene, the actual bond length (1.39 Å) is intermediate between the carbon–carbon single bond (1.53 Å) and the carbon–carbon double bond (1.34 Å). ©McGraw Hill 7 Benzene Bond Lengths Each carbon atom in a benzene ring is surrounded by three atoms and no lone pairs of electrons, making it hybridized. Each carbon is then trigonal planar with bond angles. Each carbon also has a p orbital with one electron that extends above and below the plane of the molecule. ©McGraw Hill 8 Electron Density in Benzene Overlap of six adjacent p orbitals creates two rings of e- density, one above and one below the plane of the benzene ring. These electrons make benzene electron-rich and reactive with strong electrophiles. Figure 19.1 ©McGraw Hill 9 Naming Substituted Benzenes To name a benzene ring with one substituent, name the substituent and add the word benzene. Many monosubstituted benzenes have common names which you must also learn. ©McGraw Hill 10 Nomenclature of Benzene Derivatives 1 There are three different ways that two groups can be attached to a benzene ring, so a prefix—ortho, meta, or para—can be used to designate the relative position of the two substituents. ©McGraw Hill 11 Nomenclature of Benzene Derivatives 2 If the two groups on the benzene ring are different, alphabetize the names of the substituents preceding the word benzene. If one substituent is part of a common root, name the molecule as a derivative of that monosubstituted benzene. ©McGraw Hill 12 Rules for Naming Benzene Derivatives For three or more substituents on a benzene ring: 1. Number to give the lowest possible numbers around the ring. 2. Alphabetize the substituent names. 3. When substituents are part of common roots, name the molecule as a derivative of that monosubstituted benzene. The substituent that comprises the common root is located at C1. ©McGraw Hill 13 Naming Benzene as a Substituent A benzene substituent is called a phenyl group, and it can be abbreviated in a structure as “Ph−.” ©McGraw Hill 14 Benzyl and Aryl Groups 1 The benzyl group, another common substituent that contains a benzene ring, differs from a phenyl group. Substituents derived from other substituted aromatic rings are collectively known as aryl groups. ©McGraw Hill 15 Benzyl and Aryl Groups 2 Table 19.1 Characteristic Spectroscopic Absorptions of Benzene Derivatives ©McGraw Hill 16 𝟏𝟑𝐂 NMR Absorptions of Dibromobenzenes Figure 19.2 ©McGraw Hill 17 Benzo[a]pyrene, a Common PAH Benzo[a]pyrene, produced by the incomplete oxidation of organic compounds in tobacco, is found in cigarette smoke. When ingested or inhaled, benzo[a]pyrene and other similar PAHs are oxidized to carcinogenic products. Figure 19.3 ©McGraw Hill 18 Synthetic PAHs Figure 19.4 Helicene and twistoflex—Two synthetic polycyclic aromatic hydrocarbons In helicene all the rings twist slightly creating a rigid helical shape that prevents the hydrogens on both ends from colliding. Twistoflex is also non-planar to reduce steric interactions. Both of these molecules are examples of chiral molecules that do not contain stereogenic centers. ©McGraw Hill 19 Stability of Benzene Consider the heats of hydrogenation of cyclohexene, 1,3cyclohexadiene and benzene: • All three give cyclohexane when treated with excess hydrogen in the presence of a metal catalyst. • However, the for benzene is much less than predicted. ©McGraw Hill 20 Heats of Hydrogenation for Benzene Figure 19.5 The huge difference between the hypothetical and observed heats of hydrogenation for benzene cannot be explained solely on the basis of resonance and conjugation. The low heat of hydrogenation of benzene means that benzene is especially stable—even more so than conjugated polyenes. This unusual stability is characteristic of aromatic compounds. ©McGraw Hill 21 Unusual Reactivity of Benzene Benzene’s unusual behavior is not limited to hydrogenation. Benzene does not undergo addition reactions typical of other highly unsaturated compounds, including conjugated dienes. Benzene does not react with to yield an addition product. Instead, in the presence of a Lewis acid, bromine substitutes for a hydrogen atom, yielding a product that retains the benzene ring. ©McGraw Hill 22 The Criteria for Aromaticity 1 Four structural criteria must be satisfied for a compound to be aromatic: 1. A molecule must be cyclic. To be aromatic, each p orbital must overlap with p orbitals on adjacent atoms. ©McGraw Hill 23 The Criteria for Aromaticity 2 2. A molecule must be planar. All adjacent p orbitals must be aligned so that the electron density can be delocalized. Since cyclooctatetraene is nonplanar and not aromatic, it undergoes addition reactions just like those of other alkenes. ©McGraw Hill 24 The Criteria for Aromaticity 3 3. A molecule must be completely conjugated. Aromatic compounds must have a p orbital on every atom. ©McGraw Hill 25 The Criteria for Aromaticity 4 A molecule must satisfy Hückel’s rule, and contain a particular number of electrons. Hückel's rule: Benzene is aromatic and especially stable because it contains 6 electrons. Cyclobutadiene is antiaromatic and especially unstable because it contains 4 electrons. ©McGraw Hill 26 Hückel’s Rule and Number of π Electrons 1 Hückel’s rule refers to the number of electrons, not the number of atoms in a particular ring. Table 19.2 The Number of π Electrons that Satisfy Hückel’s Rule ©McGraw Hill n 4n + 2 0 2 1 6 2 10 3 14 4, etc. 18 27 Aromatic, Antiaromatic, and Nonaromatic Considering aromaticity, a compound can be classified in one of three ways: 1. Aromatic—A cyclic, planar, completely conjugated compound with electrons 2. Antiaromatic—A cyclic, planar, completely conjugated compound with electrons 3. Not aromatic (nonaromatic)—A compound that lacks one (or more) of the following requirements for aromaticity; being cyclic, planar, and completely conjugated ©McGraw Hill 28 Larger Aromatic Rings Completely conjugated rings larger than benzene are also aromatic if they are planar and have 4n + 2 electrons. Hydrocarbons containing a single ring with alternating double and single bonds are called annulenes. To name an annulene, indicate the number of atoms in the ring in brackets and add the word annulene. ©McGraw Hill 29 Hückel’s Rule and Number of π Electrons 2 [10]-Annulene has 10 electrons, which satisfies Hückel's rule, but a planar molecule would place the two H atoms inside the ring too close to each other. Thus, the ring puckers to relieve this strain. Since [10]-annulene is not planar, the 10 electrons cannot delocalize over the entire ring and it is not aromatic. ©McGraw Hill 30 Fused Ring Aromatics Two or more six-membered rings with alternating double and single bonds can be fused together to form PAHs. There are two different ways to join three rings together, forming anthracene and phenanthrene. ©McGraw Hill 31 Resonance Structures of Fused Ring Aromatics As the number of fused rings increases, the number of resonance structures increases. Naphthalene is a hybrid of three resonance structures whereas benzene is a hybrid of two. ©McGraw Hill 32 Ionic Aromatic Compounds Both negatively and positively charged ions can be aromatic if they possess all the necessary elements. We can draw five equivalent resonance structures for the cyclopentadienyl anion. ©McGraw Hill 33 Cyclopentadienyl Compounds Having the “right” number of electrons is necessary for a species to be unusually stable due to aromaticity. Thus, although five resonance structures can also be drawn for the cyclopentadienyl cation and radical, only the cyclopentadienyl anion has 6 electrons, a number that satisfies Hückel’s rule. ©McGraw Hill 34 Cyclopentadienyl Anion Cyclopentadiene itself is not aromatic because it is not fully conjugated. However, cyclopentadiene is much more acidic (pKa=15) than most hydrocarbons because its conjugate base is aromatic and therefore, very stable. Loss of a proton causes the cyclopentadienyl anion to become fully conjugated and consequently aromatic. ©McGraw Hill 35 Tropylium Cation The tropylium cation is a planer carbocation with three double bonds and a positive charge contained in a seven-membered ring. Because the tropylium cation has three bonds and no other nonbonded electron pairs, it contains six electrons and is fully conjugated, thereby satisfying Hückel’s rule. ©McGraw Hill 36 Heterocyles Heterocycles containing oxygen, nitrogen or sulfur, can also be aromatic. With heteroatoms, we must determine whether the lone pair is localized on the heteroatom or part of the delocalized system. An example of an aromatic heterocycle is pyridine. ©McGraw Hill 37 Aromatic Heterocycles Pyrrole is another example of an aromatic heterocycle. It contains a fivemembered ring with two bonds and one nitrogen atom. Pyrrole has a p orbital on every adjacent atom, so it is completely conjugated. Pyrrole has six electrons—four from the bonds and two from the lone pair. Pyrrole is cyclic, planar, completely conjugated, and has electrons, so it is aromatic. ©McGraw Hill 38 Electrostatic Potential of Aromatic Heterocycles In pyridine, the nonbonded electron pair is localized on the N atom in an hybridized orbital • This can be seen by the region of high electron density on N. In pyrrole, the nonbonded electron pair is in a p orbital and is delocalized over the ring. • The entire ring is electron rich. Figure 19.6 Electrostatic potential maps of pyridine and pyrrole ©McGraw Hill 39 Biologically Active Heterocycles Histamine is a biologically active amine formed in many tissues. It is an aromatic heterocycle with two N atoms—one which is similar to the N atom of pyridine, and the other which is similar to the N atom of pyrrole. ©McGraw Hill 40 Deoxyribonucleic Acid DNA contains four different bases, derived from the heterocycles pyrimidine and purine. The lone pair electrons on N atoms in both structures reside in sp 2 hybridized orbitals perpendicular to the aromatic pi system. ©McGraw Hill 41 DNA bases Cytosine and thimine are derived from pyrimidine and contain six pi electrons. Adenine and guanine are derived from purine and contain 10 pi electrons. ©McGraw Hill 42 The Basis of Hückel’s Rule Why does the number of electrons determine whether a compound is aromatic? The basis of aromaticity can be better understood by considering orbitals and bonding. ©McGraw Hill 43 Molecular Orbital Theory Thus far, we have used “valence bond theory” to explain how bonds between atoms are formed. Valence bond theory is inadequate for describing systems with many adjacent p orbitals that overlap, as is the case in aromatic compounds. Molecular orbital (MO) theory permits a better explanation of bonding in aromatic systems. • MO theory describes bonds as the mathematical combination of atomic orbitals that form a new set of orbitals called molecular orbitals (MOs). • A molecular orbital occupies a region of space in a molecule where electrons are likely to be found. ©McGraw Hill 44 Forming Molecular Orbitals When forming molecular orbitals from atomic orbitals, keep in mind that a set of n atomic orbitals forms n molecular orbitals. If two atomic orbitals combine, two molecular orbitals are formed. Recall that aromaticity is based on p orbital overlap. Also note that the two lobes of each p orbital are opposite in phase, with a node of electron density at the nucleus. When two p orbitals combine, two molecular orbitals should form. ©McGraw Hill 45 Bonding and Antibonding Orbitals The combination of two p orbitals can be constructive—that is, with like phases interacting—or destructive, that is, with opposite phases interacting. When two p orbitals of similar phase overlap side-by-side, a bonding molecular orbital results. When two p orbitals of opposite phase overlap side-by-side, a antibonding orbital results. ©McGraw Hill 46 Formation of π and π^∗ Molecular Orbitals Two atomic p orbitals combine to form two molecular orbitals. The bonding MO is lower in energy than the two p orbitals. The antibonding MO is higher in energy because a destabilizing node results, which pushes nuclei apart when orbitals of opposite phase combine. Figure 19.7 ©McGraw Hill 47 Molecular Orbitals for Benzene 1 The molecular orbital description of benzene is much more complex than the two Mos formed in Figure 15.8. Since each of the 6 C atoms of benzene has a p orbital, 6 atomic p orbitals combine to form 6 pi molecular orbitals as shown in Figure 15.9. • The 6 MOs are labeled Ψ1 to Ψ6. • Ψ1 is the lowest energy and Ψ6 is the highest. • There are three bonding orbitals: Ψ1, Ψ2, and Ψ3. • There are three antibonding orbitals: Ψ4*, Ψ5*, and Ψ6* ©McGraw Hill 48 Molecular Orbitals for Benzene 2 Figure 19.8 How the six p orbitals of benzene overlap to form six molecular orbitals ©McGraw Hill 49 Molecular Orbitals for Benzene 3 The most important features of the six benzene MOs: • The larger the number of bonding interactions, the lower in energy the MO. • The larger the number of nodes, the higher in energy the M O. • Two pairs of MOs with the same energy ( and ; and ) are called degenerate orbitals. • The highest energy orbital that contains electrons is called the highest occupied molecular orbital (HOMO). • The lowest energy orbital that does not contain electrons is called the lowest unoccupied molecular orbital (LUMO). ©McGraw Hill 50 Inscribed Polygon Method of Predicting Aromaticity 1 ©McGraw Hill 51 Inscribed Polygon Method of Predicting Aromaticity 2 ©McGraw Hill 52 Inscribed Polygon Method of Predicting Aromaticity 3 This method works for all monocyclic completely conjugated systems regardless of ring size. The total number of MOs always equals the number of vertices of the polygon. The inscribed polygon method is consistent with Hückel's rule—there is always one lowest energy bonding MO that can hold twoelectrons and the other bonding MOs come in degenerate pairs that can hold a total of four electrons. ©McGraw Hill 53 Inscribed Polygon Method of Predicting Aromaticity 4 Figure 19.9 ©McGraw Hill 54 MO Patterns Leading to Aromaticity For the compound to be aromatic, these MOs must be completely filled with electrons, so the “magic numbers” for aromaticity fit Hückel’s rule. Figure 19.10 MO patterns for cyclic, completely conjugated systems ©McGraw Hill 55 Pi Stacking Compounds that contain aromatic rings with loosely held pi electrons exhibit pi stacking. Parallel pi stacking occurs with two aromatic rings stack on top of each other. Perpendicular pi stacking occurs with the p orbitals of the two aromatic rings are at a 90 degree angle. Cation-pi stacking occurs when an electron-rich aromatic ring is attracted to a positively charged ion. ©McGraw Hill 56 Pi Stacking Examples ©McGraw Hill 57 Biologial Cation-Pi Stacking Figure 19.11 Protein ribbon diagram for aromatase with enlargement of the active site ©McGraw Hill 58