Gene Regulation in Eukaryotes I PDF
Document Details
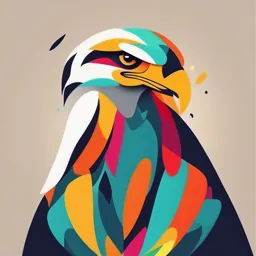
Uploaded by SimplerFoxglove
University of Minnesota
Robert J. Brooker
Tags
Summary
This chapter focuses on gene regulation in eukaryotes, specifically on the general features of transcriptional regulation. It discusses gene expression, regulatory transcription factors, enhancers, chromatin remodeling, and histone modifications as key elements in controlling gene activity.
Full Transcript
Because learning changes everything. ® Chapter 15 Gene Regulation in Eukaryotes I: General Features of Transcriptional Regulation Genetics: Analysis & Principles EIGHTH EDITION Robert J. Brooker © McGraw Hill LLC. All rights reserved. No reproduction or distribution w...
Because learning changes everything. ® Chapter 15 Gene Regulation in Eukaryotes I: General Features of Transcriptional Regulation Genetics: Analysis & Principles EIGHTH EDITION Robert J. Brooker © McGraw Hill LLC. All rights reserved. No reproduction or distribution without the prior written consent of McGraw Hill LLC. Gene Regulation In Eukaryotes I: General Features of Transcriptional Regulation © McGraw Hill Courtesy of Song Tan, Penn State University. 2 Introduction Gene expression - process by which the information within a gene is used to synthesize RNA and polypeptides, and to affect the properties of cells and the phenotype of multicellular organisms Gene regulation - the level of gene expression can vary under different conditions © McGraw Hill 3 Introduction Importance of Gene regulation 1. Respond to environmental changes such as nutrient availability, stress, etc. 2. Produce different cells types in multicellular species 3. Facilitate changes during development Some genes are only expressed during embryonic stages, whereas others are only expressed in the adult © McGraw Hill 4 Regulation Of Gene Expression Transcription Regulatory transcription factors- activate or inhibit transcription Arrangement and composition of nucleosomes DNA methylation RNA Modification Alternative splicing and RNA editing Translation Proteins regulate translation or mRNA degradation RNA interference Posttranslation Feedback inhibition and covalent modifications regulate protein function © McGraw Hill 5 Combinatorial Control Most eukaryotic genes are regulated by many factors Common factors contributing to combinatorial control are: 1. One or more activator proteins may stimulate transcription 2. One or more repressor proteins may inhibit transcription 3. Activators and repressors may be modulated by binding of small effector molecules, protein-protein interactions, and covalent modifications 4. Regulatory proteins may alter nucleosomes near the promoter 5. DNA methylation may inhibit transcription 6. Heterochromatin formation may inhibit transcription (Chapter 16) © McGraw Hill 6 15.1 Regulatory Transcription Factors and Enhancers Transcription factors are proteins that influence the ability of RNA polymerase to transcribe a given gene There are two main types General transcription factors Required for the binding of the RNA polymerase to the core promoter and its progression to the elongation stage Necessary for basal transcription Discussed in Chapter 12 © McGraw Hill 7 15.1 Regulatory Transcription Factors and Enhancers Regulatory transcription factors Serve to regulate the rate of transcription of target genes They typically influence the ability of RNA polymerase to begin transcription of a particular gene © McGraw Hill 8 Structural Features of Regulatory Transcription Factors Transcription factor proteins contain regions, called domains, that have specific functions DNA-binding Binding site for small effector molecules Dimerization A motif is a domain, or a portion of a domain, that has a very similar structure in many different proteins Figure 15.1 depicts several different domain structures found in transcription factor proteins © McGraw Hill 9 (a) Helix-turn-helix motif (b) Helix-loop-helix motif Fig 15.1a/b © McGraw Hill 10 (c) Zinc finger motif (d) Leucine finger motif Fig 15.1b/c © McGraw Hill 11 Regulatory Transcription Factors Regulatory transcription factors recognize cis regulatory elements within enhancers These sequences are known as regulatory elements, control elements, or regulatory sequences An enhancer is a DNA region, usually 50-1000 bp in length, that contains one or more regulatory elements The binding of regulatory transcription factors to regulatory elements affects the transcription of an associated gene A regulatory protein that increases the rate of transcription is termed an activator A regulatory protein that decreases the rate of transcription is termed a repressor © McGraw Hill 12 Fig 15.2 © McGraw Hill 13 Enhancers and Silencers The binding of an activator to an enhancer increases the rate of transcription This up-regulation can be 10- to 1,000-fold The binding of a repressor to an enhancer that inhibits transcription is down-regulation © McGraw Hill 14 Enhancers and Silencers Many regulatory elements within enhancers are orientation independent or bidirectional They can function in the forward or reverse orientation 5’-GATA-3’ or 5’-TATC-3’ 3’-CTAT-5’ 3’-ATAG-5’ Some regulatory elements are located within a few hundred nucleotides upstream of the promoter However, they are often found at various other sites Several thousand (even 100,000) nucleotides away Downstream from the promoter Even within introns!!! © McGraw Hill 15 Modulation of Regulatory Transcription Factor Functions Three common ways the function of regulatory transcription factors can be modulated: 1. Binding of a small effector molecule 2. Protein-protein interactions 3. Covalent modification © McGraw Hill 16 (a) Binding of a small effector molecule such as hormone (b) Protein-protein interaction Fig 15.3a/b © McGraw Hill 17 (c) Covalent modification such as phosphorylation Fig 15.3c © McGraw Hill 18 15.2 Chromatin Remodeling, Histone Variants, and Histone Modifications The three-dimensional packing of chromatin is an important parameter affecting gene expression Chromatin is a very dynamic structure that can alternate between two conformations Closed conformation Chromatin may be tightly packed Transcription may be difficult or impossible Open conformation Chromatin is accessible to transcription factors Transcription can take place © McGraw Hill 19 15.2 Chromatin Remodeling, Histone Variants, and Histone Modifications Researchers have also found that the following can affect gene transcription: Positioning of nucleosomes at or near promoters Presence of histone variants Covalent modification of histones © McGraw Hill 20 Chromatin Remodeling ATP-dependent chromatin remodeling, or chromatin remodeling, remodeling refers to dynamic changes in chromatin structure These changes range from a few nucleosomes to large scale changes Carried out by diverse multiprotein machines that reposition and restructure nucleosomes © McGraw Hill 21 Chromatin Remodeling Complexes Alter the Positions and Compositions of Nucleosomes Nucleosomes, which are discussed in Chapter 10, have been shown to change position in cells that normally express a particular gene compared with cells in which the gene is inactive A key role of some transcriptional activators is to orchestrate changes in chromatin structure One way is through ATP-dependent chromatin remodeling Energy of ATP hydrolysis is used to drive changes in the locations and/or composition of nucleosomes Makes the DNA more or less amenable to transcription © McGraw Hill 22 ATP-dependent Chromatin Remodeling Chromatin remodeling complexes change chromatin structure in one of 3 ways: Change in the position of nucleosomes Eviction of histone octamers Change in the composition of nucleosomes © McGraw Hill 23 (a) Change in nucleosome position Fig 15.4a © McGraw Hill 24 (b) Histone eviction Fig 15.4b © McGraw Hill 25 (c) Replacement with histone variants Fig 15.4c © McGraw Hill 26 Histone Variants Play Specialized Roles in Chromatin Structure and Function Histone proteins are described in Chapter 10. The five types of histone genes are moderately repetitive H1, H2A, H2B, H3 and H4 Human genome contains over 70 histone genes Most code standard histones A few of these genes have accumulated mutations that alter the amino acid sequence These are termed histone variants © McGraw Hill 27 Histone Variants Play Specialized Roles in Chromatin Structure and Function Histone variants are incorporated into a subset of nucleosomes to create specialized chromatin (see Table 15.1 in your textbook) Some histone variants promote an open chromatin conformation Other histone variants promote a closed conformation Therefore, changes in the composition of histone variants can regulate gene transcription Other functions include DNA repair and chromosome segregation (which we will not focus on in this chapter) © McGraw Hill 28 Histone Code Over 50 enzymes have been identified in mammals that covalently modify the amino terminal tails of histones These covalent modifications affect the level of transcription in 2 main ways 1. May influence interactions between the DNA and histone proteins 2. Occur in patterns that are recognized by proteins Called the histone code The pattern of modifications provide binding sites for proteins that promote alterations in chromatin structure These proteins bind to histones based on the code and affect transcription © McGraw Hill 29 Histone Modifications and their Effects on Nucleosome Structure Acetylation, methylation and phosphorylation are common covalent modifications that occur on histones (see Figure 15.10) p = phosphate ac = acetyl group m = methyl group © McGraw Hill 30 One code recognized by certain proteins is phosphorylation of serine at position 1 in H2A and acetylation of lysine at the 5th and 8th positions in H4. (a) Examples of possible histone modifications Fig 15.5a © McGraw Hill 31 Acetylation of positively charged lysines eliminates the positive charge and causes the DNA to less tightly bound Facilitates transcription (b) Effect of acetylation Fig 15.5b © McGraw Hill 32 15.3 DNA Methylation DNA methylation is the covalent attachment of methyl groups (-CH3) Carried out by DNA methyltransferase It is common in some eukaryotic species, but not all Yeast and Drosophila have little DNA methylation Vertebrates and plants have abundant DNA methylation In mammals, ~ 2 to 7% of the DNA is methylated DNA methylation usually inhibits eukaryotic gene transcription © McGraw Hill 33 (a) The methylation of cytosine Fig 15.6a © McGraw Hill 34 (b) Unmethylated (c) Hemimethylated (d) Fully methylated Fig 15.b-d © McGraw Hill 35 CpG Islands In vertebrates and plants, many genes contain CpG islands near their promoters These CpG islands are 1,000 to 2,000 nucleotides long Contain high number of CpG sites In housekeeping genes The CpG islands are unmethylated Genes tend to be expressed in most cell types © McGraw Hill 36 CpG Islands In tissue-specific genes The expression of these genes may be silenced by the methylation of CpG islands Methylation may influence the binding of transcription factors Methyl-CpG-binding proteins may recruit factors that lead to compaction of the chromatin © McGraw Hill 37 (a) Methylation inhibits the binding of an activator protein. Fig 15.7a © McGraw Hill 38 (b) Methyl-CpG-binding protein recruites other proteins that change the chromatin to a closed conformation. Fig 15.7b © McGraw Hill 39 DNA Methylation is Heritable Methylated DNA sequences are inherited during cell division; May explain genomic imprinting (Chapter 5/16) Specific genes are methylated in gametes from female or male parent via de novo methylation Pattern of one copy of the gene being methylated and the other not is maintained in the resulting offspring via maintenance methylation © McGraw Hill 40 Molecular Model for Inheritance of DNA Methylation De novo methylation is an infrequent and highly regulated event Fig 15.8 © McGraw Hill 41 15.4 Gene Activation and Gene Repression Gene activation - a series of events that allow a gene to be transcribed to produce an RNA molecule. For eukaryotic genes controlled by regulatory transcription factors: 1. One or more regulatory transcription factors (activators) bind to an enhancer. 2. The activators recruit coactivators (chromatin remodeling complexes and histone-modifying enzymes) 3. RNA polymerase binds to the core promoter to form a preinitiation complex. 4. RNA polymerase proceeds to the elongation phase and makes an RNA transcript. © McGraw Hill 42 Nucleosome Arrangements in the Vicinity of a Protein- encoding Gene A nucleosome-free region (NFR) is found at the beginning and end of many (most) eukaryotic genes. Nucleosomes tend to be precisely positioned near the beginning and end of a gene, but are less regularly distributed elsewhere Fig 15.9 © McGraw Hill 43 Transcriptional Activation Transcriptional activation involves changes in nucleosome position and composition and modifications to histones Activators, which may bind within the NFR near the core promoter or at distance enhancers, recruit chromatin remodeling complexes and histone-modifying enzymes to the promoter region © McGraw Hill 44 Changes that occur to form a Pre-initiation Complex 1. Binding of activators: Activation protein binds to regulatory elements within enhancer sequences. The enhancer may be close to the transcriptional start site or far away (as shown here). 2. Recruitment of coactivators: Activators bind directly to the DNA of regulatory elements within the enhancer, then recruit coactivators Proteins that increase transcription rate but do not bind directly to the DNA. Can enhance transcription (chromatin remodeling, histone modification, recruitment or stimulation of the preinitiation complex, and stimulation of transcriptional elongation) © McGraw Hill 45 Changes that occur with the formation of a pre-initiation complex 3. Chromatin remodeling and histone modification: An activator protein recruits a chromatin remodeling complex (such as SWI/SNF) and a histone-modification enzyme (such as histone acetyltransferase). Nucleosome may be moved, and histone may be evicted or replaced with variants. Some histones are subjected to covalent modification, such as acetylation. 4. Formation of the preinitiation complex: General transcription factors and RNA polymerase II are able to bind to the core promoter and form a preinitiation complex. © McGraw Hill 46 Simplified model for the formation of preinitiation complex at a eukaryotic gene promoter Fig 15.10 © McGraw Hill 47 Elongation Phase of Transcription Overview Elongation, or synthesis of an RNA transcript, occurs after the preinitiation complex has formed Figure 15.11 shows the different events are required for elongation to occur. These include: Formation of an open complex Promoter escape Proximal promoter pausing Histone modifications © McGraw Hill 48 Key Events During Elongation Phase of Transcription 1. Formation of an open complex- the DNA strands must be separated into an open complex so that one of them can act as a template for RNA synthesis. TFIIH has a subunit that functions as a DNA translocase, separating the DNA strands to convert the closed complex to an open complex. 2. Promoter escape - At the core promoter, GTFs and mediator bind to RNA polymerase II and prevent it from traveling along the template strand. For elongation to occur, RNA polymerase II must be released from this binding, an event called promoter escape. Transcriptional activators facilitate the switch between the initiation and elongation stages. The phosphorylation of CTD allows promoter escape. © McGraw Hill 49 Key Events During Elongation Phase of Transcription 3. Proximal promoter pausing – regulates most eukaryotic genes. RNA polymerase II pauses in RNA synthesis while still close to the transcriptional start site. Involves the binding of two factors, DRB sensitivity- inducing factor (DSIF) and negative elongation factor (NELF). To release the pause, positive transcriptional elongation factor b (P-TEFb) phosphorylates both DSIF and NELF, which results in the release of NELF and causes DSIF to facilitate elongation. RNA polymerase II is now able to transcribe the rest of the gene © McGraw Hill 50 Key Events During Elongation Phase of Transcription 4. Histone modifications - Histone-modifying enzymes play a key role in histone removal and replacement during the elongation phase of transcription, through histone acetylation, H3 methylation, and H2B ubiquitination. © McGraw Hill 51 Fig 15.11 © McGraw Hill 52 Steroid Hormones and Regulatory Transcription Factors As a specific example of a regulatory transcription factor, let’s consider those that recognize steroid hormones Regulatory transcription factors that respond to steroid hormones are termed steroid receptors The hormone binds to the transcription factor The ultimate action of a steroid hormone is to affect gene transcription Steroid hormones are produced by endocrine glands Secreted into the bloodstream Then taken up by cells that respond to the hormone © McGraw Hill 53 Steroid Hormones and Regulatory Transcription Factors Cells respond to steroid hormones in different ways Glucocorticoids These influence nutrient metabolism in most cells They promote glucose utilization, fat mobilization and protein breakdown Gonadocorticoids These include estrogen and testosterone They influence the growth and function of the gonads © McGraw Hill 54 Action of Glucocorticoid Hormones GRE (Glucocorticoid Response Elements) are found within enhancers Located near dozens of different genes, so the hormone can activate many genes Consists of two sequences that are close together 5’-AGRACA-3’ 3’-TCYTGT-5’ Glucocorticoid hormones enter the cell and bind to glucocorticoid receptor subunits that dimerize, enter the nucleus, bind to a GRE, and activate gene transcription © McGraw Hill 55 Fig 15.12 © McGraw Hill 56 The CREB Protein As a second example, let’s consider a regulatory transcription factor, the CREB protein, which responds to cAMP (cyclic adenosine monophosphate), a common signaling molecule CREB is an abbreviation for cAMP response element-binding protein CREB protein becomes activated in response to extracellular cell- signaling molecules that cause an increase in the cytoplasmic concentration of cAMP CREB protein binds to two adjacent sites with the following consensus sequence 5’-TGACGTCA-3’ 3’-ACTGCAGT-5’ Known as a CRE (cAMP response element) © McGraw Hill 57 Activity of the CREB Protein In response to extracellular signaling molecules, like epinephrine, cAMP is made and acts as a second messenger cAMP activates protein kinase A, which phosphorylates CREB protein and thereby allows it to bind to a coactivator, CREB-binding protein (CBP) The binding of CBP causes transcription of the adjacent gene to be greatly increased Note: Unphosphorylated CREB can bind to DNA, but cannot activate RNA polymerase © McGraw Hill 58 Fig 15.13 © McGraw Hill 59 Mechanisms that Result in Gene Repression Gene repression - any mechanism that inhibits the transcription of a gene, resulting in a lower level of RNA synthesis from that gene Repressor - a protein that binds directly to a DNA sequence, such as a regulatory element within an enhancer, and inhibits transcription. Repressors exert their effects by interacting with other proteins or protein complexes called corepressors Gene repression can be short-term, by directly inhibiting steps needed for gene activation, or long-term, as in gene silencing via the formation of heterochromatin Heterochromatin formation is discussed in Chapter 16. © McGraw Hill 60 Chromatin Immunoprecipitation Sequencing Also known as ChIP-Seq A technique used to map the locations of specific nucleosomes within a genome Allows determination of: Where nucleosomes are located Where histone variants are found Where covalent modifications of histones occur Chromatin immunoprecipitation is combined with DNA sequencing Performed in species where the entire genome has been sequenced © McGraw Hill 61 Chromatin immunoprecipitation sequencing (ChiP-Seq) Fig 15.14 © McGraw Hill 62 A Comparison of Transcriptional Regulation in Bacteria, Archaea, and Eukaryotes Bacterial Archaeal Eukaryotic Gene organization Often in operons Often in operons Usually in single genes; though operons are observed in some species such as Caenorhabditis elegans (nemotode worm) Location of regulatory Operators are typically located close to Operators are typically located Enhancers are typically located at elements the promoter close to the promoter. more distant sites from the core promoter, and a loop must form in the DNA to bring the enhancer and core promoter close together. GTFs Sigma (σ) factor is needed for Archaea have homologs to Six general transcription factors and promoter recognition. eukaryotic TBP (TATA-binding mediator assemble at the core protein) and to subunits within TFIIB promoter. and TFIIE. Mediator Absent Absent Present Activators and Repressors Bind to an operator site. Some of them Bind to an operator site. Some of Bind to an enhancer and recruit interact directly with RNA polymerase, them interact directly with RNA coactivator complexes. The while others make local changes in polymerase, while others make coactivators may alter chromatin DNA structure, like promoting the local changes in DNA structure, like structure and interact with GTFs formation of a loop or a bend. promoting the formation of a loop or and mediator to influence promoter Repressors may sterically inhibit RNA a bend. Repressors may sterically escape and promoter pausing. polymerase. inhibit RNA polymerase. DNA methylation Yes Yes Yes Riboswitches Yes Yes Yes (but not in animals) Tab 15.5 © McGraw Hill 63